Research conducted by Answers in Genesis staff scientists or sponsored by Answers in Genesis is funded solely by supporters’ donations.
Abstract
I present a review of the two types of planets and the orbital characteristics of their satellites and evaluate evolutionary explanations for them. While the naturalistic theories may explain certain features, other features require a number of well-timed catastrophic events. To have so many of these events is very improbable. The evolutionary theory cannot explain certain aspects of solar system bodies. To date, there have been few comprehensive proposals for a creationary model of the solar system. The invocation of design must be carefully thought through.
Keywords: Jovian planets, terrestrial planets, regular satellites, irregular satellites
Introduction
The solar system sports a bewildering array of planets and satellites. In recent decades, the contributions of both large ground-based telescopes and space probes, such as the Voyager, Galileo, and Cassini missions, have greatly increased the number of known planetary satellites. We see patterns among the planets and satellites, but we also see many exceptions to the patterns. Recent discoveries have helped establish many of these anomalies, so the time has come to discuss some of these in a recent creation framework. First, I ought to define a few terms.
Under the influence of the gravity of other objects, bodies follow curved paths through space. If their relative speeds are large, objects will pass one another once on parabolic or hyperbolic trajectories. If the speed is low enough, objects will follow closed paths that we call orbits. All orbital paths are elliptical in shape, a fact discovered empirically for the planets by Johannes Kepler (Kepler’s First Law of planetary motion) and that Sir Isaac Newton later derived in the general case from his laws of motion and gravity. With all orbits, the central body (the body about which an object orbits) is at one focus of the ellipse. The point on the ellipse closest to the central body has a special name. For objects orbiting the sun, this point is the perihelion. The point on the other side of the orbit that is farthest from the central body also has a name. For objects orbiting the sun, this point is the aphelion. For objects orbiting the earth, those points are perigee and apogee, respectively. Similar points on orbits about other objects have similar names. For instance, for objects orbiting Jupiter, those points are perijove and apojove.
Ellipses come with many degrees of flatness, a characteristic defined by the eccentricity. Eccentricity is the distance between the two foci divided by the major axis. A circle is a special kind of an ellipse, an ellipse of zero eccentricity. Even for artificial satellites, it is very difficult to get an orbit that is exactly circular. More flattened ellipses have larger eccentricities. The limiting upper value for the eccentricity of an ellipse is one. An eccentricity of exactly one corresponds to a parabola, so an ellipse cannot have eccentricity equal to one, but it may be very close to one. Many comet orbits appear to have eccentricity of one, but their eccentricities probably are less than one by a very small, albeit indistinguishable, amount.
An ellipse defines a plane. That plane may have any orientation, as long as the central body at one focus is in the plane (Kepler’s First Law of planetary motion). We define the orientation of an orbit by the angle between the orbital plane and some reference plane. We call this angle the inclination. In the case of bodies orbiting the sun, the inclination is measured with respect to the ecliptic, the orbital plane of the earth (see fig. 1). In the case of objects orbiting planets, we usually measure the inclination with respect to the equator of the planet.
The size of an orbit is defined by the major axis (or more often, the semi-major axis). The length of time to complete one orbit is the period. Kepler’s Third Law of planetary motion states that the square of a planet’s orbital period is proportional to the cube of the orbital size. Kepler discovered his three laws of planetary motion empirically, but Newton showed that this relation is true for all orbits.
When viewed from above the earth’s North Pole, the planets revolve around the sun in the counter clockwise (CCW) direction. We call this direction prograde. Since the central body of the solar system, the sun, also rotates on its axis CCW, it is best to define the prograde direction in terms of the sun’s rotation. Most of the planets also rotate on their axes in the prograde direction, though there are two exceptions, Venus and Uranus. We call this direction retrograde. In discussing the orbits of planetary satellites, it is customary to define the prograde direction in terms of the rotation of the planet about which a satellite orbits. The earth’s moon and most of the larger satellites of the other planets orbit in the prograde direction. However, some satellites, particularly smaller ones, orbit retrograde.
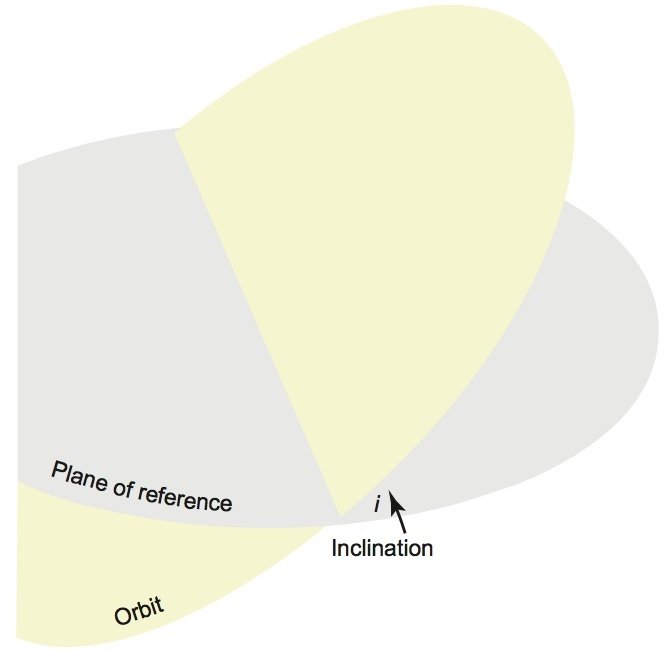
Fig.1. The inclination of an orbit, i, is the angle that the orbital plane makes with respect to some reference plane.
