Research conducted by Answers in Genesis staff scientists or sponsored by Answers in Genesis is funded solely by supporters’ donations.
Abstract
Despite having many avian characteristics, Microraptor is often reconstructed to look like a transitional form between dinosaurs and birds. A creationist reconstruction of Microraptor is needed to show how birdlike it would have appeared in life. The author performs a systematic, comparative anatomy of both form and function to show whether Microraptor would have appeared avian or reptilian. Comparing the forelimb, hindlimb, tail, and skull anatomy of Microraptor with dinosaurs and both extinct and extant birds shows that Microraptor had an avian hand with a swivel wrist and flight feathers, wings capable of full flapping motion, wings that folded against the body at rest, crouched legs that pivoted from a stable knee, hip structure to support avian walking, a short tail with unfused pygostyle, and a beaked rostrum. These characteristics show that Microraptor had an appearance very similar to that of a modern bird.
Introduction
Microraptor is often reconstructed to look like a transitional form between dinosaurs and birds. This paper is not intended to prove the avian nature of Microraptor. Paleornithologist Alan Feduccia (2020) has already done an admirable job making that case. It instead assumes that Microraptor is a bird and explores an anatomical reconstruction from that viewpoint. If Microraptor was indeed a bird then it was created on day five of creation week as one of the “birds” (Genesis 1:21, ESV) or “winged fowl” (Genesis 1:21, KJV). A reconstruction using a biblical worldview would be expected to show some anatomical and functional similarities to living winged fowl. This paper evaluates the anatomical data to show whether Microraptor looked like a transitional form as often depicted, or whether it looked more like an extant bird. This is done by comparing the forelimb, hindlimb, tail, and skull anatomy of Microraptor with the anatomy of dinosaurs and both extinct and extant birds.
Reconstructions of extinct animals are helpful components to the study of paleontology. Two common types of reconstructions are skeletal and life reconstructions. Skeletal reconstructions are commonly drawn as a silhouette of the skeletal elements articulated as in life from a lateral perspective (fig. 1). Life reconstructions put flesh and integument on the bones to estimate what the living creature looked like (figs. 2 and 3). These drawings and paintings are often referred to as paleoart.
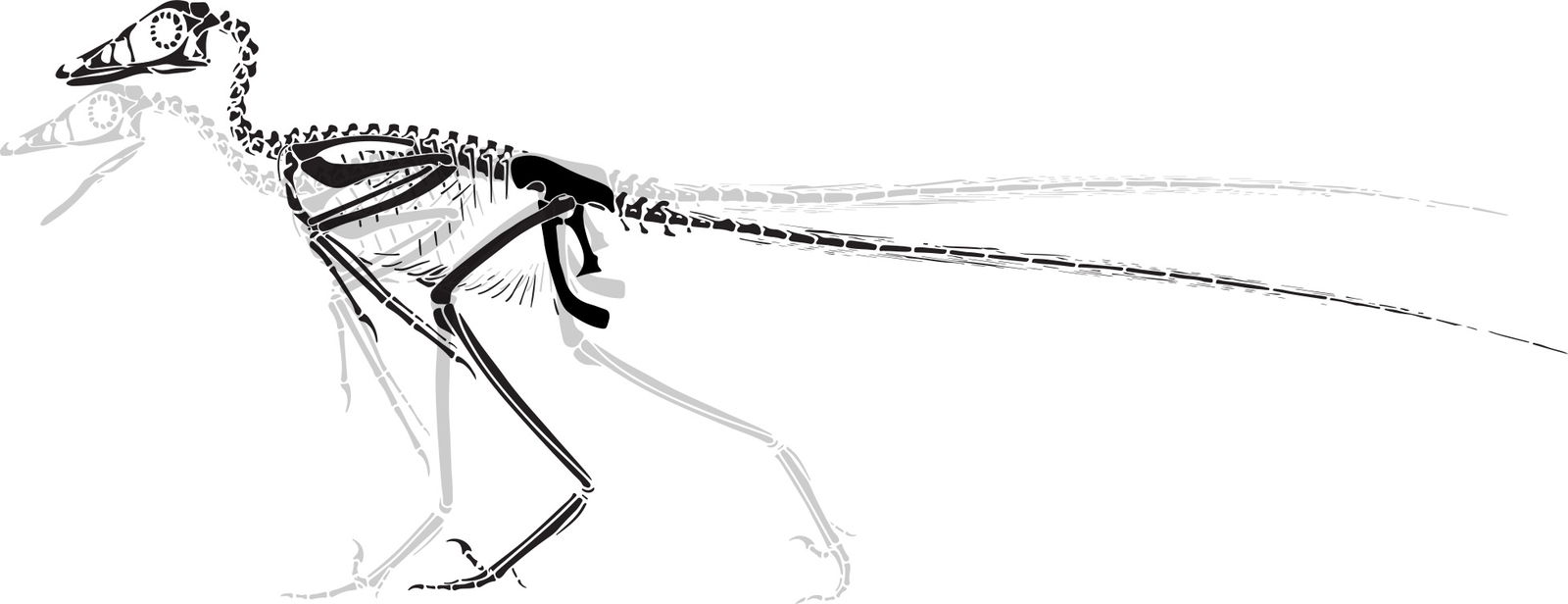
Fig. 1. Microraptor skeletal reconstruction. Dinosaur posed skeletal (gray) adapted from Hartman (n.d.) reconstruction. Bird posed skeletal (black) based on Paul (2016), Hartman (n.d.), and Headden (2006) as reference as well as specimen photos. Skeletal dimensions were adjusted based on measurements by Smithwick (2019).
