The views expressed in this paper are those of the writer(s) and are not necessarily those of the ARJ Editor or Answers in Genesis.
Abstract
Schistosomatidae is a family of parasites that infect the blood vessels of mammals and birds. The parasites have a complex life cycle that requires at least two hosts: an aquatic snail (usually freshwater), and either a bird or a mammal. Blood flukes cause devastating pathologies in hosts, and are difficult to reconcile with a creation that God declares to be “good” (Genesis 1). This paper aims to determine the number of created kinds represented in this family of parasites, the original hosts for these animals, and the diversification pattern and events that produced the current species. We employed a statistical baraminic study to detect evidence of continuity within family Schistosomatidae and any discontinuity between members of the family and other blood parasites. We also compared the pathologies, geographic ranges, and host specificities of bird schistosomes and mammal schistosomes to provide information on the original hosts and diversification pattern. Members of family Schistosomatidae appear to represent a single created kind that is distinct from the members of other families of blood parasites. Bird schistosomatids are generally less pathogenic than their mammal counterparts, and have wider geographic ranges and less host specificity. Schistosomatidae may represent a single created kind that was created to live within one or several kinds of birds, providing some benefit to its host. As members of this kind moved into different hosts or diversified along with its host, they ceased providing any benefit to their symbiotic partner. The resulting species are some of the most important and dangerous organisms on earth.
Keywords: parasites, hosts, mammals, birds, Genesis kinds, animal diversification
Introduction
Parasites are organisms that live inside of or on another organism, benefitting at a fitness cost to the host by competing with the host for resources or directly reducing the host organism’s reproductive output. Parasites were likely created as mutualistic or commensal symbiotic organisms with a specific purpose and for specific tasks (Ingle 2015). Many created kinds likely lost the ability to benefit the host (or entered animals not intended to serve as hosts) and developed parasitic lifestyles. Some organisms that are neutral of beneficial in one host are detrimental in another. Devkota et al. (2014) pointed out that the relationship of parasites and host organisms is so intimate that the symbionts are often subject to co-extinction events, as the hosts serve as vessels for the parasites that live nowhere else on earth.
The most successful parasite individuals, who produce the most offspring, must compete well for resources without killing the host. Even successful parasites reduce the fitness of the host and thus produce selective pressure on the host (Lozano 1998). Active infections reduce host energy reserves (Barber et al. 2008) and thereby influence the behavioral traits of these animals (Kortet, Hedrick, and Vainikka 2010). Robar, Murray, and Burness (2011) found that parasites can increase the resting metabolic rate of their symbiotic partners and cause hosts to increase food intake to compensate. These impacts in host energy reserves can have profound impacts on their reproductive output. Additionally, some parasites directly impede reproduction. The bacterium, Wolbachia, reduces gamete production in many insect species (Francis 2009). The rat tapeworm, Hymenolepis diminuta, causes reduced reproduction in the flour beetle, Tribolium confusum (Shostak 2009, 2012). Parasites can leave evidences of their infection on hosts long after the pathology caused by their presence (Thomas et al. 2000).
Several families of parasites contain members with the ability to infect and harm humans. Schistosomatidae contains some of the most devastating trematode parasites, including five species that infect humans. These parasites are collectively known as blood flukes, and infect the vessels of the definitive host. Many trematode parasites have a three-host life cycle, beginning with an aquatic snail (first intermediate host) and ending with an organism in which the trematode can reach sexual maturity (definitive host). Members of this family are unusual in that they directly penetrate the skin of the final host organism without using a second intermediate host. Most species of schistosomatids are parasites of birds (Schuster, Aldhoun, and O’Donovan 2014), and have wide geographical distributions (Al-Kandari et al. 2012). Individuals within the genus Austrobilharzia infect shorebirds (mainly seagulls), and are globally distributed (Lockyer et al. 2003). Other schistosomatids, such as Bivitellobilharzia nairi, infect mammals and have very narrow distributions (Devkota et al. 2014). The genus, Schistosoma, includes only species that infect mammals and contains five species that induce pathology in humans (Lockyer et al. 2003). There are 14 genera and approximately 100 species of schistosomatids (Khalil 2002).
Most phylogenies of the relationships among members of Schistomatidae are based on molecular characters and focus on Schistosoma. Each cell contains multiple copies of the mitochondrial genome, making the mitochondrial DNA (mtDNA) an abundant and efficient tool for molecular analyses of all organisms. Webster and Littlewood (2012) noted the variability of mtDNA within and between species and advocated for its use in genetic studies of schistosomatids. While molecular characters are important and useful tools in studying the associations among groups, morphological and behavioral features sometimes illustrate relationships simpler than do molecular features. Phylogenies based on morphological information have fewer disagreements than those based on molecular characters. Beltran et al. (2010) found that mating system (whether monogamy, polygamy, or polygynandry) drives morphological trait differences among schistosomatids. Morphological characters of members of this family illustrate a difference in the ancestries of the species that infect man and demonstrate that the infection of man occurred in several different groups within the family (Lockyer et al. 2003). Lockyer et al. (2003) note that morphological studies are important to connect Schistosoma with the other genera of blood flukes. While many researchers have studied the phylogeny of Schistosomatidae, the relationships among the members of the taxon have not been determined using a statistical baraminic study.
Mace, Sims, and Wood (2003) found evidence for the apobaramin (for definition, see Table 1) status of Schistosomatidae, with the members of the family being discontinuous with all other digeanean flukes. Schistosomatids share many unique features that support the apobaraminic status: they are dioecious (sexes are separate); members lack a pharynx and prepharynx; the genital pore is posterior to the ventral sucker; the vitellarium are well developed, follicular, and posterior to the ovary; eggs are non-operculate; they are found in the vascular system of birds and mammals; and they possess a gynaecophoric canal (Lockyer et al. 2003). Mace, Sims, and Wood (2003) demonstrated two monobaramins within genus Schistosoma, noted the similarities between monobaramins (each member is continuous with all other members of the group), and postulated the existence of a single schistosome baramin (holobaramin). Regardless of the number of holobaramins within this family, the original kinds diversified into the species we find infecting birds and mammals today.
Table 1. Definitions of terms often used in baraminic studies.
