The views expressed in this paper are those of the writer(s) and are not necessarily those of the ARJ Editor or Answers in Genesis.
Abstract
Little baraminological research has been conducted on fossil groups, and even less research has considered dinosaurs. In this study, I analyzed a theropod dinosaur group, Tyrannosauroidea, through the use of statistical baraminology. Four cladistic datasets were reanalyzed through baraminic distance correlation (BDC) and multidimensional scaling (MDS). Because many of the supposed intermediary forms are poorly known, they were excluded from the four analyses. In order to further test the baraminic status of Tyrannosauroidea, I performed another analysis of one of the datasets, encompassing more taxa, but with very few characters. Lastly, a subset of the Xu et al. (2012) dataset was analyzed in order to better understand the relationships among the “basal” tyrannosauroids.
As a result of these analyses, I arrived at four conclusions. Firstly, there is strong positive BDC correlation and close MDS grouping within the family Tyrannosauridae. Secondly, there is a strong negative BDC correlation and visually distinct gap in the MDS between Tyrannosauridae and the more distant members of the superfamily Tyrannosauroidea and between Tyrannosauridae and non-tyrannosauroid theropod outgroup. Thirdly, there is fairly strong evidence of positive BDC correlation and MDS clustering between Tyrannosauridae, five close members of Tyrannosauroidea (Bistahieversor, Appalachiosaurus, Dryptosaurus, Raptorex, Xiongguanlong), and possibly with the more distant tyrannosauroid Eotyrannus. Fourthly, there is negative BDC correlation and a distinct visual MDS gap between the Tyrannosauridae with some close tyrannosauroids and the more distant tyrannosauroids and outgroup taxa. Based on these results, I determine that Tyrannosauridae is monobaraminic and, along with Bistahieversor, Appalachiosaurus, Dryptosaurus, Raptorex, Xiongguanlong, and possibly Eotyrannus, is holobaraminic with respect to other members of Tyrannosauroidea and all other dinosaurs. The position of Eotyrannus in the biological trajectory of this holobaramin suggests that tyrannosaurs may have originally had smaller skulls and longer arms in proportion to their bodies and three-fingered hands. Because many of the “basal” tyrannosauroids are poorly known, further discoveries of more complete specimens may place them within this holobaramin, or they may provide a link between Tyrannosauridae and other theropods. This article marks the first dinosaur holobaramin defined via statistical baraminology, and the results of this study suggest that the taxa Dinosauria, Saurischia, and Theropoda are polybaraminic.
Keywords: Tyrannosauridae, baraminology, multidimensional scaling, baraminic distance correlation, Tyrannosauroidea, dinosaur, theropod
Introduction
Review of Dinosaur Baraminology
In order to determine the various original created kinds, creationists have begun to expand baraminological research to organisms only known through fossils. Previous studies have included fossil equids (Cavanaugh, Wood, and Wise 2003), archaeocetes (Mace and Wood 2005), hominids (Wood 2010), caseids (Aaron 2014), and Jurassic and Cretaceous Avialae (Garner, Wood, and Ross 2013). The criteria that have been used to determine the baraminic status of a fossil organism include morphology and stratigraphy (Cavanaugh, Wood, and Wise 2003). Few investigations into the baraminology of non-avian dinosaur (hereafter called “dinosaur”) groups have been undertaken. Wood (2011), in his response to Senter (2010), analyzed maniraptoran theropods, but he did not make any statements regarding their holobaraminic status. Cavanaugh (2011) reanalyzed the same character set as Senter (2010) and Wood (2010), this time using ANOPA, and concluded that there were no clear gaps between clouds of theropod taxa. Wood, Ross, and Garner (2011) studied several dinosaur character sets in order to test if baraminic distance correlation could detect discontinuities among fossil groups. In their research, they recognized several dinosaur groups as apobaramins, including the theropod groups Coleophysoidea, Neoceratosauria, Tyrannosauroidea, and Oviraptorsauria. Finally, Garner, Wood, and Ross (2013) included maniraptoran theropods in their analysis of Jurassic and Cretaceous Avialae. Presently, no dinosaur groups have been assigned to holobaramins. The holobaramin is the basic unit of baraminology and is defined as a group of organisms in which each member shares continuity with at least one other member, but the group is bounded by discontinuity from all other organisms (Wood and Murray 2003, p. 222). Determining dinosaur holobaramins will allow creationists to better understand the relationships between different groups of dinosaurs, the extent and limits of diversification and intrabaraminic potential in organisms, and the pattern of design in biology, which will lead to a deeper appreciation of God and His creation.
Introduction to the Tyrannosauroidea
A review of the previous work on Tyrannosauroidea in the conventional literature provides a useful foundation for this study. The superfamily Tyrannosauroidea has a large stratigraphic range stretching down to the Middle Jurassic (Fiorillo and Tykoski 2014, Fig. 6). The geographic range of taxa within Tyrannosauroidea includes North America, Europe, Asia (Holtz 2004; Zanno and Makovicky 2011), and possibly South America (Porfiri et al. 2014). Tyrannosauridae is a family within Tyrannosauroidea that contains animals similar in appearance to Tyrannosaurus rex, which is possibly the most popular dinosaur species of all time. In contrast to Tyrannosauroidea, Tyrannosauridae has a very restricted geographic and stratigraphic range. Tyrannosaurids are known exclusively from the Campanian and Maastrichtian stages of the uppermost Cretaceous system (Holtz 2004) from North America and eastern and central Asia (Holtz 2004; Loewen et al. 2013).
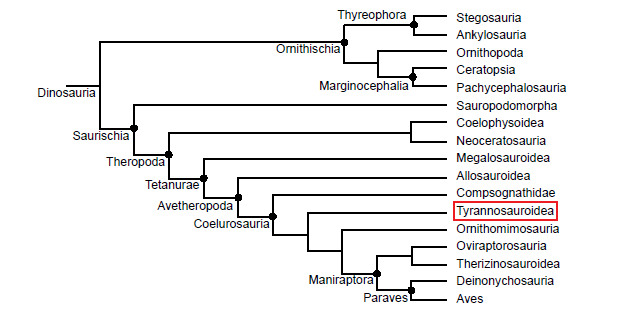
Fig. 1. Simplified cladogram of Dinosauria modified from Butler, Upchurch, and Norman (2008) and Holtz and Osmólska (2004). Taxon Tyrannosauroidea is surrounded by a red box.
Taxonomically, Tyrannosauroidea is located within Theropoda, Saurischia, and Dinosauria (Fig. 1). According to Holtz (2004), members of the group Tyrannosauroidea (Fig. 2) share several features including “an accessory broad, ventral hook-like projection from the preacetabular blade of ilium,” a “shaft of the ischium [that is] much more slender than the pubis shaft,” and “a median vertical ridge on the external surface of the ilium.” Members of the family Tyrannosauridae, found within Tyrannosauroidea, all have remarkably short forelimbs on robust pectoral girdles. All possess only two full fingers and relatively large and robust skulls in comparison to their bodies. They also possess a “distinct cornual process on the lacrimal” and “rugose texture on the fused nasals” (Li et al. 2010). Tyrannosaurids are very similar in their morphology, differing little from species to species (Paul 1989, p. 325). Because of this, Paul (1989, p. 337) lumped Tarbosaurus bataar and Daspletosaurus torosus into the genus Tyrannosaurus, however, this proposal has not been accepted by most researchers. Holtz (2004) states that the family Tyrannosauridae is made up of two groups: Tyrannosaurinae and Albertosaurinae. However, Lü et al. (2014) have recognized a third group, Alioramini, which contains three Asian longirostrine forms. In the past, some researchers included another group, Aublysodontinae, in Tyrannosauridae based on the shared trait of teeth lacking serrations (Holtz 2001; Paul 1989, p. 324). However, the genera assigned to Aublysodontinae (Aublysodon, Stygivenator, and Shanshanosaurus) are most likely juveniles of known tyrannosaurid genera (Currie, Hurum, and Sabath 2003). Additionally, the type material of Aublysodon has been lost, and the lack of serrations on the teeth of aublysodontines may be taphonomic rather than an actual anatomical feature (Holtz 2004).
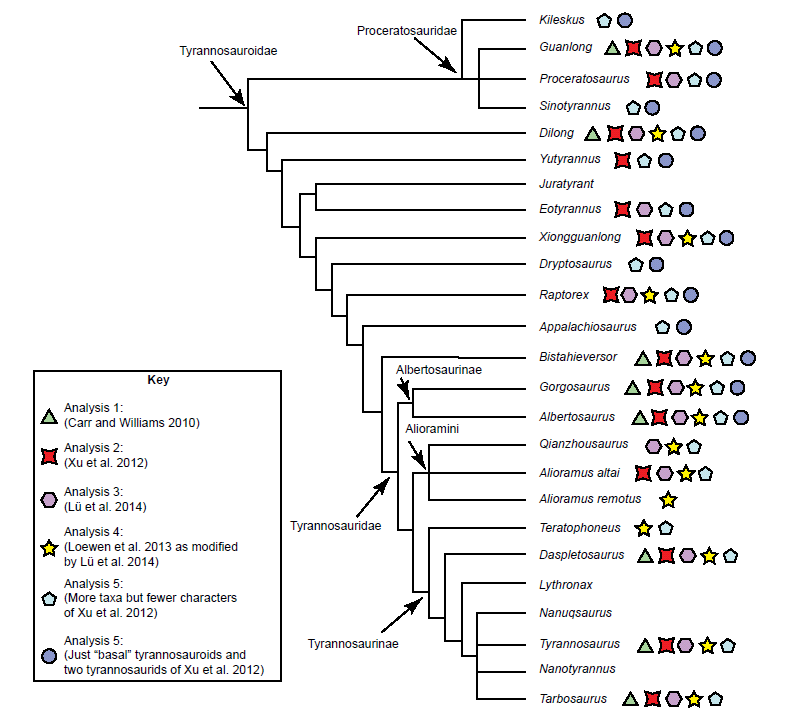
Fig. 2. Cladogram of Tyrannosauroidea modified from Lü et al. (2014) with the addition of taxa not in that publication, such as Yutyrannus (Xu et al. 2012), Lythronax (Loewen et al. 2013), Nanuqsaurus (Fiorillo and Tykoski 2014), and Nanotyrannus (Currie 2003). These taxa were placed in this cladogram based on placement in their respective publications. The presence of a taxon in a baraminological analysis is indicated by one of the symbols in the key.