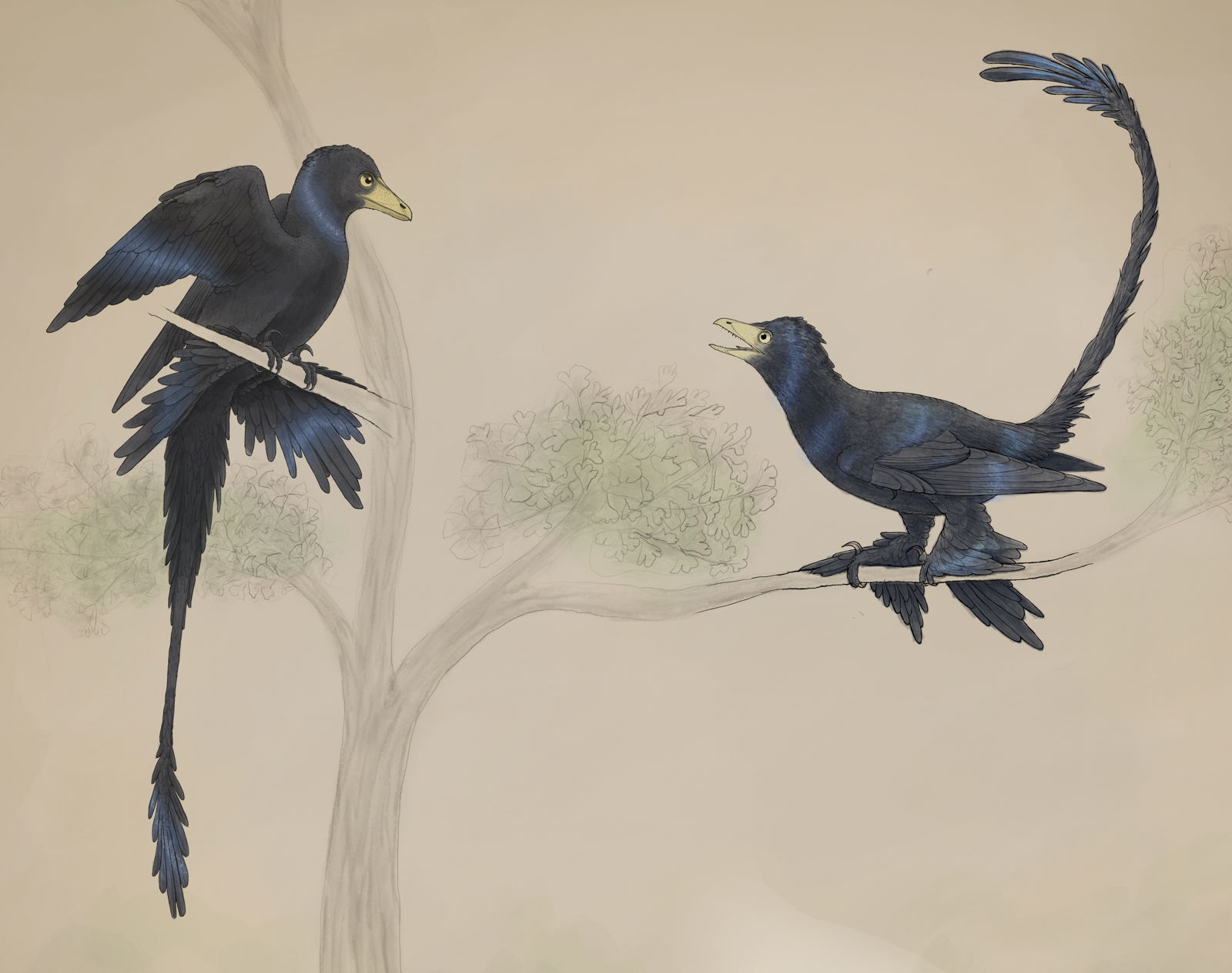
Fig. 2. Life reconstruction of a pair of male Microraptors. Anatomical proportions laid out in 3D (Blender 3.1). Initial artwork done using Procreate 5 on iPad, and finishing work done in Adobe Photoshop. Joel Leineweber 2022.