Term | Definition |
---|---|
Apobaramin | A group that contains members, who are discontinuous with all other groups but may or may not be continuous with all members of the group. |
Monobaramin | A group that contains members, who are continuous with all other members of the group and may or may not be discontinuous with all other groups. |
Holobaramin | A group that contains members, who are discontinuous with all other groups and are continuous with all members of the group. This is a complete set of individuals that represent a single created kind. |
Organisms that complete their life cycle in hosts can only diversify as their hosts diversify. Members of Schistosomatidae have two hosts in the life cycle, and can diversify with changes in either. The original kind or kinds within this parasite family likely differentiated under two conditions: they diversified along with their hosts, and they became more varied as they switched intermediate or definitive hosts. These two patterns of variation are not mutually exclusive and likely both contributed to the relationships among schistosomatid species today.
The purpose of this study is to determine the baraminic status of Schistosomatidae and to explain the dominant method of diversification in the original kind or kinds. We predict that this family represents a holobaramin, and that most variation in the family resulted from the diversification of intermediate hosts after the Creation Week.
Methods
Baraminic status of schistosomatidae
In order to understand the baraminology of the Schistosomatidae, we ran statistical baraminological analyses on two character datasets of schistosomatids and one character dataset of spirorchiids on BDISTMDS version 2.0 (Wood 2008b). This software conducts baraminic distance correlation (BDC)—the statistical significance of the similarity between two organisms determined through baraminic distance, which is measured via linear regression. In BDC, significant positive correlation between organisms implies continuity and probable inclusion into the same monobaramin, whereas significant negative correlation implies discontinuity between the organisms (Robinson and Cavanaugh 1998). Additionally, BDISTMDS plots taxa as points in three-dimensional space through classical multidimensional scaling (MDS) by reducing the many dimensions of baraminic distance to three. This reduction leads to distortion, which is measured by BDISTMDS as stress (Wood 2005b). If taxa—represented as points in MDS—cluster closely together in multidimensional character space, then this implies the taxa are continuous. Large gaps between clusters imply discontinuity.
The first schistosomatid dataset comes from a PhD thesis by Carmichael (1984), and includes 14 schistosomatid taxa (Schistosoma, Bivitellobilharzia, Heterobilharzia, Orientobilharzia, Schistosomatium, Austrobilharzia, New World Macrobilharzia, Old World Macrobilharzia, Ornithobilharzia, Sinobilharzia, Bilharziella, Trichobilharzia, Gigantobilharzia, and Dendritobilharzia), no outgroup taxa, and 40 morphological characters.
We compiled the second dataset by taking 13 reproductive characters from Beltran et al. (2010) and 16 morphological characters from Lockyer et al (2003). These authors included 27 schistosomatid species (Fig. 1) and one outgroup taxon, Griphobilharzia amoena, which is no longer considered a schistosomatid (Beltran et al. 2010). We converted the Beltran et al. (2010) continuous characters to discrete characters so that we could analyze the dataset in BDISTMDS according to Robinson and Cavanaugh (1998).
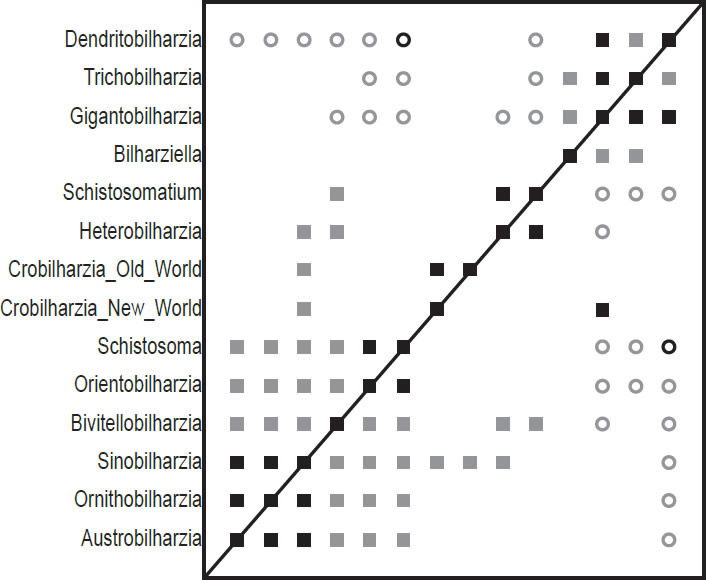
Fig. 1. This baraminic distance correlation (BDC) contains the taxa from Carmichael (1984), and is based on the characters used in his analyses. Filled squares indicate significant positive correlation and open circles indicate significant negative correlation. Black symbols represent bootstrap values greater than or equal to 90% and gray symbols represent values less than 90%.
The third dataset comes from Platt (1992) and consists of spirorchiid taxa (Spirhapalum, Plasmiorchis, Hemiorchis [now Plasmiorchis bengalensis], Spirorchis, Henotosoma [now Spirorchis haematobium], Haematotrema [now Spirorchis parvus], and Aphanospirorchis) and one clinostomid outgroup (Clinostomum). To this dataset, we added the taxon Schistosomatidae with values for each character. This dataset contained 13 morphological characters.
We ran all three datasets through BDISTMDS at a 0.95 character relevance cutoff, and no taxa were excluded in any analysis. In the Carmichael (1984) and Platt (1992) datasets, all characters were retained, but in the compiled dataset, 10 characters were excluded at the 0.95 relevance cutoff, leaving 19 characters for the analysis. BDC results from all three datasets were subjected to bootstrapping via BDISTMDS using 100 pseudoreplicates. We then used R (http://www.r-project.org) to visualize the BDC with bootstrap values. MDS results were converted to Kinemage files and were displayed with the program Mage (http://kinemage.biochem.duke.edu/software/mage.php).
Diversification Patterns within Schistosomatidae
The total number of kinds of intermediate hosts used by schistosomatids was estimated using the number of snail host families that serve as hosts for the parasites. Using a published phylogeny of Schistosomatidae (Lockyer et al. 2003), we recorded the method of diversification at each branch in the cladogram. Using each branch to represent a diversification event, we recorded the total number of diversification events and the number of each of the following methods of diversification: diversification without host switching, diversification with a change in intermediate (snail) host, and diversification with a change in definitive (vertebrate) host. Using the same phylogeny from Lockyer et al. (2003), the evidence for a Schistosomatidae holobaramin was explored, and the original hosts were predicted.
Mammal Schistosomes vs Bird Schistosomes
To determine the original hosts for members of Schistosomatidae, we analyzed the number of geographic locations that each parasite is found (North America, South America, Europe, Africa, southwest Asia and India, Russia, China and southeast Asia, and Australia and surrounding islands), the number of snail families that the parasite can parasitize, the fossil record of the intermediate hosts of the schistosomatid, and the pathology caused by the parasite. Each of these four features was grouped for all of the bird schistosomes and compared to the grouped data for mammal schistosomes.