A planet is a large body that orbits the sun. With the reclassification of Pluto as a planet in 2006, astronomers have attempted to define how large a body must be to be considered a planet (Faulkner 2009). Astronomers will continue to refine this definition in years to come. The current definition has some evolutionary trappings. For our purposes here, I will arbitrarily define the minimum planetary size to be the mass of Mercury, the smallest planet. There are many other objects much smaller than planets that orbit the sun. These objects fall into two broad groups: minor planets and comets. Minor planets (commonly called asteroids) orbit the sun with prograde, low eccentricity (typically less than 0.25), low inclination (typically less than 30°) orbits. Since planets also have prograde, low eccentricity (less than 0.2), low inclination (less than 7°) orbits, astronomers prefer the term minor planet over asteroid, because that term reinforces the orbital similarity of asteroids to planets. As of January 2014, there are more than 600,000 known minor planets,1 with thousands of new ones discovered each year. Comets have more eccentric orbits, with eccentricities typically between 0.8 and 1.0, and their orbits generally have higher inclination (all the way to 90°) than minor planets do. Nearly half of comets have retrograde orbits, which could be expressed as inclinations between 90° and 180°. The total number of known comets is less than 4,000.
Comets and minor planets have physical differences as well. Most minor planets (at least the ones that orbit the sun closer than Jupiter) have higher density, suggesting more rocky composition. On the other hand, comets have lower density, which is consistent with non-rocky composition with great porosity. Comets are made of icy material with interspersed very small solid particles that we call dust. These compositional differences are verified by spectroscopic studies of minor planets and comets. This difference between minor planets and comets mirror the difference between terrestrial and Jovian planets. The four terrestrial planets are closest to the sun and have rocky composition. The four Jovian planets are farther from the sun and have gaseous composition. Actually, much of the interiors of the Jovian planets are undoubtedly liquid, but they consist of materials that generally are gases on the earth, hence the reason we call the Jovian planets gaseous. And the Jovian planets probably have small rocky cores, but their cores account for relatively little of those planets volumes or masses. Because of comets’ very low temperatures, those same materials on comets are in the form of ice. Comets spend most of their time far from the sun, so they, like the Jovian planets, are denizens of the far reaches of the solar system. Therefore, within the evolutionary paradigm, it may not be surprising that comets and the Jovian planets share a common composition.
A satellite is a body, whether large or small, that orbits a planet. Note that there is no size limit on a satellite—a satellite may be very small or very large. Indeed, two satellites, Jupiter’s Ganymede and Saturn’s Titan, are larger than Mercury, the smallest planet. Galileo was the first to discover satellites around another planet—the four largest satellites of Jupiter. We call these satellites the Galilean satellites in honor of his discovery. Galileo called these satellites “moons” in comparison to the earth’s satellite. We commonly keep this tradition by referring to satellites of other planets as “moons.” However, in astronomical parlance, the moon refers to the earth’s satellite, and the preferred term for a body orbiting other planets is “satellite,” and I will endeavor to use that term here.
Retrograde Rotation
Astronomers have an evolutionary theory of the formation of the solar system. Some creationists have reviewed different aspects of this theory (Oard 2002; Spencer 2007; Henry 2010). The forerunner of this theory was Laplace’s nebular hypothesis of two centuries ago. According to this theory, the solar system formed from the collapse of a large cloud of gas and dust. Much of matter fell to the center to form the sun, and the remaining material flattened into a disk from which the other members of the solar system formed. The orbital and rotational motions of the various solar system bodies would have to be the result of the rotational motion of the initial cloud. This primordial rotation would have been very slight, but through the conservation of angular momentum, rotational motion would have increased as the matter in the cloud shrank in size. Since the primordial motion must have been in one direction, one might expect that all rotational and orbital motion today ought to share this (prograde) direction. Hence, any retrograde motion requires some explanation.
Evolutionists have offered some explanations for these anomalous motions. My purpose here is to describe these explanations and evaluate them at some level. Some creationists see design in these anomalies. They argue that the evolutionary origin of the solar system can produce only prograde motions, and thus any retrograde motions defy naturalistic explanation and hence must indicate design. However, there is a consistency problem with this approach. Usually, creationists invoke design to explain what appear to be very finely-tuned systems such as the ones we see in living things. Any random changes in such smoothly running, ordered systems would result in failure of the systems. Furthermore, the question is raised how random events that supposedly drive evolution could produce such ordered systems in the first place. That is, chaos, not order, ought to result from random processes. The answer, of course, is that only design could produce such order. Design implies a Designer. However, in the case of the solar system, many creationists seem to claim that random processes can produce only prograde motions, and furthermore that anomalies, or chaotic features, can result only from design. This approach seems to be the reverse of what creationists normally claim. We shall see that some random events can and even must produce what appear to be chaotic characteristics. This is what we ought to expect from random events. The question is, how probable are those random events required to explain anomalies?