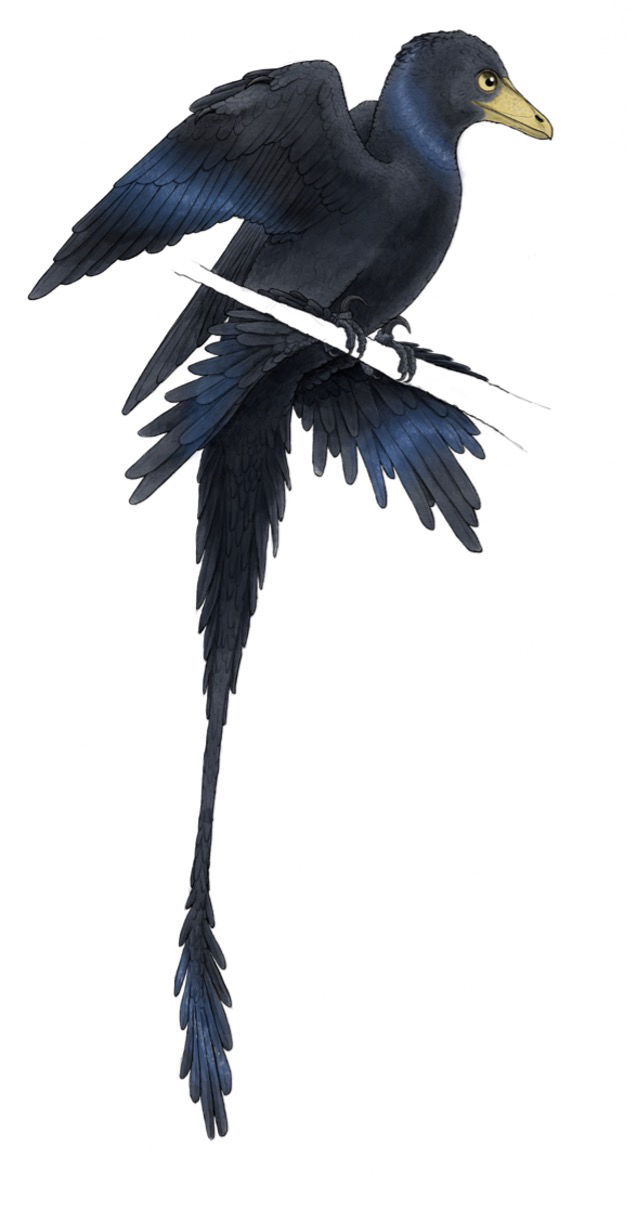
Fig. 3. Life reconstruction of Microraptor. Anatomical proportions laid out in 3D (Blender 3.1). Initial artwork done using Procreate 5 on iPad, and finishing work done in Adobe Photoshop. Joel Leineweber 2022.
Paleoart inspires and stimulates curiosity. It’s an important addition to research that helps encourage interest in topics that might otherwise have gone less noticed. Paleoart is very important when one is trying to help the reader visualize a concept that is unexpected or unconventional. When the reader is accustomed to seeing an animal represented a particular way it is often difficult to imagine them differently, even if the new representation is better supported by the evidence.
Reconstructions are visually accessible and can often capture a “thousand words” in a single image. The visual is more approachable and can help technical or lay readers comprehend anatomical descriptions.
Reconstructing extinct creatures from fossil evidence requires numerous assumptions and worldview-based paradigms. This reality applies to life reconstructions because soft tissue is not often preserved. It must be inferred or interpreted in most cases. Some do not realize that this is also true for skeletal reconstructions. Skeletal reconstructions contain much more information than just the bones. For example, a skeleton is often drawn as complete when portions of that taxon have never been found but were instead inferred from other similar taxa. The positioning of the bones, angles of articulation, and pose of the animal all convey ideas that may or may not be conclusive from the available fossil evidence. A famous example of this in paleoart is Robert Bakker’s Deinonychus illustration (Ostrom 1969). Often a silhouette is drawn behind the skeleton which implies soft tissue elements that were, in most cases, not preserved.
In addition, skeletal reconstructions often exclude important information regarding the fossil, such as the quality of preservation, level of articulation, and proximity of association. They often combine information from multiple specimens to represent a complete picture of the animal, but rarely are the specific data sources documented.
Knowing that one’s worldview affects the many decisions that are made in the reconstruction process should cause researchers to be more aware and carefully evaluate reconstructions before basing conclusions on them.
Methodology
Worldview Approach to Reconstructions
Scientists often pride themselves in being unbiased, so one must be careful not to judge or assume another’s motives (1 Corinthians 4:5). The bias we are referring to is less about the bias between two clear hypotheses and more about the often-hidden presuppositions that are present in everyone’s worldview.
Presuppositions are often subconscious unless the researcher is accustomed to examining their own presuppositions. Two examples of worldview glasses are the creationist vs. the evolutionist worldviews. These starting points create significantly differing expectations.
For example, the evolutionist’s starting point creates the expectation of a discordance of individual features gradually changing in small increments unrelated to each other and progressing from primitive to advanced. The creationist’s starting point, on the other hand, creates an expectation of fully complete features designed together in functional groups that often correspond with larger design patterns in living animals. These expectations affect (often unknowingly) how a researcher interprets the evidence.
Presuppositions are evident most often in the absence of evidence where conclusions must be inferred. In paleontology, the researcher is always missing some amount of data even in the most complete fossil specimens. This leaves numerous opportunities for assumptions and conclusions influenced by the researcher’s presuppositions to appear, either knowingly or unknowingly.
The most common result of this is assumptions based on the supposed phylogeny, often depicted with a cladogram, of an animal. Feduccia (2020, 8) says:
The other insidious trap of the current paleontological methodology is that once a cladogram is rendered, all biological inference must accommodate the cladogram; if the cladogram is incorrect then all evolutionary knowledge that flows from the cladogram will be in error.
Circular reasoning is often utilized when developing cladistics models. Animals are said to be evolving because they had evolving features. And evolutionists think the animals had evolving features because they were supposedly evolving. An example is the assumption that maniraptors are theropods. James and Pourtless (2009, 1) summarize:
We reanalyzed a standard matrix of 46 taxa and 208 characters from a recent paper by Clark, Norell, and Makovicky, and we found statistical support for the clades Coelurosauria and Maniraptoriformes and for a clade of birds and maniraptorans. Note, however, that because the matrix contains only birds and theropods, it assumes that the origin of birds lies within the Theropoda. In addition to this problem, Clark et al.’s [Chiappe and Witmer 2002] matrix contains problematic assumptions of homology, especially in the palate, basipterygoid, manus, carpus, and tarsus.