The first major discovery of what some considered to be a possible ancestor to Tyrannosauridae was in 2001 with the finding of the Lower Cretaceous Eotyrannus lengi, named from an incomplete, disarticulated but associated, juvenile or subadult specimen from England (Hutt et al. 2001). This dinosaur had several features in common with tyrannosaurids, which led the authors to conclude that it was transitional from coelurosaurian theropods to tyrannosaurids. In 2004, Xu et al. described a new Lower Cretaceous theropod which they named Dilong paradoxus. This theropod shared several characteristics with tyrannosaurids and Eotyrannus but was very small and covered with “protofeathers.” More tyrannosauroids have since been described, including the Upper Cretaceous Appalachiosaurus montgomeriensis and a Lower Cretaceous crested form named Guanlong wucaii. Although originally described as a tyrannosauroid, Guanlong was recovered by Carr and Williamson (2010) as the sister taxon to Monolophosaurus in their phylogenetic analysis, which is in agreement with a previous claim by Carr (2006) that Guanlong may be either the sister taxon to Monolophosaurus or a subadult of that genus. In contrast, Brusatte et al. (2010a) concluded the holotype of Guanlong is an adult and that it is in fact a tyrannosauroid. All recent phylogenetic studies place Guanlong within Tyrannosauroidea (Brusatte et al. 2010b; Brusatte and Benson 2013; Loewen et al. 2013; Xu et al. 2012). Guanlong is typically placed in a group called Proceratosauridae, which also contains Proceratosaurus, Kileskus, and Sinotyrannus (Lü et al. 2014). Additionally, some theropods, such as Dryptosaurus, have been placed into Tyrannosauroidea many years after their discoveries (Holtz 2004).
Terminology and Methods in Statistical Baraminology
In this study, I report the results of a baraminological analysis of four published cladistic datasets of tyrannosauroids utilizing BDISTMDS version 2.0, a software program that performs two functions. Firstly, it measures baraminic distance via linear regression to determine the statistical significance of the similarity between two organisms—a process called baraminic distance correlation (BDC). Secondly, it plots taxa as points in three-dimensional space via classical multidimensional scaling (MDS). As the many dimensions of baraminic distance are reduced to three, distortion occurs. BDISTMDS measures the amount of distortion for observed baraminic distances that occurs with this reduction in the number of dimensions, a concept called stress. Smaller values of stress correlate with a better fit for observing baraminic distances in multidimensional space. For a more detailed explanation of stress and the application of MDS to baraminic distances, see Wood (2005b). In BDC, significant positive correlation between organisms implies continuity and probable inclusion in the same monobaramin, whereas significant negative correlation implies discontinuity between the organisms (Robinson and Cavanaugh 1998). In MDS, a point represents a taxon existing in multidimensional character space. Points that cluster closely together in character space imply continuity, whereas large gaps between clusters imply discontinuity. Dissimilar outgroup organisms will often share significant positive correlation in BDC and cluster together in MDS because of their similar scores in character datasets. Characters are typically chosen in order to understand ingroup relationships, thus the only scores assigned to outgroup taxa will often be the absence of ingroup features. The presence of only absent or “primitive” characters for outgroup taxa results in all outgroup and “basal” ingroup taxa often possessing identical or almost identical scores.
The assignment of taxa to a holobaramin is ideally supported by a holistic suite of evidences. Unfortunately, with fossil organisms researchers are limited in the available categories of evidence. In this study, the data for these theropods consists entirely of skeletal and dental characters. However, the skeletons of many tyrannosaurids are very well-known, which permits for a great variety of skeletal and dental characteristics to be used in this analysis. Comparing varieties of bones and teeth is valuable, and the breakdown of characters by skeletal portion from these datasets is displayed in Table 1. Even with excellent skeletal and dental data, a great deal of information about fossil organisms is missing; thus, any conclusion based on such data is tentative. For example, McConnachie and Brophy (2008) evaluated a dataset of landfowl using BDC and MDS, through which they determined their taxa fell into four holobaramins, but hybridization was found to occur between three of those suggested holobaramins. Nevertheless, the use of statistical baraminology in the analysis of fossil taxa gives creationists a good starting place in the process of determining created kinds and Ark kinds. Since it is unlikely that new kinds of data on fossil taxa will be available in the future, closer approximations of holobaramins must come through the discovery of more complete fossils and improved methods.
Table 1. Breakdown of characters in datasets by body portion.
Carr and Williamson (2010) | Lü et al. (2014) | Loewen et al. (2013) from Lü et al. (2014) | Xu et al. (2012) | |
---|---|---|---|---|
Cranial | 215 | 197 | 288 | 195 |
Dental | 4 | 7 | 15 | 6 |
Axial | 9 | 31 | 57 | 31 |
Pectoral | 2 | 7 | 14 | 7 |
Forelimb | 9 | 18 | 23 | 18 |
Pelvic | 22 | 32 | 46 | 26 |
Hindlimb | 11 | 25 | 58 | 24 |
Body Size | 1 | 0 | 0 | 0 |
Integument | 1 | 0 | 0 | 0 |
Total | 274 | 317 | 501 | 307 |
Materials and Methods
Four cladistic datasets of tyrannosaurs were analyzed through statistical baraminology. Carr and Williamson (2010) published a description of Bistahieversor, which included a dataset of 274 morphological characters for 26 fossil taxa and unnamed fossil specimens. Twenty-one of the taxa and specimens they included had been previously assigned to Tyrannosauroidea (Dryptosaurus, Appalachiosaurus, Bistahieversor, Albertosaurus [Gorgosaurus] libratus, Albertosaurus sarcophagus, a new genus from Utah [Teratophoneus], Daspletosaurus spp., Daspletosaurus sp. [MOR 590], Tyrannosaurus rex, Tyrannosaurus [Tarbosaurus] bataar, cf. Alectrosaurus [GIN 100/50, 100], Alioramus remotus, Bagaraatan, Aviatyrannis, Dilong, Guanlong, Eotyrannus, Stokesosaurus, Illiosuchus, Tanycolagreus, and Coelurus), and five were chosen as outgroup comparisons (Monolophosaurus jiangi, Allosaurus fragilis, Velociraptor mongoliensis, Sinosauropteryx prima, Coelophysis bauri). Their phylogenetic analysis resulted in Guanlong appearing outside Tyrannosauroidea as the sister taxon to Monolophosaurus. Additionally, they did not recover Coelurus as a basal tyrannosauroid in their phylogenetic analysis.
The Brusatte et al. (2010b) dataset consists of 307 morphological characters for 23 taxa. Of the 23 taxa, 19 are ingroup tyrannosauroids (Kileskus, Guanlong, Proceratosaurus, Dilong, Eotyrannus, Stokesosaurus, Xiongguanlong, Raptorex, Dryptosaurus, Appalachiosaurus, Albertosaurus, Gorgosaurus, Alioramus, Daspletosaurus, Tarbosaurus, Tyrannosaurus, Bistahieversor, an unnamed specimen from Utah (Teratophoneus), and Sinotyrannus), and four are outgroup taxa (Allosaurus, Dromaeosauridae, Ornithomimosauria, and Compsognathidae). Xu et al. (2012) described the discovery of a tyrannosauroid with “protofeathers,” Yutyrannus, which they coded to match the Brusatte et al. (2010b) matrix in order to understand its phylogenetic position. For the remainder of the paper, this combined dataset will be referred to as the Xu et al. (2012) dataset.
Lü et al. (2014), in describing the new longirostrine tyrannosaurid Qianzhousaurus, published a dataset consisting of 317 morphological characters for 25 taxa based on a 2013 analysis by Brusatte and Benson. Of the 25 taxa, 21 are ingroup tyrannosauroids (Kileskus, Guanlong, Proceratosaurus, Dilong, Eotyrannus, Juratyrant, Xiongguanlong, Raptorex, Dryptosaurus, Appalachiosaurus, Albertosaurus, Gorgosaurus, Alioramus altus, Alioramus remotus, Qianzhousaurus, Daspletosaurus, Tarbosaurus, Tyrannosaurus, Bistahieversor, Teratophoneus, and Sinotyrannus), and four are outgroup taxa (Allosaurus, Maniraptora, Ornithomimosauria, and Compsognathidae).
In the same paper, Lü et al. (2014) placed Qianzhousaurus into a cladistic dataset created by Loewen et al. (2013). This analysis will hereafter be referred to as Loewen et al. (2013) in order to avoid confusion with the previous Lü et al. (2014) analysis, despite the changes made by Lü et al. These authors included 501 characters and 54 taxa. Of the 54 taxa, 27 were ingroup tyrannosauroids (Proceratosaurus, Kileskus, Guanlong, Sinotyrannus, Juratyrant, Stokesosaurus, Dilong, Eotyrannus, Bagaraatan, Raptorex, Dryptosaurus, Xiongguanlong, Alectrosaurus, Alioramus altai, Alioramus remotus, Gorgosaurus, Albertosaurus, Dinosaur Park Formation tyrannosaurid B, Daspletosaurus, Two Medicine Formation tyrannosaurid, Teratophoneus, Bistahieversor, Lythronax, Tyrannosaurus, Tarbosaurus, Zhuchengtyrannus, and Qianzhousaurus) and 28 were outgroup theropods (Tawa, Coelophysis, Dilophosaurus, Ceratosaurus, Dubreuillosaurus, Piatnitzkysaurus, Eustreptospondylus, Monolophosaurus, Sinraptor, Allosaurus, Tanycolagreus, Coelurus, Sinosauropteryx, Compsognathus, Juravenator, Scipionyx, Ornitholestes, Haplocheirus, Utahraptor, Deinonychus, Linheraptor, Velociraptor, Archaeopteryx, Pelecanimimus, Harpymimus, and Gallimimus). These authors did not consider Tanycolagreus to be a tyrannosauroid, whereas Carr and Williamson (2010) considered Tanycolagreus to be a basal tyrannosauroid.