We calculated the mean and standard deviation for the number of geographic regions in which a species is found for both bird schistosomes and mammal schistosomes, and compared the two using a Welch two-sample t-test. We employed a t-test comparing the average number of snail families infected by the parasite for both bird and mammal schistosomatids. In order to account for the possibility that the described species represent cryptic species and control for the amount of taxonomic study in each genus, we ran two more t-tests for the average values of the genera (instead of species) of Schistosomatidae according to the methods described above. Two linear regressions were employed to test the relationship between the number of snail families a species/genus of parasites infects and the number of geographic regions occupied by the parasite. We did this to better understand whether a larger distribution correlates with more snail families infected.
Data on the pathology of parasites and fossil records of intermediate hosts were obtained from the literature and compared between bird and mammal schistosomatids. We used these data to provide additional information on the original hosts of the Schistosomatidae parasites.
Results
Results of the baraminological analyses
The BDC results of the analysis of the Carmichael (1984) dataset (Fig. 1) show two major groups of significant positive BDC with some smaller pairs in between. The group of six taxa in the lower left corner all share significant positive BDC. Sinobilharzia within this cluster of schistosomatids correlates positively with both the New World and Old World Macrobilharzia and with Heterobilharzia. Besides these pairings, the two Macrobilharzia taxa only share positive BDC with each other. Bivitellobilharzia, also within the lower left group, shares significant positive BDC with Heterobilharzia and Schistosomatium, which in turn share positive BDC with one another. Some taxa in the lower left block share significant negative BDC with taxa in the upper right group, and all taxa in the lower left group share significant negative BDC with Dendritobilharzia in the upper right group as does Schistosomatium. Within the upper right group, Bilharziella, Gigantobilharzia, Trichobilharzia, and Dendritobilharzia all share significant positive correlation except for between Bilharziella and Dendritobilharzia. Very few positive BDC and only one negative BDC have high bootstrap values (>90%).
In general, the MDS results of the Carmichael (1984) analysis reflect the BDC results well (Fig. 2). The four taxa that were in the upper right block of the BDC (Fig. 1) are separated out from the other taxa. The rest of the taxa in Fig. 2 make a trigonal pattern in multidimensional space with couplets of taxa spread almost equidistantly from each other and from the center four taxa. Unexpectedly, Schistosoma and Orientobilharzia do not cluster with the center four taxa as they did in the BDC, but they are instead one of the three couplets. The 3D stress is 0.119, and the minimum stress is 0.0452 at six dimensions, which suggests that the MDS results are likely a reasonable representation of the actual pattern.
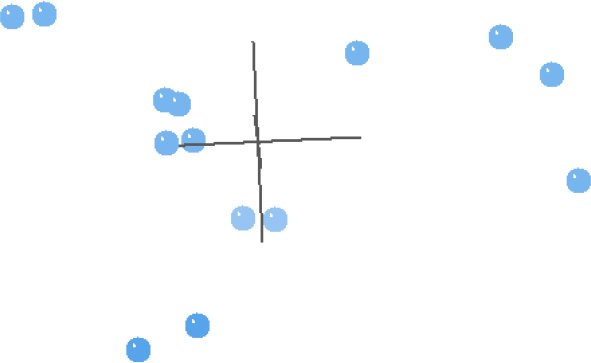
Fig. 2. This multidimensional scaling (MDS) contains taxa from Carmichael (1984), and is based on the characters used in his analyses.
The BDC results from the analysis of the compiled dataset (Fig. 3) show two major blocks of significant positive BDC with scattered significant positive BDC connecting the two blocks. Almost every taxon pair in the upper block (consisting of Schistosoma species, Ornithobilharzia canaliculata, and Austrobilharzia variglandis) shows significant positive BDC. Bivitellobilharzia nairi shares significant positive BDC with two of the taxa in the upper block, but does not share significant positive or negative BDC with any other taxa in that block. All taxa in this upper block except Schistosoma spindale share significant negative BDC with Griphobilharzia amoena, which in turn only shares positive correlation with three taxa in the lower block. The lower block of positive correlation is less coherent than the upper block, and certain taxa—notably Orientobilharzia turkestanicum—share significant positive correlation with taxa in the upper block. Schistosomatium douthitti and Bilharziella polonica are the only two taxa from the lower block to share significant negative correlation with more than one taxon from the upper block. Bootstrapping results are very poor for these BDC results.
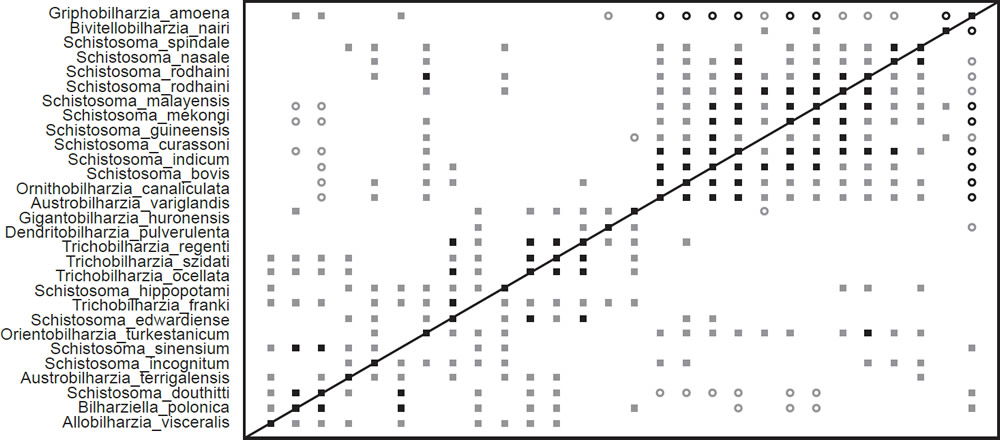
Fig. 3. The BDC results of the analysis of the Beltran et al. (2010) and Lockyer et al. (2003) dataset contains 27 taxa from Schistosomatidae and one taxon from outside the family: Griphobilharzia amoena. Filled squares indicate significant positive correlation and open circles indicate significant negative correlation. Black symbols represent bootstrap values greater than or equal to 90% and gray symbols represent values less than 90%.
The MDS results for this same analysis (Fig. 4) present a more defined picture than the BDC graph. Essentially, all of the taxa except for Griphobilharzia amoena are clustered together on the left side of Fig. 4, and there is a large gap separating Griphobilharzia amoena from the rest of the taxa. However, the 3D stress for these results is poor at 0.293, with the minimum stress of 0.065 occurring at 10 dimensions.