The data set they analyzed assumed the conclusion and then reached that conclusion by including data that would reinforce the conclusion. James and Pourtless (2009, 1) came to a different conclusion and stated, “the verificationist approach of the BMT literature may be producing misleading studies on the origin of birds.”
Biblical Worldview Approach
The following bullet points summarize the methodology used when approaching evidence with an intent to create a reconstruction with a biblical worldview.
-
Use living animal anatomy as a guide for extinct animals wherever logical.
- Look at traits in living animals to find feature correlations or similar design patterns. Similar design patterns are expected with the same created kind or even across created kinds because of a common Designer.
-
Look for evidence of fully functioning features (logical, fully-working, not half-working or half-evolved).
- Interdependent organ systems (not individually evolved, for example, feathers + uropygial gland + beak + wings)
- Functional design (do not assume vestigial features)
- Intentional design (not accidental)
-
Reference evidence from animals of the same family group, if possible.
- Remove evolutionary-tree-assumed presence or absence of features
- Family classification (created kind/holobaramin)
- Class/order grouping (apobaramin)
- Look for features that align with beautiful design principles (elegant solutions, aesthetically pleasing)
The remainder of this paper will describe the reasoning for each of the decisions made in the process of reconstructing Microraptor. This will be done as a survey of the anatomy of this animal to show whether it should be visualized as a transitional form or more like an extant bird.
Note that this approach is unapologetically functional in describing and reasoning about this genera’s features. While care was taken to review multiple cladistics data sets (Brusatte et al. 2014; Lee et al. 2014; van der Reest and Currie 2017), the characters were examined on an individual basis as a way of informing the various locomotor groups and high-level functions (flight, locomotion, feather care, etc.).
It should also be noted that the traditional meaning is intended when the words theropod, feather, and bird are used in this paper and not a more recent meaning influenced by evolutionary ideas. In particular, the word theropod here refers to theropod dinosaurs as defined by Othniel Charles Marsh in 1881.
Wings
According to Feduccia (2020, 272), Microraptor was a bird with wings similar to those of modern birds. The wings of extant birds are an entire system of features designed to work together. Evolutionists look for evidence of each of these features evolving independently, but God designed systems like this as irreducibly complex systems. A modern bird-like reconstruction of Microraptor’s wing is consistent with the anatomical data available as shown by the features below.
Swivel Wrist
The swivel wrist with a semilunate carpal is a key part of the “avian flight hand” that Feduccia (2020) considers an avian diagnostic feature of Microraptor. This half-moon shaped bone enables the wrist to swivel sideways, opposite to the usual theropod wrist movement. Modern birds share this feature. In adults, this carpal bone is fused to the metacarpals as part of the carpometacarpus; however, in juveniles it is not fused, as seen in fig. 4 (personal observation).
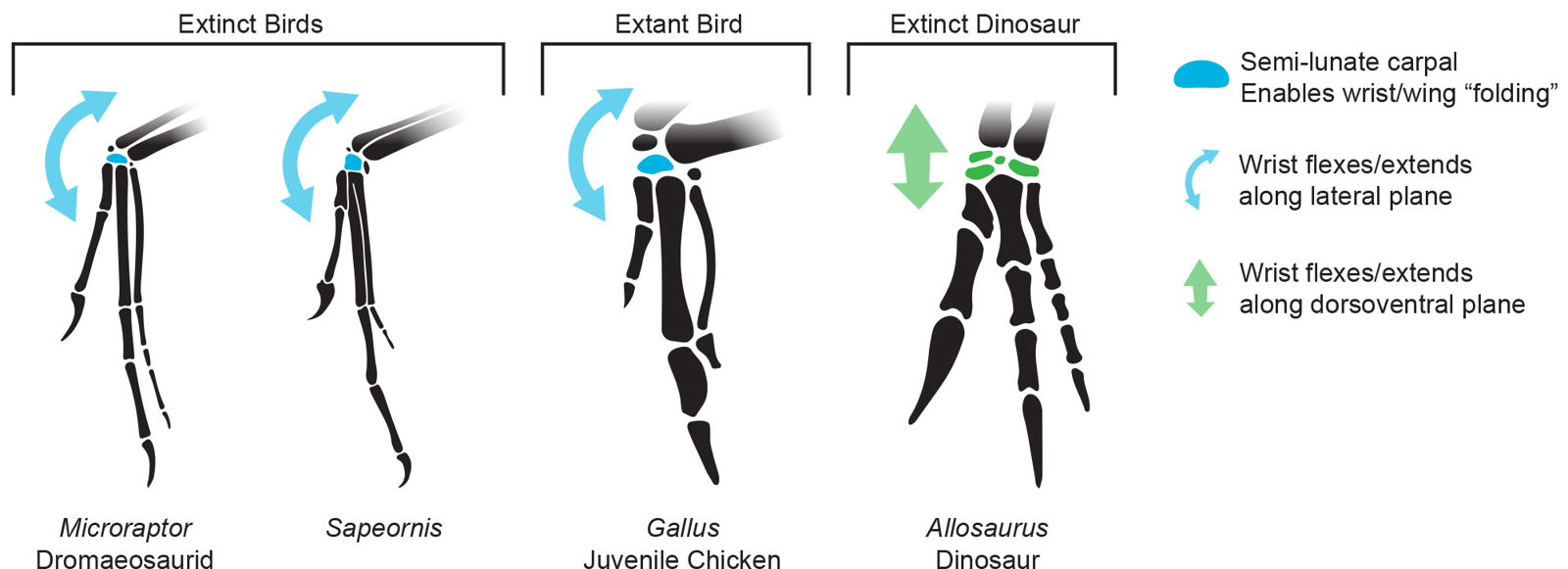
Fig. 4. Microraptor has a semilunate carpal very similar to that in extinct birds such as Sapeornis and to extant juvenile birds like Gallus. This feature enables the wrist to swivel opposite to the typical theropod wrist motion like in Allosaurus. The Microraptor, Sapeornis, and Allosaurus reconstructions were adapted from Hartman (n.d.) reconstructions. Juvenile Gallus drawn from specimen photos. Individuals scaled to equal manus length for easier comparison.