Baraminological analysis was performed using BDISTMDS v. 2.0 at http://www.coresci.org/bdist.html (Wood 2008). BDISTMDS calculated bootstrap values based on 100 pseudoreplicates. Visualization of the BDC with bootstrap values was made possible by R (http://www.r-project.org). MDS results were converted to Kinemages for display via Mage (http://kinemage.biochem.duke.edu/software/mage.php). Several taxa were omitted from both analyses due to incomplete character states. All analyses were subjected to a 0.75 character relevance cutoff. Poorly represented taxa were eliminated to raise the number of characters used to calculate baraminic distances to at least 50% from the respective dataset. This means that not all analyses had the same taxic relevance cutoff.
In the analysis of Carr and Williamson (2010), a taxic relevance cutoff of 0.39 was selected, which excluded 12 taxa (Dryptosaurus, Appalachiosaurus, a new genus from Utah [Teratophoneus], cf. Alectrosaurus [GIN 100/50, 100], Alioramus remotus, Bagaraatan, Aviatyrannis, Eotyrannus, Stokesosaurus, Illiosuchus, Tanycolagreus, and Coelurus), and left all five outgroup taxa and nine of the ingroup taxa in the analysis. The 0.75 character relevance cutoff excluded 106 characters, leaving 168 for use in this analysis.
In the analysis of Xu et al. (2012), a taxic relevance cutoff of 0.32 was selected, which excluded six taxa (Appalachiosaurus, Dryptosaurus, Kileskus, Sinotyrannus, Stokesosaurus, and Teratophoneus), leaving 18 taxa for the analysis (four outgroup taxa and 14 ingroup taxa). The character relevance cutoff of 0.75 resulted in exclusion of 146 characters, leaving 161 characters for this analysis.
For analysis of the Lü et al. (2014) dataset, a taxic relevance cutoff of 0.32 was selected, which excluded seven taxa (Appalachiosaurus, Alioramus remotus, Dryptosaurus, Juratyrant, Kileskus, Sinotyrannus, and Teratophoneus). Eighteen taxa were used in the analysis, and at a character relevance cutoff of 0.75, 163 characters were used to the exclusion of 154.
In the Loewen et al. (2013) analysis, a taxic relevance cutoff of 0.5 was selected, which excluded 19 of the original 55 taxa (Alectrosaurus, Appalachiosaurus, Bagaraatan, Coelurus, Dryptosaurus, Dubreuillosaurus, Eotyrannus, Juratyrant, Kileskus, Lythronax, Pelecanimimus, Piatnitzkysaurus, Proceratosaurus, Shenzhousaurus, Sinotyrannus, Stokesosaurus, Tanycolagreus, Utahraptor, and Zhuchengtyrannus), leaving 36 taxa for the analysis. Of the remaining taxa, 20 were outgroup taxa, and 16 were ingroup tyrannosauroids. At a 0.75 character relevance cutoff, 184 characters were excluded, and 317 characters were used.
Because of the methods used to analyze these tyrannosauroid datasets, some of the taxa considered transitional from non-tyrannosauroid theropods to Tyrannosauridae were excluded as they are poorly known. As these taxa are very important in understanding the relationship of tyrannosauroids to the rest of the Theropoda, I conducted another analysis containing as many taxa as possible at a 0.75 character relevance cutoff. This fifth analysis contained only 57 characters out of the 307 in Xu et al. (2012), but it included all of the taxa except Aviatyrannis, Juratyrant, and Stokesosaurus, which were too poorly represented to use.
In order to better understand the relationships between the various non-tyrannosaurid tyrannosauroid taxa, I determined to focus on a subset of the Xu et al. (2012) dataset, removing from the analysis the taxa farthest from the non-tyrannosaurid tyrannosauroids on both ends (distant tyrannosaurids on one end and the outgroup taxa on the other). This subset technique was first used by Wood (2005a) who wrote, “Removal of taxa that dominate correlation calculations might reveal significant negative or positive correlation patterns undetectable in the first dataset.” The technique has since been utilized by Wood (2011) and Aaron (2014). Once again using the Xu et al. (2012) dataset, I excluded the tyrannosaurid taxa Teratophoneus, Alioramus, Daspletosaurus, Tarbosaurus, and Tyrannosaurus and all of the outgroup taxa. This left only the two tyrannosaurid taxa (Albertosaurus and Gorgosaurus) that in other analyses often group with closer non-tyrannosaurid tyrannosauroids, as well as the other tyrannosauroid taxa (except for Juratyrant, Stokesosaurus, and Aviatyrannis, which were too poorly known to include). The character relevance cutoff was again set to 0.75, resulting in 34 characters to be utilized in the analysis.
All corrected baraminic matrices were then analyzed using MDS in order to visualize a three-dimensional graph of the taxa in character space. Unfortunately, all of the analyses (except for the 57 character Xu et al. [2012] analysis) had high dimensions of minimum stress. This means that caution must be used in interpreting the patterns visible in the MDS.
Results
The BDC graph of Carr and Williamson (2010) shows a block of positive correlation in the lower left corner of Fig. 3 consisting entirely of members of the family Tyrannosauridae and Bistahieversor. Most taxa in this block share significant positive correlation with one another. Notably, however, Tyrannosaurus rex only shares significant positive correlation with Tyrannosaurus (Tarbosaurus) bataar, and although it is positively correlated with the MOR 590 Daspletosaurus sp., other Daspletosaurus spp., Albertosaurus sarcophagus, and Albertosaurus (Gorgosaurus) libratus, these pairings do not have significant bootstrap values. Additionally, most of the taxa share negative correlation with the other block of positively correlated taxa. The block of positive correlation in the upper right corner of the BDC graph is made up of outgroup taxa, as well as the tyrannosauroids Dilong and Guanlong. This block appears to be made of two separate blocks of positive correlation joined at the taxon Coelophysis. Dilong shares positive correlation with Guanlong and all outgroup taxa and negative correlation with all other taxa, but none of these pairings have significant bootstrap values. Guanlong shares positive correlation with Monolophosaurus, Allosaurus, Coelophysis, and Dilong, but only its pairing with Monolophosaurus has a significant bootstrap value. Guanlong shares negative correlation with T. rex, T. bataar, both Daspletosaurus entries, and A. sarcophagus. Of these, its pairings with T. bataar and the Daspletosaurus entries have significant bootstrap values.
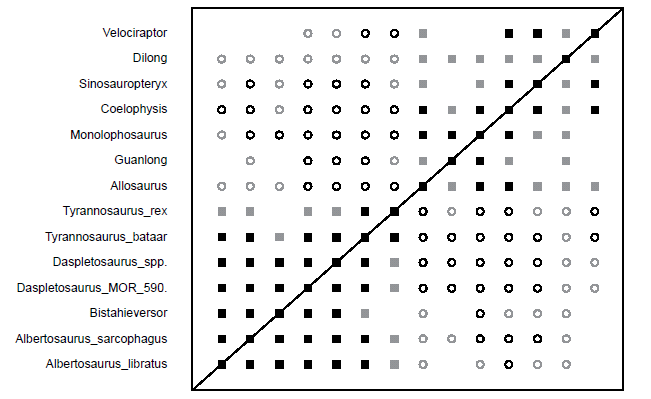
Fig. 3. Baraminic distance correlation (BDC) graph of the analysis of the Carr and Williamson (2010) dataset. Filled squares represent positive correlation, whereas open circles indicate negative correlation. Black symbols indicate bootstrap values ≥ 90%, and gray symbols indicate bootstrap values < 90%.
The 3D MDS graph for the Carr and Williamson (2010) dataset (Fig. 4) shows two major clusters with a clear separation between them. The first cluster is Y-shaped and consists solely of tyrannosaurids with Tyrannosaurus rex at the base of the Y, with Daspletosaurus forming one branch while the Albertosaurinae + Bistahieversor form the other. The second cluster consists of the outgroup taxa, Dilong, and Guanlong. The 3D stress for this analysis is rather poor at 0.175, with a minimum stress of 0.031 for MDS at eight dimensions.
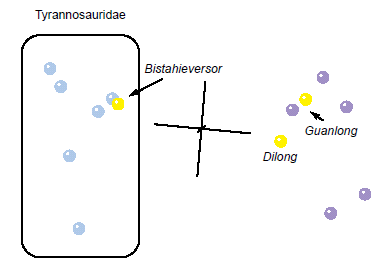
Fig. 4. Multidimensional scaling (MDS) results of the analysis of the Carr and Williamson (2010) dataset. Tyrannosaurid taxa are shown in blue, non-tyrannosaurid tyrannosauroid taxa are shown in yellow, and outgroup taxa are shown in purple.
BDC results for the Xu et al. (2012) dataset (Fig. 5) showed two major blocks of significant positive correlation separated by significant negative correlation. The lower block consists entirely of members of the Tyrannosauridae and Bistahieversor, whereas the upper block contains the outgroup taxa, as well as the tyrannosauroids Dilong, Guanlong, and Proceratosaurus. Eotyrannus shows positive correlation with the outgroup taxa, Dilong, Guanlong, and Proceratosaurus; however, Dilong is the only taxon in this block which shares significant positive correlation with Eotyrannus. The strength of the bootstrap values of the positive correlations between Eotyrannus and the other taxa were low to moderate (58–82%). Eotyrannus shares negative correlation with some of the tyrannosaurids (Tyrannosaurus, Tarbosaurus, Albertosaurus, and Gorgosaurus), and the bootstrap values for these correlations were low to moderate (45–83%). Eotyrannus is positively correlated with Xiongguanlong (bootstrap value of 79%) but shares neither positive nor negative correlation with Raptorex, even though Raptorex shares significant positive correlation with Xiongguanlong. Raptorex also shares positive correlation with three tyrannosaurid taxa (Alioramus, Gorgosaurus, and Albertosaurus) and Bistahieversor, but only its correlation with Bistahieversor has a significant bootstrap value, with the others all possessing bootstrap values over 82%. Yutyrannus does not share positive or negative correlation with any other taxon in the analysis.