Astronomers explain retrograde motions in the solar system with two mechanisms. In the case of retrograde rotation of planets, late hits in the formation of those planets are invoked. In the case of retrograde orbiting satellites, these satellites are claimed to have been captured minor planets. Retrograde satellites tend to be small, which is consistent with them being captured minor planets. Many creationists perceive these explanations as ad hoc hand waving and amount to no more than just-so stories. However, we are talking here of historic, not operational science. The generally accepted standard in historical science is whether such a process is possible and reasonable. Certainly, these sorts of propositions cannot be tested in the traditional approach of operational science.
Two Types of Planets
Based upon their gross properties, we recognize two types of planets. The terrestrial planets are the first four planets from the sun, while the last four planets are the Jovian planets. Table 1 is a brief summary of how the two types of planets differ in seven broad categories. A comprehensive theory of the solar system ought to be able to explain the differences in the two types of planets. Returning to the evolutionary theory of solar system formation, astronomers generally think that the material in the flattened disk coalesced into many small bodies called planetesimals. However, the reason why the particles began to stick together to form planetesimals has not been explained. A detailed discussion of the latest theories of this process would be most welcome in the creation literature. Forming in the now flattened disk, the planetesimals had low inclination, prograde orbits. Planetesimals in similar orbits would have low relative velocity, and such planetesimals could bump into one another and stick together to form larger planetesimals. How the planetesimals stuck together is not known either. Eventually, some of the planetesimals became large enough to have small, but appreciable, gravity. This gravity could attract other planetesimals so that some of the planetesimals began to grow to appreciable size. With increasing size, increasing gravity allowed the larger planetesimals to grow ever larger. Eventually, a few of the larger planetesimals became large enough that their gravity dominated within their respective regions of distance from the sun. These objects would effectively clean their regions of most other planetesimals and eventually become the planets.
Table 1. A comparison of terrestrial and Jovian planets.
Characteristic | Terrestrial | Jovian |
---|---|---|
Distance from sun | Near | Far |
Density | High | Low |
Mass | Low | High |
Size | Small | Large |
Rotation period | Slow | Fast |
Number of satellites | Few | Many |
Rings | No | Yes |
In this hypothesis, the forming sun’s nascent radiation would have heated the planetesimals in the inner solar system. This heating would evaporate and drive away the abundant lighter elements, such as hydrogen and helium, but also much of the nitrogen and oxygen as well. At greater distance from the sun, the radiation was not intense enough to evaporate and drive away these lighter, volatile elements. Thus, the planetesimals, and hence the forming planets, in the inner solar system would be deficient in the cosmically abundant hydrogen and helium. However, more distant forming planets would retain most of their primordial hydrogen and helium and thus would be abundant in the lighter elements. In the solar system, the frost line that separates these two distinct regions is estimated to be about five astronomical units (AU) from the sun; Jupiter is 5.2 AU from the sun. This is the evolutionary explanation of why there are the two kinds of planets. The planetesimals closer to the sun, from which the terrestrial planets formed, lacked the lighter elements. This is why the terrestrial planets have such high density. However, the Jovian planets formed from planetesimals far from the sun, and hence retained the lighter materials, which explains why the Jovian planets have such low density. Hence, the first two characteristics of Table 1 are related. The next two characteristics are related too, because originally the planetesimals were dominated by lighter materials, so the loss of lighter material left very little matter for the terrestrial planets (as compared to the Jovian planets), and so the terrestrial planets are much smaller and less massive than the Jovian planets. These features are consistent with the evolutionary explanation.
What of the last three differences between the terrestrial and Jovian planets, rotation period, the number of satellites and the existence of rings? Presumably, many of the satellites of the solar system formed in place in orbit around their parent planets. These satellites thus are greatly grown planetesimals that, while under the gravitational influence of the developed planets, managed to escape amalgamation. This is especially true of the larger satellites of the Jovian planets, but less so of their smaller satellites, because many of them could be captured minor planets. Notice that one of the properties of the Jovian planets is that they have many satellites, while terrestrial planets tend to have few, if any satellites. Indeed, there are three satellites among the terrestrial planets, but only the earth’s moon has any appreciable mass (Mars’ two satellites are exceedingly small). As I shall soon show, the moon has orbital characteristics that suggest its origin was unique. So, one could say that having no significant satellites is a characteristic of terrestrial planets. Evolutionists assume that the abundant mass in the realm of the Jovian planets due to no large loss of hydrogen and helium is the reason why that there are so many satellites of the outer planets—there was just far more mass for a large number of satellites to form.
Ring systems typically are explained in terms of a satellite that ventured too close to a planet and was disrupted by the planet’s tidal force. Indeed, it is now known that rings are very short-lived phenomenon, and thus creationists have used the existence of rings as evidence of their recent origin (Slusher 1980; Snelling 1997; Henry 2006). However, it does not follow that the solar system is young because planetary ring systems are young. Evolutionists agree that rings are young, but they hypothesize that each of the rings recently formed by the disruption of a satellite. After all, Jovian planets have many satellites that could be so sacrificed. However, one could ask how probable it is that we live at a time when all four Jovian planets have rings, when rings are such short-lived phenomena. And this raises the question of why Jovian planets have so many satellites in the first place. The evolutionary theory may not satisfactorily explain that, so ultimately it cannot explain why the Jovian planets have rings either. Lastly, the evolutionary theory cannot explain rapid rotation of the Jovian planets.