Feduccia (2020, 273) summarizes this feature when he says that Dromaeosauridae are “characterized by avian features, the presence of pennaceous avian feathers, and a semilunate carpal, which allows a swivel-like movement of the wrist, essential in flight. Classic theropods (exemplified by Coelophysis, Allosaurus, etc.) lack such a carpal element.”
(Burnham 2008) states about Microraptor that “the arm was able to fold like a bird’s wing because of the arrangement of the wrist bones.”
Evolutionists have a difficult time explaining what caused this dramatic change in forelimb function. Meadows (2014, 1) describes it this way:
Somewhere along the way from early dinosaurs to birds, wrists changed so much that we could be excused for thinking birds don’t even have them. Wrists went from straight to bent and hyperflexible, allowing birds to fold their wings neatly against their bodies when not flying. Underlying this change is a drop in the number of wrist bones from nine to just four.
The paper that Meadows is summarizing (Botelho et al. 2014) multiple times compares “birds and bird-like dinosaurs” to other theropod dinosaurs. They defined bird-like dinosaurs as “the closest nonavian relatives of birds—that is, maniraptorans like Oviraptorosauria, Dromaeosauridae.”
In reconstructions, the wrist of Microraptor should never be shown as a grasping wrist, pulling things toward the midline of the body. Instead, the wrist folds sideways beside the body just as would be expected of a bird wing.
Digit Position and Mobility
In extant adult birds the metacarpals and some of the carpals fuse to make the carpometacarpus. While in Microraptor the bones are not fused via ossification, they do appear to act as a single unit like in modern birds. This is consistent with the modern juvenile avian condition as seen in fig. 5. This appears to severely limit mobility of the digits in the manus.
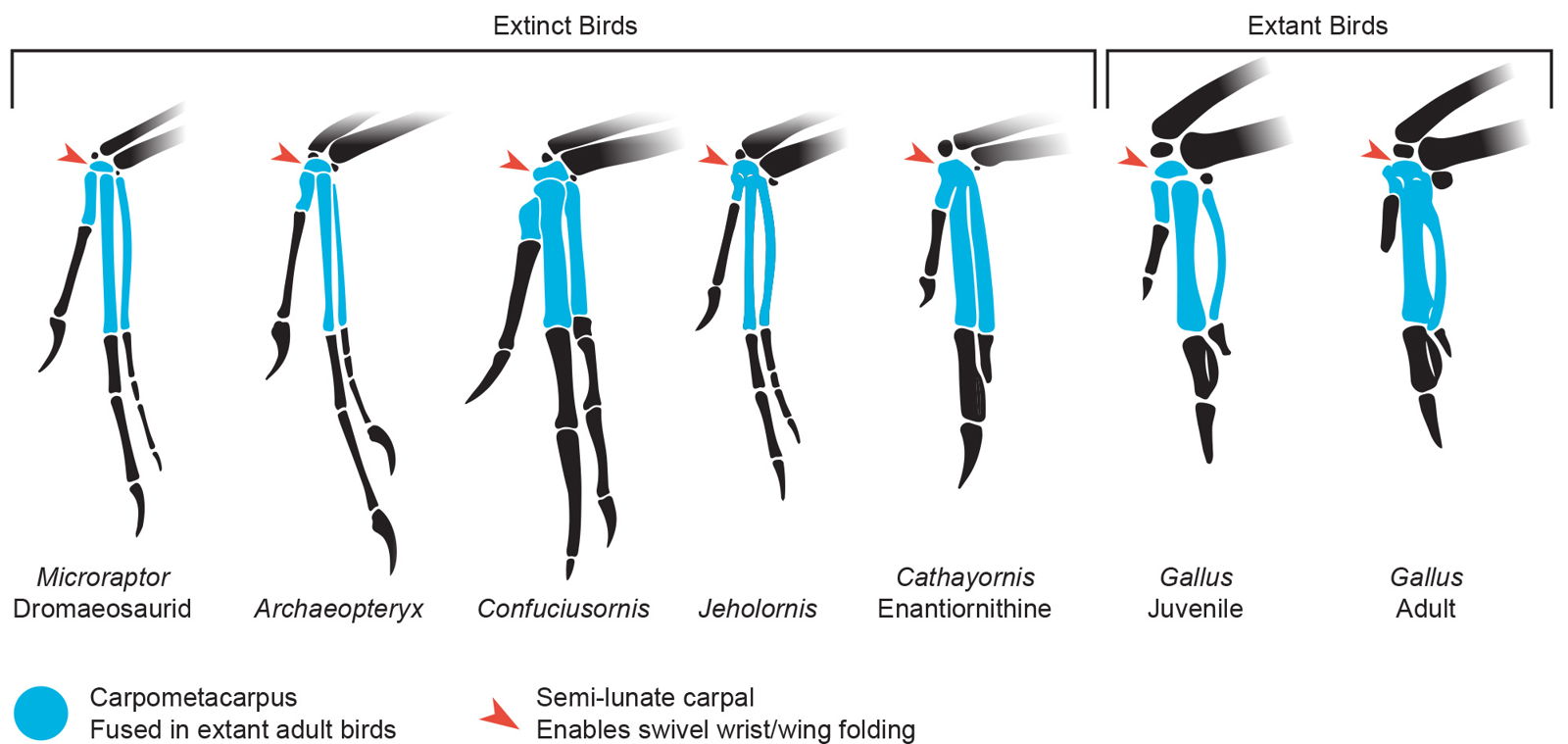
Fig. 5. Microraptor and the juvenile Gallus show similar digit anatomy. The four bones making up the carpometacarpus appear to function as a single unit even if they do not retain the full bone fusion of modern adult birds. Microraptor, Archaeopteryx, Jeholornis, Confuciusornis, and Cathayornis (extinct birds) all represent varying amounts of bone fusion of the carpometacarpus.Microraptor, Archaeopteryx, Confuciusornis, Jeholornis, Cathayornis, and adult Gallus drawings adapted from Hartman (n.d.) skeletal reconstructions. Juvenile Gallus bones drawn from specimen photos. Individuals scaled to equal carpometacarpus length.
Some extinct birds like Microraptor exhibit a lack of ossified bone fusion very similar to young extant birds as Heers and Dial (2012, 301) describe:
Similar to many fossilized theropods, but unlike their adult counterparts, immature chukar (Alectoris chukar; and presumably juveniles of other precocial species . . . ) have unfused thoracic vertebrae, an unfused synsacrum and small pelvis, an extremely small keel, no V-shaped ulnare (wrist bone) and tarsal (ankle) bones that are not fused to the tibia or metatarsus.