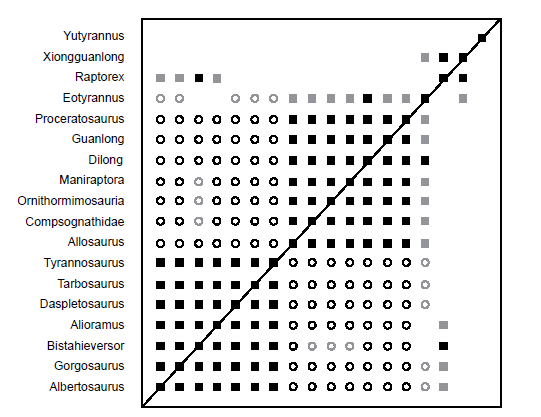
Fig. 5. BDC results of the analysis of the Xu et al. (2012) dataset. Filled squares represent positive correlation, whereas open circles indicate negative correlation. Black symbols indicate bootstrap values ≥ 90%, and gray symbols indicate bootstrap values < 90%.
The 3D MDS results for the Xu et al. (2012) dataset show two major clusters separated by a gap in character space (Fig. 6). The Tyrannosauridae form a definite linear pattern with Tarbosaurus and Tyrannosaurus at one end and the Albertosaurinae and Alioramus at the other end. Bistahieversor, Raptorex, and Xiongguanlong continue this trajectory toward the other cluster. A gap in character space separates Xiongguanlong from Eotyrannus, which does not seem to follow the trajectory. There is a smaller gap between Eotyrannus and the cluster consisting of the outgroup taxa, Dilong, Guanlong, and Proceratosaurus. Yutyrannus stands as a single point far away from all other taxa. The stress of the MDS at three dimensions is 0.105, with the minimum stress at 0.0489 in eight dimensions.
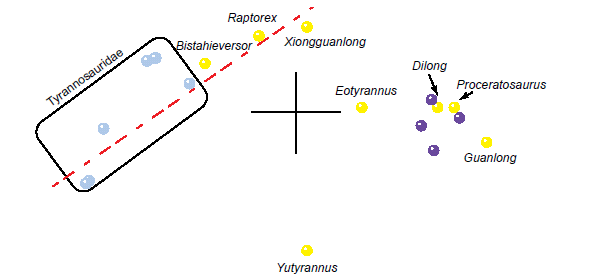
Fig. 6. MDS results of the analysis of the Xu et al. (2012) dataset. Tyrannosaurid taxa are shown in blue, non-tyrannosaurid tyrannosauroid taxa are shown in yellow, and outgroup taxa are shown in purple.
The BDC results from Lü et al. (2014) (Fig. 7) are practically identical to those of Xu et al. (2012). This is not surprising considering that both analyses are based on almost identical datasets. Xu et al. (2012) is based on Brusatte et al. (2010b), to which Brusatte and Benson (2013) added more characters. Lü et al. (2014) added even more characters as well as the genus Qianzhousaurus. Qianzhousaurus shares significant positive correlation with the rest of the Tyrannosauridae and Bistahieversor, and it shares significant negative correlation with the outgroup taxa, Dilong, Guanlong, and Proceratosaurus. Raptorex and Qianzhousaurus share positive correlation with a bootstrap value of 60%.
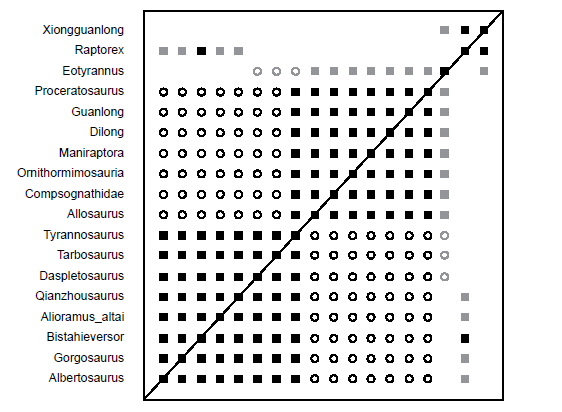
Fig. 7. BDC results of the analysis of the Lü et al. (2014) dataset. Filled squares represent positive correlation, whereas open circles indicate negative correlation. Black symbols indicate bootstrap values ≥ 90%, and gray symbols indicate bootstrap values < 90%.
The 3D MDS graph of the Lü et al. (2014) dataset (Fig. 8a) is similar in pattern to the 3D MDS of Xu et al. (2012). The Tyrannosauridae cluster is more curved than in the MDS of Xu et al. (2012), but the taxa are in the same order. The gap between Xiongguanlong and Eotyrannus appears smaller, and it is almost equal to the gap between Eotyrannus and Dilong. Although Eotyrannus does not appear to be on the trajectory of the Tyrannosauridae + Bistahieversor + Raptorex + Xiongguanlong cluster, from another angle (Fig. 8b) it can be seen that it is at the end of what appears to be a nearly linear trajectory, and that the Eotyrannus to Tyrannosaurus trajectory appears to run parallel to a more tightly clustered outgroup + Guanlong + Proceratosaurus trajectory. Dilong falls in between the two trajectories, but it is closer to the outgroup and proceratosaurid cluster. The stress of the MDS in three dimensions is 0.105, with the minimum stress at 0.039 in six dimensions.
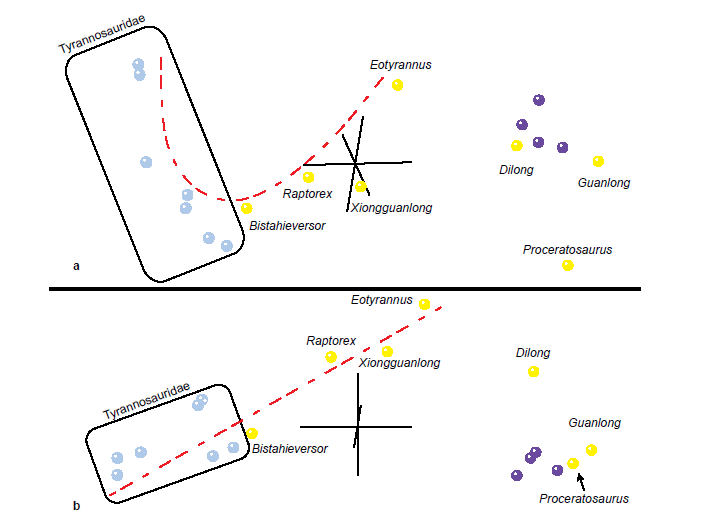
Fig. 8. MDS results of the analysis of the Lü et al. (2014) dataset. Tyrannosaurid taxa are shown in blue, non-tyrannosaurid tyrannosauroid taxa are shown in yellow, and outgroup taxa are shown in purple. The same MDS results are shown from two different viewing angles (a and b).
The BDC results from the analysis of Loewen et al. (2013) show two major blocks of significant positive correlation (Fig. 9). All of the taxa and specimens in the lower block are within the family Tyrannosauridae (except Bistahieversor, although Loewen et al. [2013] recovered Bistahieversor as a derived tyrannosaurid in their phylogenetic analysis) and show significant positive correlation with each other. Additionally, all tyrannosaurids and Bistahieversor show significant negative correlation with all taxa in the second block with only a few exceptions. The upper block consists of the outgroup taxa, Dilong, and Guanlong. Each taxon within this block shares significant positive correlation with every other taxon with a few exceptions. Notably, Dilong has bootstrap values of shared positive correlation between it and the dromaeosaurid taxa (Deinonychus, Linheraptor, and Velociraptor), Archaeopteryx, Haplocheirus, Scipionyx, Juravenator, and Harpymimus of 60–87%. Dilong shares neither positive nor negative correlation with Alioramus, Qianzhousaurus, and Gallimimus. Xiongguanlong and Raptorex, two tyrannosauroids not within Tyrannosauridae, share significant positive correlation with each other and with both species of Alioramus. Raptorex also shares significant positive correlation with Qianzhousaurus. Xiongguanlong shares positive correlation with Qianzhousaurus, but the bootstrap value is not significant (85%). Raptorex and Xiongguanlong share positive correlation with Dilong and Gorgosaurus, and Raptorex shares positive correlation with Albertosaurus, but none of these correlations has significant bootstrap values.
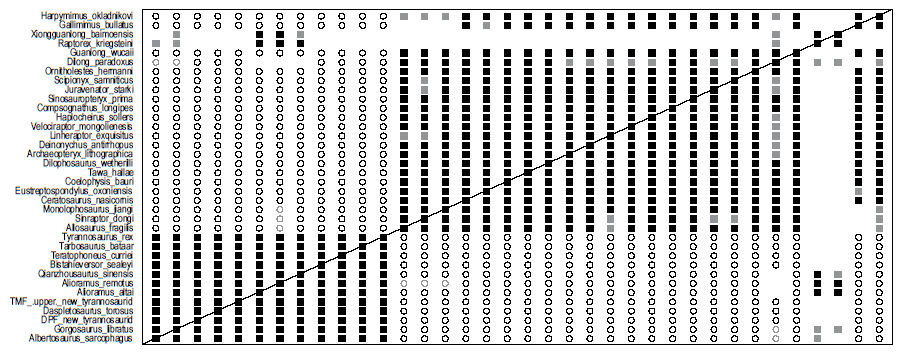
Fig. 9. BDC results of the analysis of the Loewen et al. (2013) (as modified by Lü et al. [2014]) dataset. Filled squares represent positive correlation, whereas open circles indicate negative correlation. Black symbols indicate bootstrap values ≥ 90%, and gray symbols indicate bootstrap values < 90%. Click image for larger display.