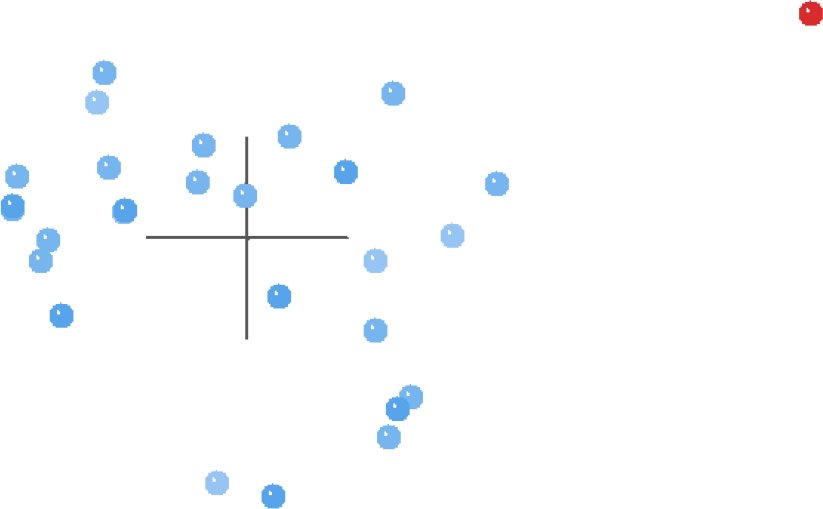
Fig. 4. MDS results of the analysis of the Beltran et al. (2010) and Lockyer et al. (2003) dataset. Schistosomatidae taxa are shown in blue and Griphobilharzia amoena is shown in red.
The spirorchiid BDC results (Fig. 5) show a series of three groups of positive correlation that each intersect at a single taxon. Haematotrema, Henostoma, and Spirorchis all share significant positive BDC, as do Spirorchis, Hemiorchis, and Plasmiorchis. Plasmiorchis also shares significant positive BDC with Spirhapalum. The spirorchiid taxon Aphanospirorchis does not share positive correlation with any other taxon in the analysis, but it shares significant negative correlation with the outgroup taxon Clinostomum. Clinostomum also shares negative correlation with Henotosoma, and the outgroup taxon Schistosomatidae shares negative correlation with Spirhapalum.
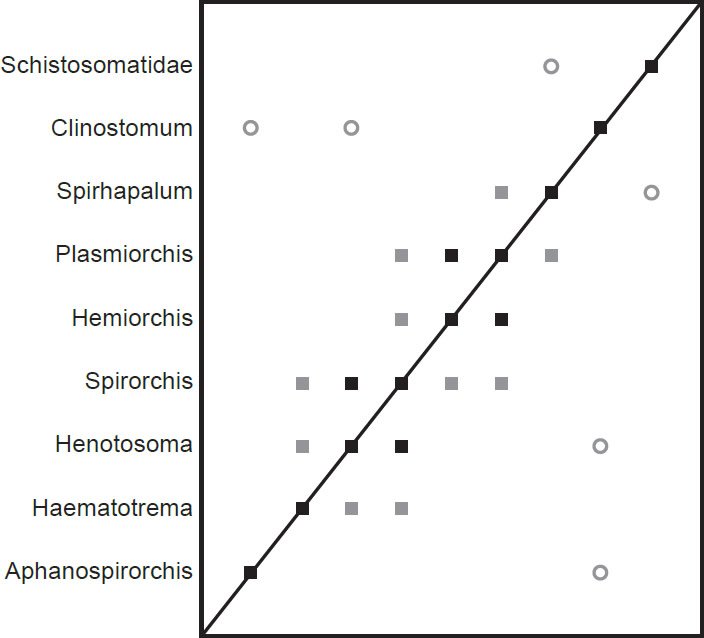
Fig. 5. BDC results of the analysis of the Platt et al. (1992) dataset. Filled squares indicate significant positive correlation and open circles indicate significant negative correlation. Black symbols represent bootstrap values greater than or equal to 90% and gray symbols represent values less than 90%.
The MDS results for the spirorchiid analysis (Fig. 6) show a single cluster of spirorchiid taxa (red) separated in multidimensional character space from Clinostomum (green) and from Schistosomatidae (blue), and both of these outgroup taxa are separated from each other by an even greater distance than either is separated from the spirorchiid cluster. The 3D stress for these results is 0.082, and the minimum stress is 0.077 at four dimensions, which suggests that this MDS graph is likely a very good representation of their arrangement in multidimensional space.
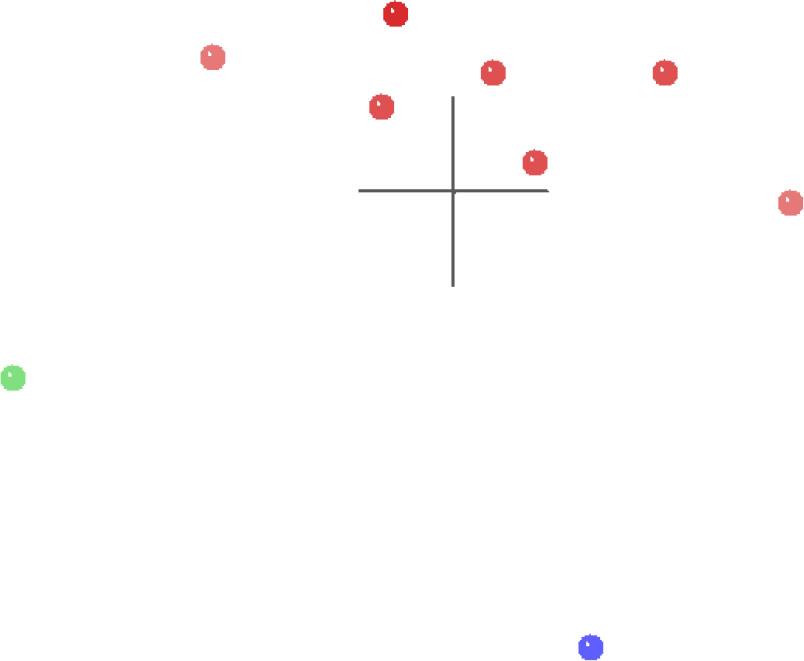
Fig. 6. MDS results of the analysis of the Platt et al. (1992) dataset. Spirorchiidae taxa are shown in red, Schistosomatidae is shown in blue, and Clinostomum is shown in green.