There are several possible explanations for this lack of bone fusion. Perhaps there is a preservation bias for younger individuals to be buried earlier in the Flood because they could not fly as far. Hebert (2023) collected evidence of slower and longer growth in clams and crocodiles, for example. It is also possible that these avian baramins were created to have less bone fusion. This “arrested development,” as Feduccia (2020) labels it, does not necessitate a non-avian status. In fact, Feduccia says that this arrested development distorts the phylogenetic analysis by making these animals appear more “basal” than they actually are. Hopefully newer discoveries and research will continue to shed light on this phenomenon. For example, a microraptorine was found recently which had a “fusion of the pubes to the ilia” (Xing and Feng 2016).
Burnham (2008, 39) states “it is possible that [Microraptor’s] second metacarpal is somewhat fused with the outer metacarpal since most specimens that were examined have these two metacarpals appressed.”
Feduccia and Czerkas (2015, 4) take this further, observing the following:
There is a very close adherence of the outer and middle fingers, providing support for anchoring primary feathers, which is also true for Microraptor and Confuciusornis. Although these forms as well as Caudipteryx are often restored with separated fingers engaged in some type of presumed theropodan predatory behavior, such activity would not have been possible.
Some evolutionary reconstructions show fingers awkwardly sticking out from part of the wing or something akin to a theropod arm and hand with feathers tacked onto the forearm. This requires exposed fingers at some stage because a mechanism is needed to transition arms into wings. Commonly proposed mechanisms for this transition include predatory grasping and tree climbing which both require exposed claws and digits. The presence of long primary feathers attached to the middle finger makes both of these mechanisms problematic. As Feduccia and Czercas (2015) point out, the second and third digits would be joined together by soft tissue placing them inside the wing (see Pro/Postpatagia below).
All three digits play important functional roles in the extant avian wing. The first digit has some mobility and acts as an attachment for the alula feathers which birds use to help generate lift and prevent stall during descent (Lee et al. 2015). The middle digit acts as the attachment for the primary flight feathers. The last digit helps to stabilize and control the primary flight feathers. These flight-hand digits are so specialized they are highly unlikely to be used for grasping (Hutson and Hutson 2018).
One small difference, seen in fig. 5, is that the third metacarpal on Gallus is more curved than the other taxa. This is common in extant birds, however, as can be seen in the skeletal drawings of Katrina van Grouw (2013), the curvature of that bone varies by genera. Chatterjee and Templin (2012) observe in Microraptor a “strongly bowed outer metacarpal” and “a flattened digit for attachment of the primaries.”
Despite having unfused manual digits, modern juvenile birds have little more flexibility than an adult (personal observation). This lack of digit mobility appears to be the case for Microraptor where the manus would have swiveled and moved as a single unit, not as three independently grasping fingers. In extant juvenile birds this is mostly due to soft tissue including cartilage, ligaments, tendons, and the postpatagia. In light of these observations, especially the inferred inability of Microraptor to grasp if its digits were positioned as “fingers” sticking out from a partial wing, reconstructions should show a functional wing configuration (see Appendix).