The 3D MDS graph of Loewen et al. (2013) shows four major clusters of taxa in character space (Fig. 10). The tyrannosaurid + Bistahieversor cluster on the left once again takes on a linear shape, with Tyrannosaurus and Tarbosaurus on the upper end and with Alioramus remotus on the bottom. The two taxa in between the tyrannosaurid trajectory and the large cluster of outgroup taxa, Guanlong, and Dilong are Raptorex and Xiongguanlong. Unlike previous MDS results, these two taxa are not aligned with the tyrannosaurid trajectory. The two taxa above the large outgroup, Guanlong, and Dilong cluster are the ornithomimosaurs Harpymimus and Gallimimus. The stress in three dimensions for these MDS results was poor at 0.192. The minimum stress was 0.032 in 13 dimensions.
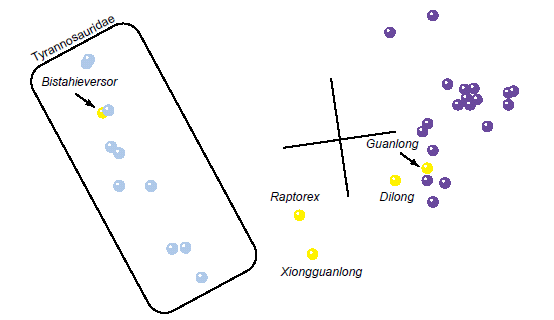
Fig. 10. MDS results of the analysis of the Loewen et al. (2013) (as modified by Lü et al. [2014]) dataset. Tyrannosaurid taxa are shown in blue, non-tyrannosaurid tyrannosauroid taxa are shown in yellow, and outgroup taxa are shown in purple.
Fig. 11 shows the BDC for the 57-character analysis of Xu et al. (2012), which was performed for the purpose of including as many of the poorly known tyrannosauroid taxa as possible. Two clear blocks of positive correlation are evident in Fig. 11. The lower block contains the Tyrannosauridae, Bistahieversor, Appalachiosaurus, Dryptosaurus, Raptorex, and Xiongguanlong. The shared positive correlations between the tyrannosaurid taxa all have significant bootstrap values with a few exceptions mainly involving Teratophoneus. Additionally, Tyrannosaurus and Tarbosaurus do not share positive or negative correlation with Teratophoneus. Appalachiosaurus shares positive correlation with all tyrannosaurid taxa, and the bootstrap values of these correlations are all significant except with Daspletosaurus, Tarbosaurus, and Tyrannosaurus. Dryptosaurus positively correlates with every taxon in the lower block, and all but four of its correlations have significant bootstrap values. Raptorex shares positive correlation with all tyrannosaurid taxa and Appalachiosaurus, and most of the bootstrap values for these correlations are significant. Only when Raptorex is compared with Teratophoneus, Daspletosaurus, Tarbosaurus, and Tyrannosaurus are the bootstrap values not significant. Xiongguanlong only positively correlates with Raptorex and Dryptosaurus within the lower block of positive correlation. Its positive correlation with Raptorex has a significant bootstrap value, but this is not the case for its positive correlation with Dryptosaurus. Interestingly, Xiongguanlong also shares significant positive correlation with the tyrannosauroid Eotyrannus. Other than these three pairings, Xiongguanlong does not show positive or negative correlation with any other taxon in the analysis. Eotyrannus does share positive correlation with the following non-tyrannosaurid taxa: Dilong, Maniraptora, Ornithomimosauria, and Allosaurus; however, none of these pairings have significant bootstrap values (41–63%). All tyrannosaurid taxa and Bistahieversor show negative correlation with all outgroup taxa, the Proceratosauridae (Kileskus, Proceratosaurus, Sinotyrannus, and Guanlong), and Dilong with the only exceptions being some pairings including Teratophoneus and Bistahieversor. The majority of these negative correlations do not have significant bootstrap values, but many do, especially when comparing Daspletosaurus, Tarbosaurus, and Tyrannosaurus to the upper block. Among the non-tyrannosaurid tyrannosauroids that are in the lower block, Appalachiosaurus shows negative correlation with all taxa in the upper block except Maniraptora, Dryptosaurus shows negative correlation with all taxa in the upper block except Maniraptora and Sinotyrannus, and Raptorex shows negative correlation with all taxa in the upper block except Ornithomimosauria, Maniraptora, and Sinotyrannus. Tyrannosaurus and Tarbosaurus share negative correlation with Eotyrannus, but the bootstrap values are very poor (36% and 35%, respectively). Yutyrannus does share positive correlation with the Proceratosauridae, and it shares negative correlation with Appalachiosaurus, Raptorex, and all tyrannosaurid taxa except Teratophoneus and Alioramus. None of the correlations Yutyrannus shares with any other taxa exhibit significant bootstrap values.
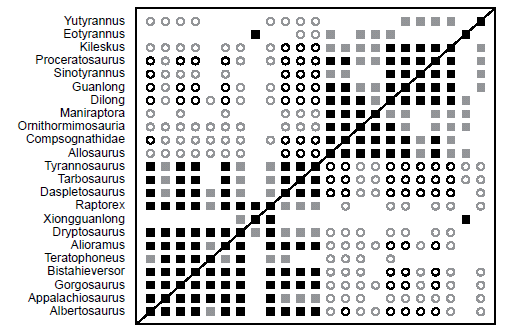
Fig. 11. BDC results of the second analysis of the Xu et al. (2012) dataset, which was conducted for the purpose of including as many taxa as possible. Filled squares represent positive correlation, whereas open circles indicate negative correlation. Black symbols indicate bootstrap values ≥ 90%, and gray symbols indicate bootstrap values < 90%.
The 3D MDS results of the 57-character Xu et al. (2012) analysis (Fig. 12) show two large clusters of taxa, echoing the BDC of the same dataset. The cluster on the left in Fig. 12 is made up of tyrannosaurids, Bistahieversor, Dryptosaurus, Appalachiosaurus, and Raptorex. Teratophoneus, a tyrannosaurid, groups away from the main tyrannosaurid cluster, which is unusual. This is most likely due to the small number of characters used and to the previous scarcity of Teratophoneus remains, as evidenced by its placement well within the tyrannosaurid cluster in the MDS results of the analysis of Loewen et al. (2013). This cloud of taxa shows a somewhat curvilinear trajectory, but it is not as clearly defined as it is in the other analyses. Xiongguanlong and Eotyrannus seem to continue the trajectory leading to the outgroup taxa. Interestingly, the taxa in the non-tyrannosaurid cluster that are closest to Eotyrannus are not tyrannosauroids, but rather Maniraptora, Ornithomimosauria, and Allosaurus. The stress at three dimensions for the MDS is 0.155 with the dimension of minimum stress being the fourth dimension at 0.154.
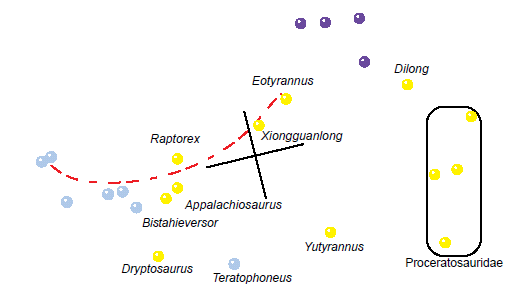
Fig. 12. MDS results of the second analysis of the Xu et al. (2012) dataset, which was conducted for the purpose of including as many taxa as possible. Tyrannosaurid taxa are shown in blue, non-tyrannosaurid tyrannosauroid taxa are shown in yellow, and outgroup taxa are shown in purple.
The final analysis is a subset of the Xu et al. (2012) dataset, and it consists only of non-tyrannosaurid tyrannosauroid taxa and two tyrannosaurids: Gorgosaurus and Albertosaurus. The BDC results (Fig. 13) show two blocks of positive correlation, one consisting of the two tyrannosaurids, Bistahieversor, Appalachiosaurus, Dryptosaurus, Xiongguanlong, Raptorex, and Eotyrannus, whereas the other contains the Proceratosauridae (Proceratosaurus, Kileskus, Sinotyrannus, and Guanlong), Dilong, and Yutyrannus. These two blocks do not share any positive correlation with one another, and all taxa share negative correlation with every taxa in the opposite block except for Eotyrannus, which shares no negative correlation with any taxa. Eotyrannus only shares positive correlation with Xiongguanlong, and this pairing has a non-significant bootstrap value (63%). The shared correlation pairings between most taxa in the lower block have significant bootstrap values, although the pairings between Xiongguanlong and the two tyrannosaurid taxa are not significant. In the upper block of positive correlation, Dilong does not have positive or negative correlation with Yutyrannus, and all of its pairings with other taxa do not have significant bootstrap values. The four proceratosaurids all share significant positive correlation, except for Sinotyrannus which only shares significant positive correlation with Guanlong. Like Dilong and Eotyrannus, none of the correlations between Yutyrannus and any other taxa have significant bootstrap values.
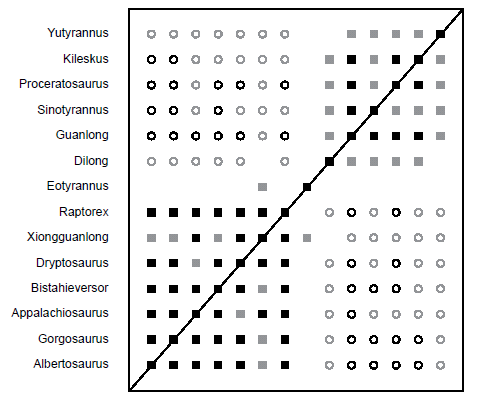
Fig. 13. BDC results of the subset analysis of the Xu et al. (2012) dataset, containing only two tyrannosaurid taxa and non-tyrannosaurid tyrannosauroid taxa. Filled squares represent positive correlation, whereas open circles indicate negative correlation. Black symbols indicate bootstrap values ≥ 90%, and gray symbols indicate bootstrap values < 90%.