Diversification Patterns within Schistosomatidae
Members of family Schistosomatidae occur in at least 15 families of aquatic snails. If each family represents a separate baramin, the collection of species of schistosomes use at least 15 separately created organisms to complete their life cycles. Additionally, these parasites use dozens of families of birds and mammals as definitive or final hosts. We recorded 28 diversification events from the phylogeny of Schistosomatidae (Lockyer et al. 2003; Fig. 7). Of the 28 total points of diversification, these parasites diversify without entering a novel host 20 times. We found two instances of a switch in final hosts, and six occurrences of changing snail families. Diversification without accompanying host switch accounted for (71%) of the events in the phylogeny of Schistosomatidae. The most derived species of Schistosomatidae infect the fewest number of intermediate and definitive hosts, which are mammals.
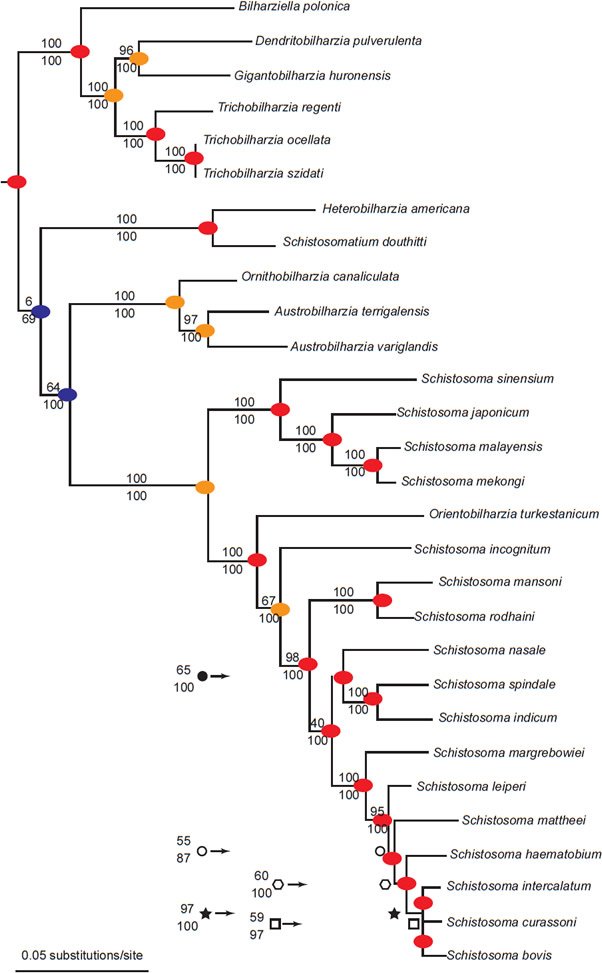
Fig. 7. This phylogeny is from Lockyer et al. (2003). A blue circle represents different final hosts in the two branches connected by the node. An orange circle represents different snail hosts in the two branches connected by the node. Finally, red circles indicate that both branches share at least one snail family as hosts and share the same class of definitive host (either bird or mammal).
The phylogeny from Lockyer et al. (2003) portrayed two groups of diversification (Fig. 7), with one group containing exclusively bird schistosomes and one group having both bird and mammal schistosomes. A phylogeny of 13 of the genera of Schistosomatidae with Griphobilharzia (a blood fluke of crocodilians) as an outgroup supported this history (Fig. 8). The second cladogram is built using morphological characters of the parasites, and had Austrobilharzia as a sister group to the rest of genera of the family. The first phylogeny from Lockyer et al. (2003) placed Austrobilharzia within the group containing both bird and mammal schistosomatids. Both phylogenies had bird parasites representing the most primitive members of Schistosomatidae. In both cladograms, most of the bird genera were more primitive than the first mammal genus (75% in both).
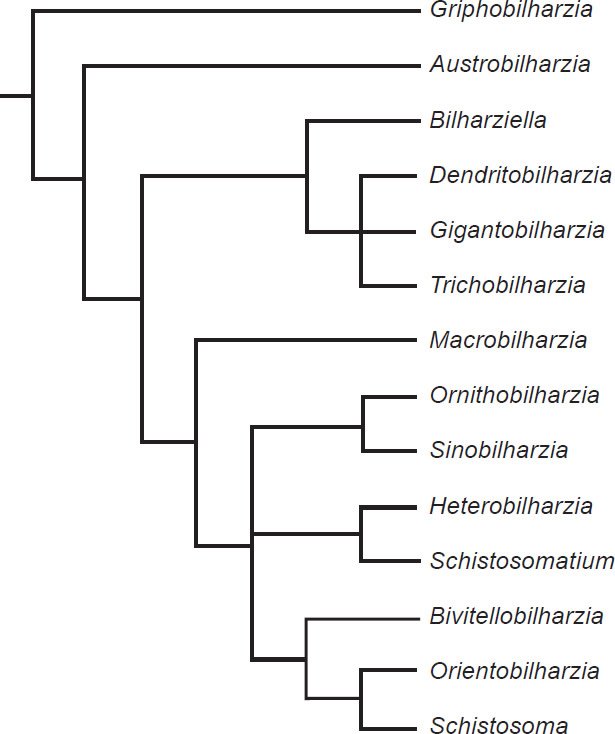
Fig. 8. This phylogeny is also taken from Lockyer et al. (2003). It is a copy of a cladogram from Morand and Müller-Graff (2000) that uses morphological characters to represent the diversification within Schistosomatidae.
Mammal Schistosomes vs Bird Schistosomes
Species of bird schistosomes use more snail families as intermediate hosts than species of mammal schistosomes (t = 2.3078, p = 0.04526), and occur in more geographic locations than do mammal parasites (t = 2.691, p = 0.02371). The same relationship holds for geographic locations occupied by schistosomatid genera. Bird parasitizing genera are found in more areas than genera that include mammal parasites (t = 4.5933, p = 0.004871). There was no statistically significant difference in the number of snail families used as intermediate hosts between bird blood flukes and mammal blood flukes (t = 1.4412, p = 0.1888). The number of geographic regions a parasite is found in increased with the number of snail families a parasite could use (F1,27 = 29.12, p < 0.001; Fig. 9). The variation in the number of snail families used as intermediate hosts explained 52% of the variation in the number of geographic regions. We found the same relationship among genera of schistosomes, with the distribution of the genus increasing with the number of snail families infected (F1,27 = 7.83, p = 0.009364; Fig. 10).