Propatagia and Postpatagia
Preservation of soft tissues such as patagia is rarely observed, but when they are it provides important clues for creating more accurate reconstructions of extinct animals. One such soft tissue structure that is distinct in birds is the postpatagia.
The postpatagia is a complex of muscles and tendons designed to connect to the skeletal system for passive feather motion. It also includes a collection of ligaments that surround the primary and secondary feather attachments. Hieronymus (2016, 2) notes that “these structures are well-placed to directly link elements of the passive musculoskeletal coordination system with flight feather movements”. Zheng et al. (2017) describe it as “dorsal and ventral dermis, fibrous network, muscles, ligaments, fascia.” Feduccia and Czerkas (2015) note that the “cambered propatagium is the major lift-generating component of the wing proximal to the wrist . . . its presence is considered a highly reliable, if not unequivocal, indicator of flight.” The complexity and specialization of this structure make it clear that it was designed as part of a fully functioning wing system.
The postpatagia also has the side effect of binding the second and third digits together to support the primary feathers. Xiaoli Wang et al. (2017b) document several specimens including Microraptor which have evidence of both propatagia and postpatagia. Zheng et al. (2017) also document the propatagial tendon in Microraptor as do Agnolin and Novas (2013) where they observe that the “presence of extensor process on carpometacarpus may indicate that most averaptorans possessed a well-developed propatagium.” When the wing is closed it folds out of the way and when the wing is open it acts as an airfoil that helps the wing generate lift.
In addition, during wing extension the propatagial tendon helps enable the auto-extension feature of bird wings. When the elbow extends, the swivel wrist automatically extends simultaneously, opening the wing and vice versa.
We suggest that reconstructions should show the second and third digits of the manus as attached by soft tissue. Movement would have been likely limited, but what movement was possible would have probably occurred in unison with bones of the whole wing, as in modern birds. The first digit may have had some independent mobility as in extant birds, but only from the distal phalange and claw. Its movement would have been along a single plane, not as mobile as in the human opposable thumb.
We suggest reconstructions display the forelimb as shaped like a wing, not a theropod arm. This means the elbow would never extend fully due to the propatagial tendon, and the flap of the propatagia would span from the shoulder to the wrist as seen in fig. 6. The postpatagia would add significant soft tissue to the trailing edge of the forelimb as well and it appears to have extended from the elbow all the way to the last phalange of the third digit as in extant birds (see Appendix).
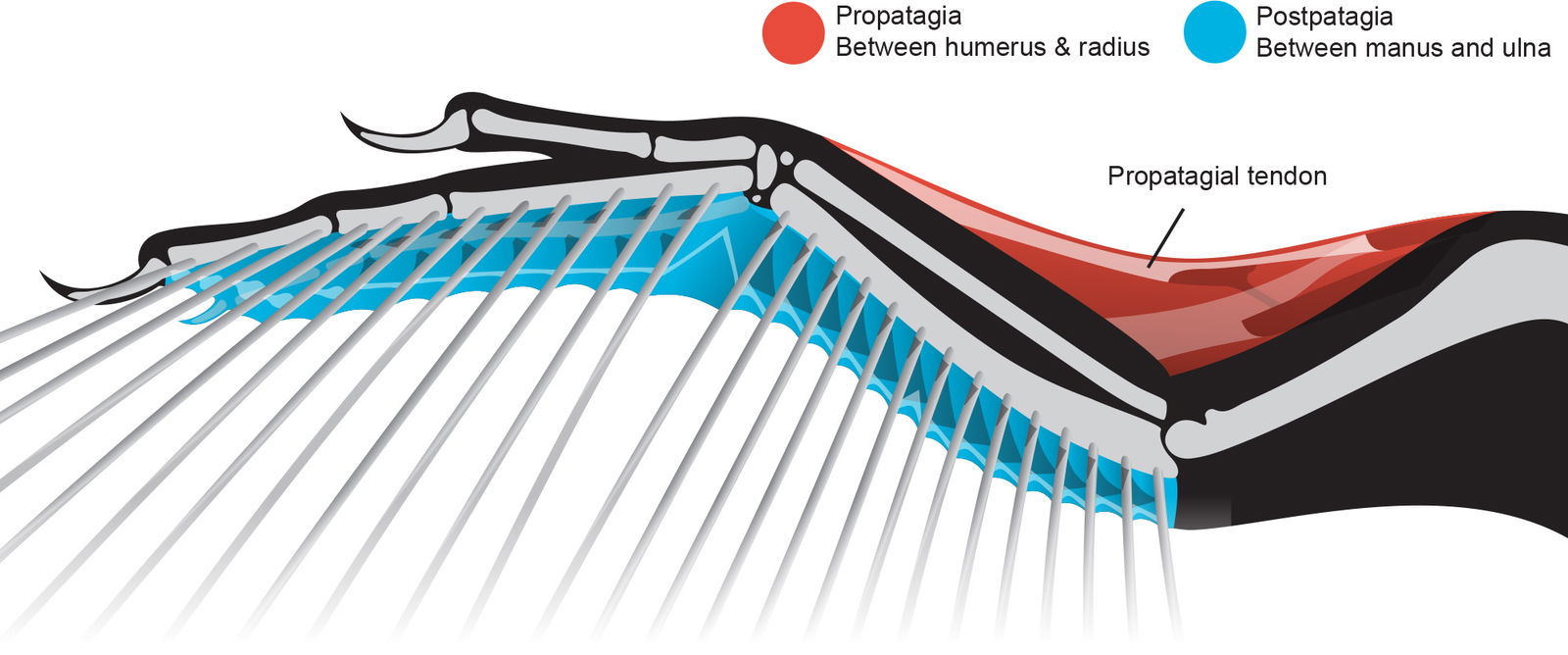
Fig. 6. The propatagia and postpatagia are a complex system of muscles and tendons that make up part of the specialized avian wing. Evidence of both has been found in Microraptor specimens. Specific tendon and muscle anatomy inferred from Hieronymus (2016). Microraptor skeletal drawing adapted from Hartman (n.d.) reconstructions.