While most planetesimals ended up in the planets, in the evolutionary scenario not all planetesimals were formed into planets. Many of the leftover planetesimals collided into the forming and newly formed planets early in solar system history. Astronomers call this era of frequent impacts the early and late heavy bombardments. Supposedly, the late heavy bombardment ended about 3.8 billion years ago, and the solar system has experienced a much diminished and continuously decreasing period of impacts since then. According to the theory, the planetesimals that have survived to today are the minor planets and comets that we now see. Those planetesimals closer to the sun lost their lighter elements and thus have compositions similar to the terrestrial planets. These are the minor planets. The planetesimals far from the sun retained the lighter elements and have compositions similar to the Jovian planets. These are the comets. Given that minor planets at least out to the asteroid belt generally have rocky composition, the division between the two compositions was in the vicinity of or just beyond the asteroid belt. Incidentally, this theory posits that the asteroid belt is a collection of planetesimals that failed to amalgamate into a planet, perhaps because the perturbing influence of Jupiter’s gravity that kept those bodies churned. The strong gravity of the Jovian planets probably managed to eliminate most of the planetesimals in their midst.
Beyond the orbit of Neptune there are no planets, so the planetesimals there presumably still exist. Over the past two decades, astronomers have discovered hundreds of objects orbiting there. To emphasize their location beyond the orbit of Neptune, astronomers frequently call these objects Trans Neptunian Objects (TNOs). With its 2006 demotion from planet status, Pluto is just one of the largest TNOs. Most astronomers think that TNOs are in the Kuiper Belt, a hypothetical toroidal distribution of comet nuclei orbiting the sun beyond the orbit of Neptune, and the source of short period comets (Newton 2002; Worraker 2004). Being so far from the sun, their composition likely will be icy, as the composition of most small bodies (Jovian planet satellites) so far from the sun is. While the Kuiper belt is the proposed source of short period comets, most astronomers think that it also is the ultimate source of all comets (Faulkner 1997). With this view, the gravity of the Jovian planets gradually perturbs the objects in the Kuiper belt. Some of these perturbations pull Kuiper belt objects into the inner solar system, producing short period comets, which have low inclination, prograde orbits. Other perturbations boost Kuiper belt objects into much higher orbits. These perturbations supposedly randomized the orbits of the objects so that they have a spherical distribution, with many orbiting the sun retrograde. This would be the hypothetical Oort cloud, from which extra-solar perturbations return some of these objects back into the inner solar system to appear as long period comets, comets with highly eccentric, high inclination orbits, with half the orbits prograde and half retrograde.
This has been a very brief discussion of the evolutionary explanation of the origin of the solar system, which purports to explain not only the sun, but the two types of planets, and the existence of minor planets, comets, and satellites. In this hypothesis, the larger satellites and some of the smaller satellites are primordial. That is, these satellites formed early on in orbit around their respective planets. However, as previously mentioned, there is another possible origin scenario for some satellites. The alternate origin is that some satellites are captured minor planets or even comets. These captures could have happened any time after the initial formation of the solar system, and could even happen today. Are there any signatures of capture events? We would expect that capture usually results in very elliptical and often highly inclined orbits. Many of these orbits may be retrograde (equivalent to an inclination greater than 90°). Thus, if a satellite has a very elliptical, highly inclined, or retrograde orbit, then we might suspect that it may have been captured. Incidentally, we might expect capture events in a recent creation, especially with the inclusion of catastrophes, so we ought not necessarily to fear the possibility of capture for an explanation for some of the satellites.
Before moving on, I ought to mention a few problems with the evolutionary scenario for the solar system’s formation. First, gas clouds do not spontaneously collapse—clouds that we observe appear to be in hydrostatic equilibrium and lack sufficient gravity to initiate collapse. There are several suggested mechanisms to initiate collapse, such as a shock wave or cooling by dust particles, but all of them require that stars first exist (Faulkner 2001). Second, astronomers can only guess at a mechanism to cause the matter in the disk to begin to stick together to form planetesimals, for the particles normally would not coalesce. Third, even if tiny particles begin to stick together, it is a large step to get the small particles to grow into ones massive enough to have sufficient gravity to continue the growth process. Typically, collisions between particles are so energetic as to disrupt the particles. Thus, there is much hand waving to initiate solar system formation in this way. Fourth, while the sun contains more than 99% of the mass of the solar system, it contains only about 1% of the angular momentum of the solar system. Evolutionists have proposed that much of the angular momentum was transferred from the sun to the planets through magnetic fields, or that early solar winds carried angular momentum away, or that magnetic braking has occurred. Fifth, while the evolutionary theory can explain certain differences between the terrestrial and Jovian planets, it cannot explain all the differences. For instance, Jovian planets all rotate very rapidly on their axes, and terrestrial planets generally rotate more slowly. Yet, there is no reason why this should be the case.
While the evolutionary theory can explain the difference in size and composition between the Jovian and terrestrial planets, what is the creationary explanation of why there are two types of planets? Alas, at this time there is no proposal for this. That does not mean that a creationary explanation is not possible. Rather, it means that one has not been developed yet. This question does not appear even to have been raised in the creation literature, let alone answered. Further work on this is most desirable.
Discussion
Let us now discuss specific cases of planets and their satellites. As previously mentioned, two planets rotate retrograde. Astronomers usually explain this by collisions of very large objects into those planets late in their formation in such a way that the collisions imparted reverse spin. Though some people have difficulty understanding how this could happen, it actually could work. If a body rotating prograde is overtaken by a faster moving large body so that the impacting body has a grazing impact on the first body on the side facing the sun, then the collision would impart a retrograde torque. A single collision is unlikely to impart enough retrograde torque to completely reverse the body’s spin, especially if the impacted body is large, but a number of such collisions could do so. This is understandable with Venus’ very slow retrograde spin, but Uranus’ retrograde spin is very fast.