The MDS results for this subset of the Xu et al. (2012) dataset (Fig. 14) show two clusters of taxa separated by a noticeable gap in character space, which is in agreement with the BDC results. The two tyrannosaurid taxa along with Bistahieversor and Raptorex cluster closely with Appalachiosaurus nearby but slightly off of the path. Farther away from this cluster is Xiongguanlong, with Eotyrannus beyond that, such that Eotyrannus, Xiongguanlong, and Appalachiosaurus seem to form a straight line in multidimensional character space. Stress in three dimensions was very poor at 0.245, and the minimum stress of 0.243 was at four dimensions.
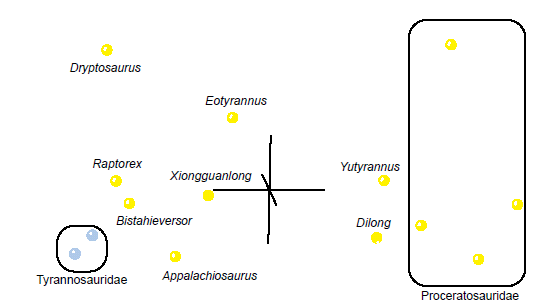
Fig. 14. MDS results of the subset analysis of the Xu et al. (2012) dataset, containing only two tyrannosaurid taxa and non-tyrannosaurid tyrannosauroid taxa. Tyrannosaurid taxa are shown in blue and non-tyrannosaurid tyrannosauroid taxa are shown in yellow.
Discussion
The BDC results for all of the analyses show that there is strong positive correlation within the family Tyrannosauridae. Additionally, the BDC results from the first five analyses show strong negative correlation between Tyrannosauridae and all outgroup taxa. The MDS results agree with the BDC on this point, showing a clumped tyrannosaurid cluster far off from the outgroup taxa. These results suggest that there is strong continuity within the family Tyrannosauridae and strong discontinuity between tyrannosaurids and non-tyrannosauroid theropods. Carr and Williamson (2010) had considered Bistahieversor to belong outside the family Tyrannosauridae, but Loewen et al (2013) recovered it in their phylogenetic analysis as a derived tyrannosaurid. Bistahieversor, based on the results of every one of these analyses, should be considered to be continuous with this group and discontinuous with the outgroup taxa.
However, the other tyrannosauroid taxa complicate matters. In the BDC results of Xu et al. (2012), Lü et al. (2014), and Loewen et al. (2013), Raptorex always shares positive correlation with members of the Tyrannosauridae, which suggests continuity. However, in none of those three BDC analyses does Raptorex share negative correlation with any taxa. It is not until the two additional analyses of Xu et al. (2012) that Raptorex shows negative correlation with some of the outgroup taxa, the Proceratosauridae, Dilong, and Yutyrannus. These results suggest that Raptorex is continuous with the tyrannosaurids and Bistahieversor, and that this group together is discontinuous from the outgroup taxa.
Xiongguanlong shares positive correlation with Raptorex in the BDC results of Loewen et al. (2013), Lü et al. (2014), and Xu et al. (2012), as well as in the two additional BDC analyses of Xu et al. (2012). Therefore, Xiongguanlong should be considered continuous with Raptorex. However, Xiongguanlong almost never shares positive correlation with any of the tyrannosaurid taxa. It is only in the BDC results of Loewen et al. (2013) and the subset of Xu et al. (2012) that Xiongguanlong shows positive correlation with any tyrannosaurid taxa. These results suggest that Xiongguanlong is continuous with this group.
Interestingly, Xiongguanlong also shares positive correlation with Eotyrannus in the BDC results of Lü et al. (2014), Xu et al. (2012), and both additional analyses of Xu et al. (2012). Eotyrannus shares positive correlation with the outgroup taxa, the proceratosaurids, and Dilong in Lü et al. (2014) and Xu et al. (2012), and it shows positive correlation with Maniraptora, Ornithomimosauria, Allosaurus, and Dilong in the Xu et al. (2012) small character full analysis. However, Eotyrannus does not show any positive or negative correlation with any taxon other than Xiongguanlong in the Xu et al. (2012) subset analysis. The results of these analyses tentatively suggest that Eotyrannus may be continuous with Xiongguanlong, and the BDC result of the subset analysis do not confirm a relationship between Eotyrannus and the outgroup taxa, Proceratosauridae, Dilong, Sinotyrannus, or Yutyrannus. MDS results of the subset analysis suggest that Eotyrannus is discontinuous from Dilong, Yutyrannus, and Proceratosauridae (although the stress for the MDS was very poor for this analysis).
If Eotyrannus is truly continuous with Xiongguanlong, and Xiongguanlong is continuous with Raptorex, Bistahieversor, and Tyrannosauridae, then why do Eotyrannus and Xiongguanlong not show positive correlation with tyrannosaurid taxa in most BDC analyses? The answer is revealed in the MDS results. In every MDS graph, the Tyrannosauridae + Bistahieversor cluster takes on a linear or curvilinear shape. This trajectory always has the tyrannosaurines Tyrannosaurus and Tarbosaurus at one end (although the MDS of Carr and Williamson [2010] places a gap in character space between the two taxa which is absent in other analyses), and the tyrannosaurid constituents from the other end vary between the alioramins (Loewen et al., 2013) and Bistahieversor + Albertosaurinae (Carr and Williamson 2010; Lü et al., 2014; all Xu et al. 2012 analyses). Even though these taxa are the last tyrannosaurids in the trajectory, the trajectory continues with Appalachiosaurus in the additional Xu et al. (2012) analysis including as many taxa as possible. Raptorex is next in all three MDS results for Xu et al. (2012) and in Lü et al. (2014), followed by Xiongguanlong in those same MDS graphs (although its placement is odd in the subset analysis MDS), and then by Eotyrannus in those MDS results except for the original MDS of Xu et al. (2012) where it seems to cluster with the very distant tyrannosauroids. The reason that Xiongguanlong and Eotyrannus do not typically show positive correlation with the tyrannosaurid taxa is because they are far removed from them in character space. Nevertheless, there is a clear trajectory in every one of these MDS graphs, although a few taxa are sometimes out of place from analysis to analysis. A similar linear pattern of taxa in morphological character space occurred when Cavanaugh, Wood, and Wise (2003) analyzed the fossil equids. In the BDC results, they found that there was a chain of positive correlation from Hyracotherium to Equus but that the Hyracotheriinae and other early taxa showed significant negative correlation with the Equinae. When they viewed the taxa in three-dimensional character space using ANOPA, they found the taxa formed a linear trajectory from Hyracotherium to Equus with one side branch near the top (Anchitheriinae). Thus, it is clear why Hyracotherium and similar taxa would show significant negative correlation with the Equinae since they are far away from each other in biological character space. Nevertheless, the taxa are connected by significant positive correlation each step up the trajectory. The order of the taxa within the trajectory correctly matched the stratigraphic placement of their fossils. The authors concluded that all members were in the same monobaramin, and that this was a true post-Flood stratomorphic series.
It would appear that a similar situation exists for these tyrannosauroid taxa. A chain of positive correlation seems to connect Xiongguanlong, Raptorex, Appalachiosaurus, Bistahieversor, and Tyrannosauridae. Within Tyrannosauridae, the Alioramini and Albertosaurinae are located on one end whereas the Tyrannosaurinae are on the other, farther away from the non-tyrannosaurid tyrannosauroid taxa. This is not surprising when one considers the body plan of tyrannosaurids: Alioramins and albertosaurines are much more gracile than tyrannosaurines, whereas tyrannosaurines have stronger and larger skulls, wider jaws, and proportionately smaller arms than either alioramins or albertosaurines. Thus, it would seem that they take the tyrannosaurid body plan to the extreme. Based on these results, I tentatively suggest that this trajectory reflects pre-Flood diversification that has been preserved in Flood rocks.
If I am correct that Eotyrannus is continuous with Xiongguanlong but not with Dilong or Proceratosauridae, then it is possible that Eotyrannus is the first taxon of this linear cluster. Unfortunately, Eotyrannus is only known from one fragmentary skeleton from the Isle of Wight, England (Hutt et al. 2001). A new description of the Eotyrannus material is underway (Naish 2014), and this may bring some answers as to its placement relative to the Tyrannosauridae. Eotyrannus does share several features in common with Tyrannosauridae including a mediolaterally oriented premaxillary tooth row, D-shaped premaxillary teeth in cross section, and fused nasals (Hutt et al. 2001). Based on the BDC and MDS results of the subset analysis of Xu et al. (2012), I tentatively consider Eotyrannus to be continuous with Tyrannosauridae + Bistahieversor + Appalachiosaurus + Raptorex + Xiongguanlong and discontinuous from more distant tyrannosauroids and outgroup taxa. Unlike tyrannosaurids, Eotyrannus apparently had rather long arms and hands in proportion to its body with three functional digits (Hutt et al. 2001). Unfortunately, the forelimbs of Xiongguanlong are unknown (Li et al. 2010), but Raptorex possesses puny arms with two-fingered hands like a tyrannosaurid (Sereno et al. 2009). It should be noted, however, that according to Fowler et al. (2011), Raptorex may be a juvenile Tarbosaurus. The fossil was obtained through a sale, and its location of origin was unknown. Sereno et al. (2009) suspected it was from the Yixian Formation of China, which would put Raptorex in the Lower Cretaceous. Fowler et al. (2011) provided arguments in favor of an Upper Cretaceous, Mongolian origin for the fossil, putting it in the same beds as the tyrannosaurid Tarbosaurus. Since the fossil is a juvenile or subadult, Fowler et al. (2011) concluded that it is probably a juvenile Tarbosaurus. Thus, at this point Raptorex should not be seriously considered as an example of the appearance of a non-tyrannosaurid tyrannosauroid. The forelimbs of Appalachiosaurus are also unknown (Carr, Williamson, and Schwimmer 2005; Jovanelly and Lane 2012).