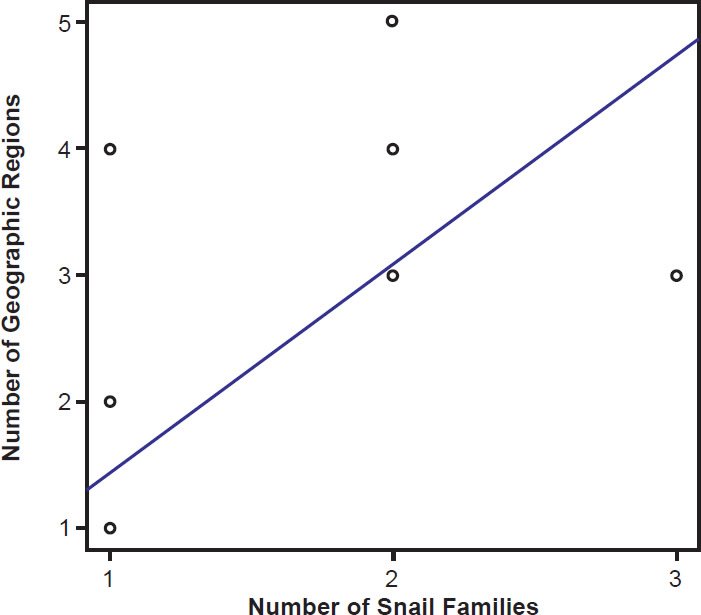
Fig. 9. The range of the schistosomatid is plotted against the host specificity. The blue line represents the line of best fit.
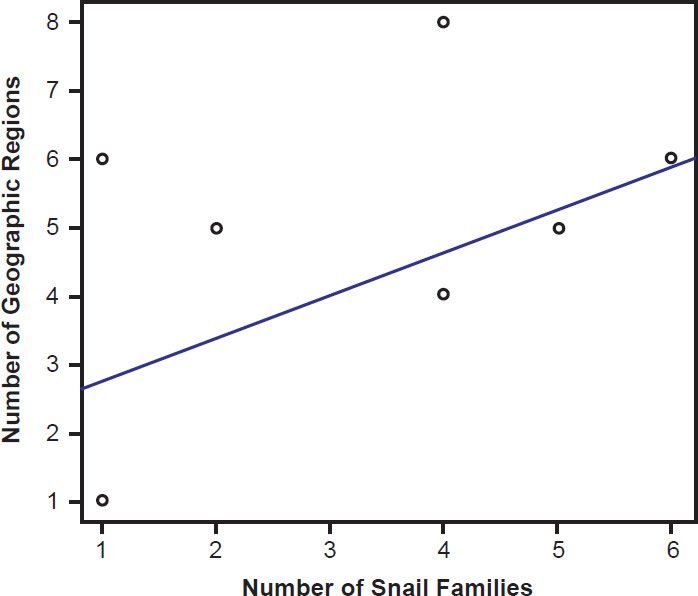
Fig. 10. The range of the genera of schistosomatid species is plotted against their host specificity. The blue line represents the line of best fit.
Bird schistomatids are believed to be less pathogenic to normal hosts than mammal parasites (Lichtenbergova and Horak 2012). While bird parasites can cause severe pathology in large numbers, most seem to cause less of an impact in the hosts than do mammal schistosomes (Wojcinski et al. 1987). The species Trichobilharzia regent invades the central nervous system (CNS) of waterfowl while migrating from the vascular system to the nasal passages, and can damage the nervous system (Lichtenbergová and Horák 2012). The damage caused by these species of parasites is temporary unless the parasite occurred in high numbers. Trichobilharzia regent can also cause significant pathology in rodents when it moves into the CNS and elicits an immune response (Lichtenbergová and Horák 2012). Schistosomatids that infect birds cannot fully mature in mammals and die prior to reaching sexual maturity (Wojcinski et al. 1987). The parasites of mammal vessels cause destruction in livestock, wildlife, and human populations (Snyder and Loker 2000). Five species of the genus Schistosoma cause intense pathology and collectively infect more than 200 million people worldwide (Lockyer et al. 2003; Snyder and Loker 2000).
Pulmonate and caenogastropod snails are infected by primitive bird and mammal schistosomes, with bird parasitizing species using more families of these snails than the mammal parasitizing species. The most common genus of snails infected by Schistosoma spp., Biomphalaria, did not occur in the present range of the parasite until the Pleistocene, demonstrating that diversification within Schistosoma is a recent phenomenon (Snyder and Loker 2000), and occurred after the Flood. The Pleistocene Epoch occurred towards the end of the Cenozoic Era, and likely represents post-Flood deposits (Anonymous 2009). Mace, Sims, and Wood (2003) proposed that the diversification within Schistosoma may have been complete prior to the Flood. Lockyer et al. (2003) demonstrated the presence of four groups of mammal infecting schistosomatids. Each of these groups occurs in a single family of snails: two groups of parasites in Lymnaeidae, one group in Planorbidae, and one in the caenogastropod family Pomatiopsidae. Schistosoma spp. may have shifted from using caenogastropods to pulmonate snails to enter into the African continent and achieve a great deal of diversification (Lockyer et al. 2003; Snyder and Loker 2000).
Discussion
All of the BDC results had few correlations with high bootstrap values, which suggests that these correlations are highly dependent upon the particular characters chosen for the original dataset (Wood 2008a). None of the datasets had large numbers of characters, with the spirorchiid dataset possessing only 13 characters. However, Wood (2008a) noted that the number of characters does not seem to have any statistically significant effect on the detectability of the baraminic status for a group of taxa. The characters in these datasets were not holistic, which is the desired state for baraminological analyses (Wood and Murray 2003). Nevertheless, Wood’s (2008a) crude measure for holism did not show a statistically significant difference between datasets of indeterminate baraminic status and those where baraminic status could be determined.
The BDC results of Carmichael (1984) (Fig. 1) seems to suggest discontinuity within the family Schistosomatidae between Dendritobilharzia, Trichobilharzia, Gigantobilharzia, and possibly Bilharziella on the one hand, and the other schistosomatid taxa on the other hand. Interestingly, the four taxa that are set apart are forms which are only found in birds. However, the MDS results
(Fig. 2) revealed a very peculiar geometry with a trigonal pattern for the taxa that are not solely found in birds. Peculiar geometries such as lines, tetrahedra, or the trigonal pattern seen here can help inform unusual BDC results. It is likely that the characters chosen for this dataset are biased (consciously or unconsciously) in some way (Wood 2005a). The characters used in the analyses are those that emphasize the dissimilarity among members of the family Schistosomatidae. This bias could increase the likelihood of finding schistosomatid species to be discontinuous with other species of the family. Although the BDC results suggest possible discontinuity within this family, the evidence for discontinuity is lacking in the MDS results. We conclude that there is no evidence for discontinuity within Schistosomatidae in this analysis.