However, a randomly distributed collision of any magnitude likely would alter the orbit of a planet. Unless an orbit is already quite elliptical, such a random collision would tend to make the orbit more elliptical. The orbits of the planets are nearly circular, so a random collision would tend to make the orbit more elliptical. Venus and Uranus have the most circular orbits of the eight planets. If one were to look at just their orbits, Venus and Neptune would be judged the planets least likely to have suffered large, late impacts. But in the evolutionary paradigm, the retrograde rotation of Venus and Uranus imply that they were most likely to have suffered large, late collisions. Thus, the orbits and rotation directions of Venus and Uranus contradict one another with regard to late, large collisions. To salvage this in an evolutionary explanation, one must posit very improbable directions and timings in those impacts.2
There is a great range in the tilt of the axes of the various planets. The earth has a very familiar 23½° tilt. The tilt of Mars is very similar at 25°. Saturn’s tilt is 30°, but Jupiter has a tilt of only 3°. Uranus has a large 98° tilt, but one can view this as 82° with retrograde rotation. Either way, Uranus’ rotation is roughly at right angles to its revolution. Again, astronomers usually explain these variations in axial tilt by appealing to large, off-center impacts. If a large impact occurs near the pole of a forming planet, the collision would impart top or bottom spin to the planet. When combined with initial tilt very close to zero, viscous motions in the forming planet could produce a new, single spin axis inclined to the orbital plane. This answer thus demands that there were many violent collisions in the early history of the solar system. These collisions would tend to destroy the forming planets. Many astronomers now think that the planets may have formed and reformed a number of times in the early stages of their development.
However, there is a problem for the late-hit explanation for planetary rotation axes. Most of the major satellites3 and many of the smaller satellites orbit very closely to the equatorial planes of their respective planets and in the same direction that the planets spin. This is true of the rings of the four Jovian planets as well. With the evolutionary theory, this fact requires that each planet and its forming region established a preferred axis of rotation early on. That is, if a planet and its satellites had formed before the rotation axis of the planet had been reoriented to its current value, then the satellites would orbit in the old equatorial plane. This is not what we see. Consider the 27 known satellites of Uranus. The innermost 18 satellites have very circular orbits that have very low inclination with respect to the Uranian equator. Any reorientation of the rotation axis of Uranus must have happened prior to the formation of those satellites. However, this would have been prior to the formation of the planet itself, when the Uranian system still was a cloud. An impact on a cloud would not realign the entire cloud’s rotation axis. In other words, a late, large collision could explain the re-orientation of the planet if the planet mostly had formed already, but it could not explain the realignment of its many satellites. What we observe requires that the current rotation axis of each planet must have been established before most of the satellites formed. This places some rather severe constraints upon the order and timing of events within the evolutionary theory, and this is a possible topic of further discussion.
To counter this problem, planetary scientists propose that there was a gap in time between the formation of a planet and the coalescence of its satellites. The impact that realigned the planetary rotation axis could not reorient the satellites’ orbits, so the reorienting impact must have happened during this time gap. The material that would become the satellites was in the form of planetesimals orbiting in the original equatorial plane, now inclined to the new equatorial plane. The rapid rotation of the planet produced an equatorial bulge, and the bulge produced a torque on the orbiting planetesimals. The torque itself could not cause the planetesimals to realign to the new equator, but rather their orbits would precess along the new equatorial plane. The precessing orbits would lead to collisions between the planetesimals that canceled motion perpendicular to the new equatorial plane, gradually realigning the planetesimal orbits to the new equator. After this, satellites would form from the planetesimals aligned to the new equator. This mechanism with its good timing would have to have happened with all four Jovian planets.
According to this theory, Uranus originally rotated prograde, but had its orientation changed by impacts. Presumably, Uranus’ satellites originally orbited the same direction as Uranus, but this mechanism alone would have left the Uranian satellites orbiting retrograde, opposite to the rotation of the planet. The solution to this difficulty is to hypothesize at least one additional impact during the time gap. The first impact would impart a large change to the tilt of Uranus, but not enough of a change to reverse the direction of rotation. This first episode was followed by realignment of the orbiting planetesimals to the new equatorial plane. A second impact would further increase the tilt of Uranus, making the planet’s rotation retrograde. Subsequent precession and collisions would realign the orbiting planetesimals with the second new equatorial plane, resulting in orbits in the same direction as Uranus’ rotation. One could generalize the situation so that any number of tilt-changing collisions could have happened, so that a particular planet’s tilt would not necessarily have experienced a single, large impact. However, one must question whether all of these impacts would have occurred in the narrow window of time between the coalescence of a planet and the coalescence of its satellites. If just one tilt-altering impact occurred outside of this window, there would be satellites orbiting in a different common plane. Thus, the naturalistic explanation, while possible, is improbable.
This is a good time to discuss the possibility that some satellites are captured minor planets or comets. As previously noted, the hallmarks of a capture event are highly inclined, very elliptical, and possibly retrograde orbits. Astronomers frequently refer to such orbits as irregular, as opposed to regular orbits, which have low inclination, low eccentricity, and are prograde. There is no universally accepted definition of regular or irregular orbits. For purposes here, I shall use the term regular to refer to low inclination, low eccentricity, prograde orbits, while I shall use the term irregular to refer to high inclination, high eccentricity orbits, regardless of whether the orbit is prograde or retrograde.