Since the Mesozoic sediments are thought to have been laid down in Noah’s Flood (Austin et al. 1994; Brand 2009; Snelling 2009), one would not expect a stratomorphic series to appear in Mesozoic rocks. Fig. 15 shows the trajectory from the MDS analysis of the Lü et al. (2014) dataset, but with the stratigraphic stage of each taxon indicated. Overall, the morphological trajectory does in fact correlate with the general stratigraphy, which is unexpected for fossil taxa in Flood rocks. However, upon closer inspection, the pattern falls apart within the Tyrannosauridae. Daspletosaurus is always between the Tyrannosaurus and Tarbosaurus points on the one side and the Albertosaurinae and Alioramini on the other in every MDS result from every analysis in which the taxa were included (except for its position on a branch of the Y-shaped cluster in the Carr and Williamson [2010] analysis), yet it is stratigraphically below Albertosaurus, Qianzhousaurus, and Alioramus. Raptorex might be from the Lower Cretaceous, or it may be a juvenile Tarbosaurus from the Upper Cretaceous. If the latter is the case, then this suggests that a juvenile tyrannosaurid phenetically resembles non-tyrannosaurid tyrannosauroids, which may be similar to tyrannosaurid ancestors. This is interesting, but until the debate on the identity of Raptorex is settled, no more observations on this relationship will be possible.
The monobaraminic status of the family Tyrannosauridae is confirmed by continuity interpreted from the BDC and MDS results of this study. The restricted stratigraphic and geographic ranges of the tyrannosaurids and the shared body plan also suggest a monobaraminic status. I suggest, based on the BDC and MDS results from this study, that the monobaramin should be extended to include the tyrannosauroids Bistahieversor, Appalachiosaurus, Dryptosaurus, Raptorex, Xiongguanlong, and possibly Eotyrannus. Dryptosaurus is known from very poor remains, and it is possible with the discovery of more fossils that it will be excluded. Additionally, Eotyrannus is not well-known, and it may not be continuous with this group. Nevertheless, the linear/curvilinear trajectory seen in some of the MDS results stretching from Eotyrannus to Tyrannosaurus suggests that this is a single monobaramin, and that it resembles a biological trajectory. However, it is important to note that all of these taxa lived at the same general time since they were killed by the same event. Nevertheless, it is possible that Eotyrannus might be more similar to an ancestral tyrannosaur than tyrannosaurids and that tyrannosaurids might have changed in the pre-Flood world to take on the role of a large predator, which would make them look more “derived” than Eotyrannus.
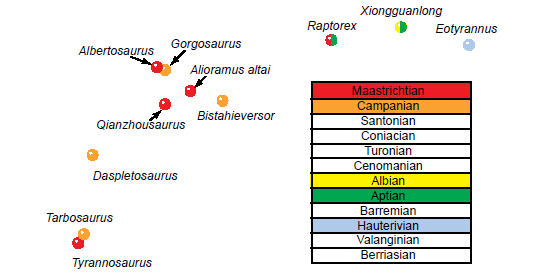
Fig. 15. Tyrannosaurid and non-tyrannosaurid tyrannosauroid taxa that formed a curvilinear cluster in the MDS results of the Lü et al. (2014) dataset. Taxa are colored according to their stage of the Cretaceous. Xiongguanlong is yellow and green because it cannot be placed more precisely than Aptian-Albian. Raptorex may be a non-tyrannosaurid tyrannosauroid from the Aptian, or it may be a juvenile Tarbosaurus from the Maastrichtian. Stages for taxa obtained from Fiorillo and Tykoski (2014) and Fowler et al. (2011).
I tentatively suggest, based on the BDC and MDS results of the six analyses, that Tyrannosauridae + Bistahieversor + Appalachiosaurus + Dryptosaurus + Raptorex + Xiongguanlong + Eotyrannus is discontinuous with the other tyrannosauroids used in these analyses (Proceratosauridae and Dilong) and with outgroup theropods used in these analyses. Thus, I conclude that the group including Tyrannosauridae, Bistahieversor, Appalachiosaurus, Dryptosaurus, Raptorex, Xiongguanlong, and Eotyrannus is a holobaramin. These results agree with and expand upon those obtained by Wood, Ross, and Garner (2011), who recovered Tyrannosauroidea as an apobaramin; however, their analysis only contained tyrannosaurid taxa.
Further discoveries of Eotyrannus material or other tyrannosauroid fossils may alter these conclusions. Future analyses may show that Eotyrannus is in fact continuous with Dilong or other tyrannosauroids. This may result in the addition of Dilong and other tyrannosauroids into this holobaramin, or it may indicate that this is not a holobaramin, but that Tyrannosauroidea is simply a monobarmin within a larger holobaramin of theropods. Another possibility is that Eotyrannus will be determined to be discontinuous from Xiongguanlong in future analyses, thus putting it outside the tyrannosauroid holobaramin. A recent publication by Porfiri et al. (2014) has suggested that the megaraptorans, previously thought to group with the allosauroid Neovenator, are actually tyrannosauroids. They also concluded that Eotyrannus is a megaraptoran. Future analyses including these taxa will hopefully qualify the baraminological relationships of the Tyrannosauroidea.
If Eotyrannus is indeed a member of this holobaramin, then this suggests that some interesting changes have occurred within this tyrannosauroid holobaramin. For instance, Eotyrannus had long, grasping hands with three manual digits. This is very different than the tyrannosaurid condition of short two-fingered hands. If the morphological trajectory of this holobaramin reflects a true pathway of diversification, then this suggests that tyrannosaurids may be descended from ancestors that resembled Eotyrannus (although certainly Eotyrannus had changed since the initial creation of tyrannosaurs, so it cannot be called an ancestor any more than Tyrannosaurus could be). This would mean that, like horses, tyrannosaurs lost a digit (although horses lost more than one). Additionally, the enlargement of the skull relative to the body, the increasing robustness of the skull, and the enlargement of the teeth might be related to hypercarnivory of large animals. One might expect that the original created tyrannosaur would have no use for these features, but that they might come about after the Fall. I would suggest that the large, strong skulls full of enormous teeth are characteristics controlled by genes built into the tyrannosaur genome that did not express themselves until they were necessary for stable ecosystems in the pre-Flood world. I suspect that the original created tyrannosaur had longer, grasping arms and a much smaller skull in proportion to its body. Tyrannosaurids, even though they do not possess a third finger, do possess a third metacarpal, although it is greatly reduced in thickness compared to the digit-bearing metacarpals I and II (Holtz 2004). This is similar to the condition in horses which have thin splints of bone thought to be derived from metacarpal/metatarsal II and IV. Interestingly, Quinlan, Derstler, and Miller (2007) reported finding a Tyrannosaurus rex fossil with three fingers on each hand in the Hell Creek Formation of Montana. They noted that the phalanges of digit III and metacarpal III were fused into a single, slightly flexed unit. Larson (2013) reported that he observed a Nanotyrannus specimen (BHI-6437) possessing digit III on both hands (unfortunately, the specimen is currently not able to be studied and may never be if it is auctioned). This condition has not been found in other fossils of Tyrannosaurus rex, Nanotyrannus, or in other tyrannosaurids (Holtz 2004). Thus, the small arms of tyrannosaurids and the loss of digit III might accurately be called vestigial structures even within the creationist paradigm.
The definition of the Tyrannosauridae + Bistahieversor + Appalachiosaurus + Dryptosaurus + Raptorex + Xiongguanlong + Eotyrannus holobaramin imposes certain baraminological constraints on higher dinosaur clades. Notably, Dinosauria, Saurischia, and Theropoda—as they are traditionally understood—must be polybaraminic since the presence of the tyrannosauroid holobaramin means at least one other holobaramin exists within these groups. All three clades have been cladistically defined as including Aves, of which holobaramins and apobaramins have been recognized (Garner, Wood, and Ross 2013; Wood 2005a). Additionally, these clades contain land animals, which were created on Day 6 (Genesis 1:24–25), and flying animals, which were created on Day 5 (Genesis 1:20–21).
It is not the purpose of this paper to define whether discontinuity surrounds any of these three larger groups; nevertheless, I hypothesize that most likely Dinosauria, Saurischia, and Theropoda will be found to be apobaramins, as I suspect that further analysis will probably reveal a morphological gap separating them from other groups at their respective levels. These claims should be tested with further baraminological analyses.
Conclusion
After reviewing the BDC and MDS results from all five analyses, as well as relevant stratigraphic and paleobiogeographic data, I make the following conclusions: 1) Tyrannosauridae is a monobaramin; 2) Tyrannosauridae + Bistahieversor + Appalachiosaurus + Dryptosaurus + Raptorex + Xiongguanlong + Eotyrannus is holobaraminic with respect to more distant members of Tyrannosauroidea and all other dinosaurs; 3) The members of this new holobaramin form a trajectory in character space which may suggest a pattern for diversification in the pre-Flood world; 4) Tyrannosaurs may have originally had smaller skulls and larger arms in proportion to their bodies as well as three-fingered hands; 5) Dinosauria, Saurischia, and Theropoda are polybaraminic. It is possible that with further fossil discoveries, Eotyrannus may be found not to belong to this holobaramin, or intermediates may be found linking the more distant tyrannosauroids to the Tyrannosauridae. Thus, caution must be used with the results and implications of this analysis, as it should with all baraminological studies of fossil taxa. It is the hope of the author that this initial study of a dinosaur family through the use of statistical baraminology will be followed by similar analyses of other dinosaur taxa.
Acknowledgments
I would like to thank Todd Wood for his assistance in using the BDISTMDS software and in interpreting the results obtained. Thomas Clevenger helped in the initial analyses, and without his assistance this project would have taken considerably longer. I would also like to thank John Whitmore for his suggestions during these analyses. Several individuals, including Brandon Marini and E.A.M., helped in editing and revising the manuscript. Finally, I would like to thank the two anonymous reviewers for their suggestions which vastly improved the paper, and I would like to thank Andrew Snelling for his help throughout the entire review process.
References
Aaron, M. 2014. Baraminological analysis of the Caseidae (Synapsida: Pelycosauria). Journal of Creation Theology and Science Series B: Life Science 4: 19–22.