The BDC results of the compiled dataset (Fig. 3) suggest continuity within Schistosomatidae to the exclusion of Griphobilharzia. This conclusion is strongly supported by the MDS graph (Fig. 4), which shows an obvious gap in multidimensional character space between Griphobilharzia and the schistosomatid cluster. Interestingly, Griphobilharzia is the only fluke in the analysis with an ectotherm for its final host (Crocodylus johnstoni—the Australian freshwater crocodile). Platt et al. (1991) described Griphobilharzia amoena as a schistosomatid, but they placed it in its own subfamily: Griphobilharziinae. It is now considered a closer relative of the Spirorchiidae—flukes that parasitize freshwater turtles. The BDC and MDS results taken together suggest that there is continuity between the members of Schistosomatidae, and there is discontinuity between Schistosomatidae and Griphobilharzia.
The modified dataset of Platt (1992) contained the taxon Schistosomatidae as an outgroup to the Spirorchiidae. The BDC results (Fig. 5) suggest continuity between most of the spirorchiids, and there is no evidence of continuity with either Clinostomum or Schistosomatidae. The MDS results (Fig. 6) suggest continuity within Spirorchiidae and discontinuity between Spirorchiidae, Clinostomum, and Schistosomatidae.
Combining the results of all three analyses, we present the following tentative conclusions. Firstly, we conclude that Schistosomatidae is a monobaramin because there is evidence of continuity within the family. Secondly, Schistosomatidae appears to be discontinuous from Griphobilharzia, Spirorchiidae, and Clinostomum, which, combined with its monobaraminic status, would make it a holobaramin. Thirdly, Spirorchiidae also appears to be a holobaramin because of its internal continuity and its discontinuity from Clinostomum and Schistosomatidae. Unfortunately, we were not able to test the relationship between Griphobilharzia and the spirorchiid taxa, although we hypothesize that Griphobilharzia will be found to be within the spirorchiid holobaramin. We assume this based on the entirely aquatic life cycle of the parasites, and several shared morphological features. Our conclusions are tentative mainly because the datasets were not holistic, and they had few characters. Although, as noted earlier, Wood (2008a) did not find a statistically significant difference in holism and character number between datasets that suggested holobaramins from those datasets that were indeterminate, we still think that more rigorous datasets on schistosomatids and spirorchiids should be compiled in the future to test our conclusions. The results of this study agree with and expand upon the conclusion by Mace, Sims, and Wood (2003) that Schistosomatidae is an apobaramin.
Mace, Sims, and Wood (2003) argued for the apobaramin status of Schistosomatidae, and described two monobaramins within Schistosoma: one with terminal spines on the eggs, and one with lateral spines on the egg. The researchers noted that both monobaramins might be untied in a single baramin. Our data suggest that all members of Schistosoma are in the same holobaramin, and all members of the family Schistosomatidae are also in the same holobaramin. Additionally, the phylogeny from Lockyer, et al. (2003) is congruent with many other phylogenies presented on Schistosomatidae that are based on other molecular features (Beltran et al. 2010), morphological features (Carmichael 1984), and reproductive behaviors (Beltran et al. 2010). Meyer, et al. (2007) pointed out how often phylogenies supporting universal common descent disagree (even when using the same characters). The lack of disagreement among the phylogenies for Schistosomatidae provides support for holobaramin status of schistosomatids. Phylogenies for schistosomatids contain two groups within the family (Lockyer et al. 2003). Both these groups contain bird parasites with nearly global distributions, further supporting the similarities among species within this family and the holobaramin status of these organisms.
We noticed that most of the diversification (20 out of 28 diversification events) within the schistosome kind occurred within the same snail host family (Fig. 7). If the different snail families represent separate snail holobaramins, much of the variation in the schistosomatid group seems to have generated within the same host kind. Additional research on other parasite groups will shed light on the efficacy of using the presence of similar parasites as evidence for holobaramin status of the hosts. Our data indicate that this may be fruitful and a useful tool for understanding organism kinds.
If all the blood flukes within Schistosomatidae are a single created kind, the life history and created intent of the baramin needs exploration. Mace, Sims, and Wood (2003) noted that these parasites might be invading the wrong hosts, creating the pathology that was not part of God’s design. Ingle (2015) suggested that parasites could have been designed to provide a mechanism for horizontal gene flow, connecting different organisms within an ecosystem. An organism that could move into and out of a variety of different hosts, without causing pathology would function well to move mobile genetic elements among species in a community. Our data (Figs. 9 and 10) suggest that bird schistosomatids are less selective when it comes to hosts than mammal schistosomes and are more globally distributed. These data suggest that this baramin may have been created to dwell in birds, connecting them with the ecosystem, and that shifting to mammal hosts reduced variability within the resulting group. The reduced pathology of bird schistosomes when compared to mammal schistosomes further supports this, although it is important to note that some bird schistosomatids (such as Trichobilharzia) do cause significant pathology (Lichtenbergová and Horák 2012). The parasites within this genus are considered more specialized (Lockyer et al. 2003) than other bird flukes and may be invading the wrong host or the wrong location in the host. Trichobilharzia spp. do migrate through the central nervous system, which is unique among the schistosomatids (Lichtenborgová and Horák 2012).
This study indicates that all blood flukes in family Schistosomatidae are part of a single created kind that has diversified significantly since creation. As the original group differentiated, more derived species became more host specific and more virulent. The bird parasites may represent the original form best, and are generalist parasites that cause less pathology than the more derived mammal blood flukes. Additional studies on the pathology of different schistosomatids and the characteristics of host immune responses are needed to better understand the intent and design of the schistosome kind (Mace, Sims, and Wood 2003).
Acknowledgments
We appreciate the valuable comments and suggestions made by Christian Hayes as he edited this manuscript for us. The anonymous reviewers provided useful insights that strengthened the arguments in this manuscript.
References
Al-Kandari, W. Y., S. A. Al-Bustan, A. M. Isaac, B. A. George, and B. S. Chandy. 2012. “Molecular Identification of Austrobilharzia Species Parasitizing Cerithidea cingulata (Gastropoda: Potamididae) from Kuwait Bay.” Journal of Helminthology 86 (4): 470–78.
Anonymous. 2009. ‘The Stones Cry Out.” https://answersingenesis.org/geology/geologic-time-scale/the-stones-cry-out/.
Barber, I., H. A. Wright, S. A. Arnott, and R. J. Wootton. 2008. “Growth and Energetics in the Stickleback-Schistocephalus Host-Parasite System: A Review of Experimental Infection Studies.” Behaviour 145:647–68.