Mars has two very small satellites, Phobos and Deimos. With the close proximity to the asteroid belt, astronomers have long thought that these two satellites were captured by Mars’ gravity. The two Martian satellites even have similar spectra to C-type (carbonaceous) minor planets. However, recent thermal spectroscopy of Phobos suggests that its surface has a large component of phyllosilicate (Giuranna et al. 2010), a substance common on the Martian surface but not common in C-type minor planets. Even more importantly, the orbits of Phobos and Deimos are regular. These factors argue against capture as the origin scenario for them.
At the time of this writing, Jupiter has 67 known satellites. The innermost eight satellites have regular orbits. Four of these eight are the Galilean satellites, the only large satellites of Jupiter (two are roughly the size of the moon, and two are slightly larger). There is a large gap between the first eight satellites and the ninth satellite. Satellites 9–15 have prograde orbits relative to Jupiter with various inclinations and eccentricities, but all are consistent with a capture origin. The final 52 satellites all orbit retrograde with a range in inclinations and eccentricities. Again, these statistics are consistent with capture origin. It is strange that the capture candidate satellites are segregated with regard to orbit direction and that there are so many retrograde orbits among them. Keep in mind that all of the non-Galilean satellites are very small.
Saturn has 62 confirmed satellites, but only one of them is large (Titan is larger than Mercury). The next six larger satellites have diameters ranging from 12% to 44% that of the moon. The remaining 55 satellites are very small. The first 21 satellites from Saturn include the seven larger ones, and all have regular orbits. Many of these innermost satellites orbit near or within the rings. The 21st and last regular satellite is Titan. The 22nd satellite, Hyperion, has low inclination, but it has a moderately high eccentricity of a little more than 0.1. It also is in a 4:3 resonance with Titan, suggesting a capture or other catastrophic event in its past. The 23rd, satellite, Iapetus, is nearly three times the distance from Saturn as Titan is. Its orbit has low eccentricity, but its inclination is about 15°, suggesting the possibility of capture. After Iapetus there is a large gap, with the remaining 39 satellites beyond the gap having high inclination, high eccentricity orbits. Nine of those satellites, 24, 25, 27, 29, 31, 32, 34, 35, and 40, orbit prograde. Among the irregular Saturnian satellites, the ratio of retrograde to prograde is about 4:1, less than the approximately 7:1 for the irregular satellites of Jupiter. Though the final 21 satellites orbit retrograde, there is not quite the segregation of prograde and retrograde satellites as with the Jovian system.
Uranus has 27 known satellites. Its largest, Titania, has nearly half the diameter of the moon, but it has only about 5% of the moon’s mass. Uranus has four other major satellites. Three of them are slightly smaller than Titania, and the fourth, Miranda, is slightly less than half the size of those three. The Uranian satellites divide nicely into three groups. The inner 13 have very regular orbits among the rings of Uranus. The five major satellites come next, all with regular orbits. There is a large gap between the five major satellites and the remaining nine outer satellites. The nine outer satellites all have irregular orbits, with only one orbiting prograde. This ratio of 8:1 in favor of the retrograde irregular orbits may not be significant, because of the relatively small sample size of prograde satellites. For instance, the discovery of only one additional prograde irregular satellite would reduce the ratio to 5:1.
Neptune has 14 known satellites. The innermost seven are small, have regular orbits, and some are interspersed in the Neptunian ring system. Next is Triton, Neptune’s only large satellite. Triton has 78% the diameter of the moon, but it has only 29% of the moon’s mass. It is the only large satellite in the solar system that has a retrograde orbit. Its orbit is highly inclined, but it has very low eccentricity. Given that two of the three orbital characteristics are irregular, Triton ought to be classed as an irregular satellite. One is tempted to explain Triton’s retrograde, highly inclined orbit by capture or some other catastrophic event, but that ought to result in a decidedly non-circular orbit. Circularizing the orbit would require a series of unlikely events. These orbital characteristics do not match, and so the origin and history of Neptune remains an enigma. There is a very large gap between Triton and the remaining six outer satellites. The orbits of the outer satellites are irregular, with half being retrograde and half being prograde. Thus, Neptune is the only Jovian planet with parity in the orbital direction of its irregular satellites, but the sample size is admittedly low, and this picture could change as more satellites are likely to be discovered.
In summation, the innermost small satellites of the Jovian planets have regular orbits and often are within the ring systems. With only one exception, the major satellites have regular orbits too. The one exception, Triton, has a circular, high inclination, retrograde orbit. The outer small satellites frequently are separated from the inner and large satellites by a great gap. The outer satellites have irregular orbits, with a preponderance of retrograde orbits.