Austin, S. A., J. R. Baumgardner, D. R. Humphreys, A. A. Snelling, L. Vardiman, and K. P. Wise. 1994. Catastrophic plate tectonics: a global Flood model of earth history. In Proceedings of the Third International Conference on Creationism, ed. R. E. Walsh, 609–621. Pittsburgh, Pennsylvania: Creation Science Fellowship.
Brand, L. 2009. Faith, reason, & earth history. Andrews University Press: Berrien Springs, Michigan.
Brusatte, S. L., R. B. J. Benson, P. J. Currie, and X. Zhao. 2010a. The skull of Monolophosaurus jiangi (Dinosauria: Theropoda) and its implications for early theropod phylogeny and evolution. Zoological Journal of the Linnean Society 158, no. 3: 573–607.
Brusatte, S. L., M. A. Norell, T. D. Carr, G. M. Erickson, J. R. Hutchinson, A. M. Balanoff, G. S. Bever, J. N. Choiniere, P. J. Makovicky, and X. Xu. 2010b. Tyrannosaur paleobiology: New research on ancient exemplar organisms. Science 329, no. 5998: 1481–1485.
Brusatte, S. L., and R. B. J. Benson. 2013. The systematics of Late Jurassic tyrannosauroids (Dinosauria: Theropoda) from Europe and North America. Acta Palaeontologica Polonica 58, no. 1: 47–54.
Butler, R. J., P. Upchurch, and D. B. Norman. 2008. The phylogeny of the ornithischian dinosaurs. Journal of Systematic Paleontology 6, no. 1: 1–40.
Carr, T. 2006. Is Guanlong a tyrannosauroid or a subadult Monolophosaurus? Journal of Vertebrate Paleontology 26: 48A.
Carr, T., and T. E. Williamson. 2010. Bistahieversor sealeyi, gen. et sp. nov., a new tyrannosauroid from New Mexico and the origin of deep snouts in Tyrannosauroidea. Journal of Vertebrate Paleontology 30, no. 1: 1–16.
Carr, T., T. E. Williamson, and D. R. Schwimmer. 2005. A new genus and species of tyrannosauroid from the Late Cretaceous (middle Campanian) Demopolis Formation of Alabama. Journal of Vertebrate Paleontology 25, no. 1: 119–143.
Cavanaugh, D. 2011. An ANOPA study of coelurosaurian theropods, CBS Annual Conference Abstracts. Journal of Creation Theology and Science Series B: Life Sciences 1: 18.
Cavanaugh, D. P., T. C. Wood, and K. P. Wise. 2003. Fossil equidae: A monobaraminic, stratomorphic series. In Proceedings of the Fifth International Conference on Creationism, ed. R. L. Ivey Jr., 143–153. Pittsburgh, Pennsylvania: Creation Science Fellowship.
Currie, P. J. 2003. Cranial anatomy of tyrannosaurid dinosaurs from the Late Cretaceous of Alberta, Canada. Acta Palaeontologica Polonica 48, no. 2: 191–226.
Currie, P. J., J. H. Hurum, and K. Sabath. 2003. Skull structure and evolution in tyrannosaurid dinosaurs. Acta Palaeontologica Polonica 48, no. 2: 227–234.
Fiorillo, A. R., and R. S. Tykoski. 2014. A diminutive new tyrannosaur from the top of the world. PLoS ONE 9, no. 3: e91287. doi:10.1371/journal.pone.0091287.
Fowler, D. W., H. N. Woodward, E. A. Freedman, P. L. Larson, and J. R. Horner. 2011. Reanalysis of “Raptorex kriegsteini”: A juvenile tyrannosaurid dinosaur from Mongolia. PLoS ONE 6, no. 6: e21376. doi:10.1371/journal.pone.0021376.
Garner, P., T. Wood, and M. Ross. 2013. Baraminological analysis of Jurassic and Cretaceous Avialae. In Proceedings of the Seventh International Conference on Creationism, ed. M. Horstemeyer. Pittsburgh, Pennsylvania: Creation Science Fellowship.
Holtz, T. R. Jr. 2001. The phylogeny and taxonomy of the Tyrannosauridae. In Mesozoic vertebrate life, ed. D. H. Tanke and K. Carpenter, 64–83. Bloomington, Indiana: Indiana University Press.
Holtz, T. R. Jr. 2004. Tyrannosauroidea. In The Dinosauria, ed. D. B. Weishampel, P. Dodson, and H. Osmólska, 111–136. Berkeley, California: University of California Press.
Holtz, T. R., Jr. and H. Osmólska. 2004. Saurischia. In The Dinosauria, ed. D. B. Weishampel, P. Dodson, and H. Osmólska, 21–24. Berkeley, California: University of California Press.
Hutt, S., D. Naish, D. M. Martill, M. J. Barker, and P. Newberry. 2001. A preliminary account of a new tyrannosauroid theropod from the Wessex Formation (Early Cretaceous) of southern England. Cretaceous Research 22, no. 2: 227–242.
Jovanelly, T. J., and L. Lane. 2012. Comparison of the functional morphology of Appalachiosaurus and Albertosaurus. The Open Geology Journal 6: 65–71.
Larson, P. 2013. The validity of Nanotyrannus lancensis (Theropoda, Lancian—Upper Maastrichtian of North America). Society of Vertebrate Paleonotology 73rd Annual Meeting Program and Abstracts, 159.
Li, D., M. A. Norell, K.-Q. Gao, N. D. Smith, and P. J. Makovicky. 2010. A longirostrine tyrannosauroid from the Early Cretaceous of China. Proceedings of the Royal Society of Biology 277, no. 1679: 183–190.
Loewen, M. A., R. B. Irmis, J. J. W. Sertich, P. J. Currie, and S. D. Sampson. 2013. Tyrant dinosaur evolution tracks the rise and fall of Late Cretaceous oceans. PLoS ONE 8, no. 11: e79420. doi:10.1371/journal.pone.0079420.
Lü, J., L. Yi, S. L. Brusatte, L. Yang, H. Li, and L. Chen. 2014. A new clade of Asian Late Cretaceous long-snouted tyrannosaurids. Nature Communications 5, no. 3788. doi: 10.1038/ncomms4788.
Mace, S. R., and T. C. Wood. 2005. Statistical evidence for five whale holobaramins (Mammalia: Cetacea). Occasional Papers of the Baraminology Study Group 5: R10.
McConnachie, M., and T. R. Brophy. 2008. A baraminological analysis of the landfowl (Aves: Galliformes). Occasional Papers of the BSG 11: 9–10.
Naish, D. 2014. Ostrich dinosaurs invade Europe! Or do they? Tetrapod Zoology. Accessed August 19, 2014. http:// blogs.scientificamerican.com/tetrapod-zoology/2014/06/03/ostrich-dinosaurs-invade-europe-or-do-they/.
Paul, G. S. 1989. Predatory dinosaurs of the world. New York, New York: Simon and Schuster.
Porfiri, J. D. F. E. Novas, J. O. Calvo, F. L. Agnolin, M. D. Ezcurra, and I. A. Cerda. 2014. Juvenile specimen of Megaraptor (Dinosauria, Theropoda) sheds light about tyrannosauroid radiation. Cretaceous Research 51: 35–55.
Quinlan, E. D., K. Derstler, and M. M. Miller. 2007. Anatomy and function of digit III of the Tyrannosaurus rex manus. Geological Society of America Abstracts with Programs 39, no. 6: 77.
Robinson, D. A., and D. P. Cavanaugh. 1998. A quantitative approach to baraminology with examples from Catarrhine primates. Creation Research Society Quarterly 34, no. 4: 196–208.
Senter, P. 2010. Using creation science to demonstrate evolution: application of a creationist method for visualizing gaps in the fossil record to a phylogenetic study of coelurosaurian dinosaurs. Journal of Evolutionary Biology 23, no. 8: 1732–1743.
Sereno, P. C., L. Tan, S. L. Brusatte, H. J. Kriegstein, X. Zhao, and K. Cloward. 2009. Tyrannosaurid skeletal design first evolved at small body size. Science 326, no. 5951: 418–422.
Snelling, A. A. 2009. Earth’s catastrophic past: Geology, Creation, and the Flood. Dallas, Texas: Institute for Creation Research.
Wood, T. C. 2005a. A creationist review and preliminary analysis of the history, geology, climate, and biology of the Galápagos Islands. Center for Origins Research Issues in Creation 1.
Wood, T. C. 2005b. Visualizing baraminic distances using classical multidimensional scaling. Origins (GRI) 57: 9–29.
Wood, T. C. 2008. BDISTMDS software, v. 2.0. Center for Origins Research, Bryan College. Distributed by the author.
Wood, T. C. 2010. Baraminological Analysis Places Homo habilis, Homo rudolfensis, and Australopithecus sediba in the Human Holobaramin. Answers Research Journal 3: 71–90.
Wood, T. C. 2011. Using creation science to demonstrate evolution? Senter’s strategy revisited. Journal of Evolutionary Biology 24, no. 4: 914–918.
Wood, T. C., and M. J. Murray. 2003. Understanding the pattern of life: Origins and organization of the species, ed. K. Wise. Nashville, Tennessee: Broad & Holman Publishers.
Wood, T. C., M. Ross, and P. A. Garner. 2011. Detecting discontinuity in the Dinosauria using baraminic distance correlation, CBS Annual Conference Abstracts. Journal of Creation Theology and Science Series B: Life Sciences 1: 26–27.
Xu, X., M. A. Norell, X. Kuang, X. Wang, Q. Zhao, and C. Jia. 2004. Basal tyrannosauroids from China and evidence for protofeathers in tyrannosauroids. Nature 431: 680–684.
Xu, X., K. Wang, K. Zhang, Q. Ma, L. Xing, C. Sullivan, D. Hu, S. Cheng, and S. Wang. 2012. A gigantic feathered dinosaur from the Lower Cretaceous of China. Nature 484, no. 7392: 92–95.
Zanno, L. E., and P. J. Makovicky. 2011. On the earliest record of Cretaceous tyrannosauroids in western North America: implications for an Early Cretaceous Laurasian interchange event. Historical Biology 23, no. 4: 317–325.