Beltran, S., Y. Desdevises, J. Portela, and J. Boissier. 2010. “Mating System Drives Negative Associations Between Morphological Features in Schistosomatidae.” BMC Evolutionary Biology 10: 245.
Carmichael, A. C. 1984. Phylogeny and Historical Biogeography of the Schistosomatidae. East Lansing, Michigan: Michigan State University.
Devkota, R., S. V. Brant, A. Thapa, and E. S. Loker. 2014. “Sharing Schistosomes: The Elephant Schistosome Bivitellobilharzia nairi Also Infects the Greater One-Horned Rhinoceros (Rhinoceros unicornis) in Chitwan National Park, Nepal.” Journal of Helminthology 88 (1): 32–40.
Francis, J. W. 2009. “Symbiosis, Relationship and the Origin of Species.” In vol. 5 of Genesis Kinds: Creationism and the Origin of Species. Edited by T. C. Wood and P. A. Garner, 163–92). Eugene, Oregon: Wipf and Stock.
Ingle, M. E. 2015. “Parasitology and Creation.” Answers Research Journal 8: 65–75. https://answersingenesis.org/biology/disease/parasitology-and-creation/.
Khalil, L. F. 2002. “Family Schistosomatidae Stiles & Hassall, 1898.” In Keys to the Trematoda. Edited by D. I. Gibson, A. Jones, and R. A. Bray, 419–32. Wallingford, United Kingdom: CABI Publishing.
Kortet, R., A. V. Hedrick, and A. Vainikka. 2010. “Parasitism, Predation and the Evolution of Animal Personalities.” Ecology Letters 13 (12): 1449–58.
Lichtenbergová, L., and P. Horák. 2012. “Pathogenicity of Trichobilharzia spp. for Vertebrates.” Journal of Parasitology Research 2012: 761968.
Lockyer, A. E., P. D. Olson, P. Ostergaard, D. Rollinson, D. A. Johnston, S. W. Attwood, V. R. Southgate et al. 2003. “The Phylogeny of the Schistosomatidae Based on Three Genes with Emphasis on the Interrelationships of Schistosoma Weinland, 1858.” Parasitology 126 (3): 203–24.
Lozano, G. A. 1998. “Parasitic Stress and Self-Medication in Wild Animals.” Advances in the Study of Behavior 27: 291–317.
Mace, S. R., B. A. Sims, and T. C. Wood. 2003. “Fellowship, Creation, and Schistosomes.” Acts & Facts 32 (3).
Meyer, S. C., S. Minnich, J. Moneymaker, P. A. Nelson, and R. Seelke. 2007. Explore Evolution: The Arguments for and Against Neo-Darwininism. London, England: Hill House Publishers.
Morand, S., and C. D. Müller-Graf. 2000. “Muscles or Testes? Comparative Evidence for Sexual Competition Among Dioecious Blood Parasites (Schistosomatidae) of Vertebrates.” Parasitology 120 (1): 45–56.
Platt T. R. 1992. “A Phylogenetic and Biogeographic Analysis of the Genera of Spirorchinae (Digenea: Spirorchidae) Parasitic in Freshwater Turtles.” Journal of Parasitology 78 (4): 616–29.
Platt, T. R., D. Blair, J. Purdie, and L. Melville. 1991. “Griphobilharzia amoena n. gen., n. sp. (Digenea: Schistosomatidae), a Parasite of the Freshwater Crocodile Crocodylus johnstoni (Reptilia: Crocodylia) from Australia, with the Erection of a New Subfamily, Griphobilharziinae.” Journal of Parasitology 77 (1): 65–8.
Robar, N., D. L. Murray, and G. Burness. 2011. “Effects of Parasites on Host Energy Expenditure: The Resting Metabolic Rate Stalemate.” Canadian Journal of Zoology 89 (11): 1146–55.
Robinson, D. A., and D. P. Cavanaugh. 1998. “A Quantitative Approach to Baraminology With Examples from the Catarrhine Primates.” Creation Research Society Quarterly 34 (4): 196–208.
Schuster, R. K., J. A. Aldhoun, and D. O’Donovan. 2014. “Gigantobilharzia melanoidis n. sp. (Trematoda: Schistosomatidae) from Melanoides tuberculata (Gastropoda: Thiaridae) in the United Arab Emirates.” Parasitology Research 113 (3): 959–72.
Shostak, A. W. 2009. “Tapeworm (Hymenolepis diminuta) Infection in Flour Beetles (Tribolium confusum): Does it Cause a Trade-Off Between Host Fecundity and Egg Size?” Canadian Journal of Zoology 87 (11): 1087–95.
Shostak, A. W. 2012. “Sequential and Concurrent Exposure of Flour Beetles (Tribolium confusum) to Tapeworms (Hymenolepis diminuta) and Pesticide (Diatomaceous Earth).” Journal of Parasitology 98 (3): 453–9.
Snyder, S. D., and E. S. Loker. 2000. “Evolutionary Relationships among the Schistosomatidae (Platyhelminthes: Digenea) and an Asian Origin for Schistosoma.” Journal of Parasitology 86 (2): 283–8.
Thomas, F., R. Poulin, J.-F. Guégan, Y. Michalakis, and F. Renaud. 2000. “Are there Pros as well as Cons to being Parasitized.” Parasitology Today 16 (12):533–6.
Webster, B. L., and D. T. J. Littlewood. 2012. “Mitochondrial Gene Order Change in Schistosoma (Platyhelminthes: Digenea: Schistosomatidae).” International Journal for Parasitology 42 (3): 313–21.
Wojcinski, Z. W., I. K. Barker, D. B. Hunter, and H. Lumsden. 1987. “An Outbreak of Schistosomiasis in Atlantic Brant Geese, Branta bernicla hrota.” Journal of Wildlife Diseases 23 (2): 248–55.
Wood, T. C. 2005a. “A Creationist Review and Preliminary Analysis of the History, Geology, Climate, and Biology of the Galápagos Islands.” Center for Origins Research Issues in Creation 1.
Wood, T. C. 2005b. “Visualizing Baraminic Distances Using Classical Multidimensional Scaling.” Origins (GRI) 57: 9–29.
Wood, T. C. 2008a. “Animal and Plant Baramins.” Center for Origins Research Issues in Creation 3.
Wood, T. C. 2008b. BDISTMDS software, v. 2.0. Center for Origins Research, Bryan College. Distributed by the author.
Wood, T. C. and M. J. Murray. 2003. Understanding the Pattern of Life: Origins and Organization of the Species. Edited by K. Wise. Nashville, Tennessee: Broad & Holman Publishers.