How does one interpret these satellites within the evolutionary paradigm? The regular orbit satellites probably are primordial, forming with their respective planets. The satellites with irregular orbits probably resulted from later capture events. These captures usually would have involved the gravitational interaction of at least three bodies. There are similarities between the orbits of many of the irregular satellites. For instance, five of the irregular prograde satellites of Jupiter have similar orbits. This group is called the Himalia group, after one of the larger members of the group. Similarly, most of the other irregular satellites of Jupiter fall into one of three categories, the Carme, Ananke, and Pasiphaë groups, named for the prototype satellite of each group. Presumably, the members of these four groups did not result from individual captures, but rather they came from four captured minor planets that in turn fractured into the many satellites, most likely as the result of a collision with another body. Similarly, many of Saturn’s irregular satellites fall into three groups, the Inuit, Gallic, and Norse groups. The names of the groups come from the mythologies that the names of the members of each group came from. The irregular satellites of Uranus and Neptune do not have such clear delineation into groups as Jupiter and Saturn, but this may be hindered by the smaller sample size. There probably are yet undiscovered satellites of Uranus and Neptune, so if and when more of their satellites are found this picture may change. The strange orbit of Triton is difficult to explain with the evolutionary paradigm—a circular orbit is difficult to achieve from a capture scenario. There remains the problem of explaining why the orbital planes of the regular satellites are so close to the equatorial plane of their respective planets.
How might we interpret planetary satellites in a recent creation framework? We would agree with evolutionary astronomers that the regular satellites are primordial, albeit would strongly disagree on the time involved. Instead of the planets and their regular satellites forming over millions of years, we believe that they were made in less than one day. Natural processes as they operate today could not do this, so we believe that God accomplished this in a manner apart from how the universe now works, or at the very least, greatly sped up. As for why the regular satellites have orbits within the equatorial planes of their respective planets, we might suggest that God did this to demonstrate the futility of explaining these satellites naturally. This same sort of reasoning would explain the peculiar orbit of Triton. We would also agree that the irregular satellites probably resulted from capture events, often followed by fragmentation to produce the various groups. This suggestion would particularly appeal to those who propose catastrophes across the solar system at the time of the Flood or other epochs.
The orbit of the earth’s moon is most interesting. It is the only major satellite that orbits near the ecliptic. That fact strongly suggests that the origin of the moon is different from any other satellite. Of course, this is no surprise to creationists, because of the moon’s unique purposes (Genesis 1:14–18). Furthermore, God did not make the moon on Day Four along with its planet as He did the other satellites in the solar system, because He made the earth on Day One. How do evolutionists explain the moon’s existence? Originally there were three major theories of the moon’s origin: co-creation, fission, and capture. There are strong objections to all three theories, and these are discussed elsewhere in the creation literature (DeYoung and Whitcomb 2010). Because of these problems, astronomers began developing hybrid models in the 1970s to explain the moon’s origin. These models posit that while the earth was still forming, it suffered a grazing-incidence collision of a Mars-sized object. A portion of that body sank into the earth, but the remainder, as well as some of the earth’s surface and mantle, was thrust into orbit around the earth, from which the moon formed (for a brief critique of the impact hypothesis, see Oard 2000). This is not the same origin scenario for other satellites, which makes the moon unique. Creationists see the moon’s unique orbital status as design, but evolutionists view it as happenstance.
The moon does share one property with many other satellites—it has synchronous rotation. Synchronous rotation is the condition where a body rotates and revolves at the same rate. As seen from a planet’s surface, a synchronous satellite keeps one face toward the planet, and so the planet does not appear to rotate (but it does). Why do satellites do this? It is easy to explain with tides, albeit in a time scale longer than the recent creation model. Satellites raise tides on their parent planets as the moon does on the earth. At the same time, the planets produce tides on their satellites. The tidal bulges raised upon the satellites provide “handles” upon which the planets can exert torques upon their satellites. These torques slow or speed rotation until the satellites are locked into synchronous rotation. The satellites do a similar thing to their planets, but given the huge mass differences between the satellites and planets, the corresponding spin change in the planets is far smaller. Readers may be familiar with the tidal interaction that slows the earth’s rotation. This produces an upper limit of 1.3 billion years for the age of the earth-moon system (DeYoung 1990).
The tidal interaction that brings satellites into synchronous rotation acts very slowly, probably taking longer than the thousands of years in the creation model. It would be desirable to see a good review of this mechanism and the required time scales in the creation literature. The fact that so many of the satellites exhibit synchronous rotation could be used as evidence that the solar system is far older than 6000 years. Recent creationists could easily respond that most satellites were created with or near synchronous rotation. While possible, this seems ad hoc. It would be most helpful if we could identify a purpose for the creation of satellites so. This is a possible topic for further study, but this probably would not amount to a scientifically testable thesis.
In recent years we have discovered a few satellites that do not rotate synchronously. One example is Phoebe, a medium size satellite of Saturn. Evolutionists easily can claim that there has not been sufficient time for Phoebe to have been locked into synchronous rotation. First, it orbits very far from Saturn, taking more than 550 days to orbit once. The tidal interaction is a very steep function of distance, so it would take a very long time for Phoebe to become tidally locked. Furthermore, Phoebe has a moderately eccentric, retrograde orbit. As previously discussed, these are trademarks of capture event. It is possible that Phoebe has not been orbiting Saturn very long, even in a 6000 year old solar system.
Conclusion
I have briefly surveyed some of the gross properties and orbital characteristics of the planets and satellites in light of possible evolutionary and creationary explanations. There are two reasons for thinking that the moon has a unique origin. First, it is the only terrestrial planet satellite of any size. Mars, the only other terrestrial planet with satellites, has two very small ones. If it is a general characteristic of terrestrial planets that they do not have satellites, then the moon ought not to be there. Second, the moon’s orbit lying close to the ecliptic rather than in the earth’s equatorial plane suggests that its origin is different from that of the satellites of the other planets. With the God-given purposes that the moon has (Genesis 1: 14–18), this is not unexpected in the creation model. However, in the evolutionary model, one must assume that the moon just happened to form with these unusual properties from a unique, random event.
To explain the retrograde rotation but nearly circular orbits of Venus and Uranus naturally, one must rely upon a series of improbable events within the evolutionary paradigm. While the creationary model does not necessarily predict such interesting characteristics for these two planets, they are not unexpected either. It may be that the Creator left such indications to dumbfound those who wish to explain the world by entirely naturalistic means. Still it would be helpful if we could develop some better explanation for these two planets other than simply, “God did it.” In discussing purpose of created things, we likely will need to develop a new standard of evidence. This is an issue that I have wrestled with for some time, and I do not yet have a fixed idea for this.
The evolutionary theory can qualitatively explain some of the differences between the two types of planets. It does this by relating the density, mass, and size of the planets to the differences in composition of the planetesimals that formed them. In a similar manner, the evolutionary theory also qualitatively explains why the smaller bodies in the solar system (satellites, minor planets, and comets) tend to transition from rocky to icy composition between Mars and Jupiter. However, the evolutionary theory has no explanation for the differences in rotation rates of the two types of planets, nor the number of satellites, nor the presence of rings systems. Moreover, the evolutionary theory has great difficulty in explaining why the regular satellites orbit in the current equatorial planes of their respective planets. Most textbooks emphasize the former success while ignoring the latter failures. Unfortunately, the creation model as of yet does not offer explanations for the differences in objects in the solar system as a function of distance from the sun. The absence of a coherent creation model may be due to a lack of study on our part, but it may also stem from limitations inherent in applying science to creation events. Perhaps the issues that I have raised here will stimulate further work. We ought not to fear invoking some processes that may have worked in the past, though I would distinguish between Creation Week and post-Creation Week processes. Creation Week processes may have been greatly sped up and may have involved elements of the miraculous, while processes after that week likely followed natural laws as we now know them. Creation Week events would be replete with design implications, implications not amenable to the scientific method. Hence many of our explanations would be more theological or philosophical. It appears that many evolutionists’ explanations are better described this way too, though it appears that few have noticed this.
A broad model of a recent creation of the solar system ought to attempt to account for certain features in the solar system:
- Why there are two types of planets.
- Explain the axial tilts of the planets.
- Explain why most satellites in the solar system have synchronous rotation.
- Explain the groupings of small regular satellites, major satellites, and small irregular satellites of the Jovian planets.
- Account for the odd orbit of Triton.
Invoking design to explain some features, such as planetary tilts, may appeal to some creationists, but such suggestions are ad hoc, unless accompanied by some reasons as to what purpose the supposed design fills. That is, some may see design where none exists. We must be very careful in claiming design for features that under other circumstances might be interpreted as chaotic.
References
DeYoung, D. B. 1990. The earth-moon system. In Proceedings of the Second International Conference of Creationism, ed. R. E. Walsh, and C. L. Brooks, vol. 2, pp. 79–84. Pittsburgh, Pennsylvania: Creation Science Fellowship.
DeYoung, D., and J. Whitcomb. 2010. Our created moon, pp. 30–33. Green Forest, Arkansas: Master Books.
Faulkner, D. R. 1997. Comets and the age of the solar system. Creation Ex Nihilo Technical Journal 11, no. 3:264–273.
Faulkner, D. R. 2001. Does the collapse of a gas cloud to form a star violate the Second Law of Thermodynamics? Creation Research Society Quarterly 38, no. 1:40–44.
Faulkner, D. R. 2009. Planet Pluto 1930–2006. Creation Matters 14, no. 1:1–3.
Giuranna, M., T. L. Roush, T. Duxbury, R. C. Hogan, C. Carli, A. Geminale, and V. Formisano. 2010. Compositional interpretation of PFS/MEx and TES/MGS thermal infrared spectra of phobos. Planetary and Space Science 59, no. 13:1308–3125.
Henry, J. 2006. The age and fate of Saturn’s Rings. Journal of Creation 20, no. 1:123–127.
Henry, J. 2010. Solar system formation by accretion has no observational basis. Journal of Creation 24, no. 2:87–94.
Newton, R. 2002. The short-period comets ‘problem’ (for evolutionists): Have recent ‘Kuiper Belt’ discoveries solved the evolutionary/long-age dilemma? TJ 16, no. 2:15–17.
Oard, M. J. 2000. Problems for ‘giant impact’ origin of moon. Creation Ex Nihilo Technical Journal 14, no. 1:6–7.
Oard, M. J. 2002. The naturalistic formation of planets exceedingly difficult. TJ 16, no. 2:20–21.
Slusher, H. S. 1980. Changes in Saturn’s ring. In: Age of the cosmos, ICR Technical Monograph no. 9:65–72. El Cajon, California: Institute for Creation Research.
Snelling, A. 1997. Saturn’s rings: Short-lived and young. Creation Ex Nihilo Technical Journal 11, no. 1:1.
Spencer, W. 2007. Migrating planets and migrating theories. Journal of Creation 21, no. 3:12–14.
Worraker, B. 2004. Missing: A source of short-period comets. TJ 18, no. 2:121–127.