Wing Claws
Some think that wing claws are an anomaly in birds. The reality is that more than half of the extant orders of birds have taxa with one or two claws on their wings (Fisher 1940, fig. 7). The claws are usually hidden by feathers so many assume they do not exist. But according to Fisher, claws have been found on the wings of ratites (fig. 8), vultures, hawks, eagles, parrots, blackbirds, swifts, screamers, terns, kites, chickens, geese, and ducks, just to name a few.
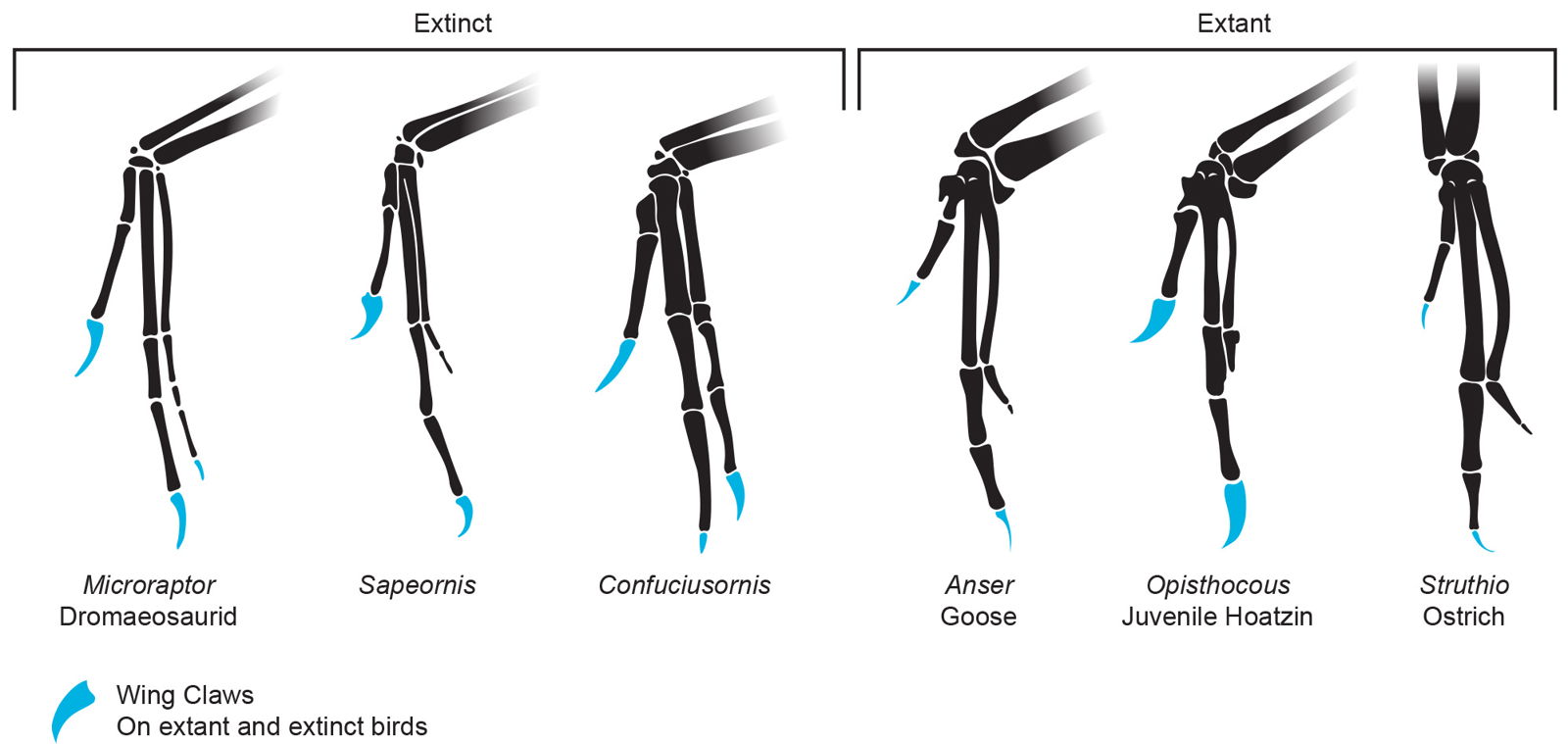
Fig. 7. Note the claws on the ostrich, goose, and hoatzin, and the three clawed digits on the extinct birds Confuciusornis and Sapeornis. Skeletal drawings adapted from Hartman (n.d.) reconstructions and photos of specimens. Individuals scaled to equal manus length.
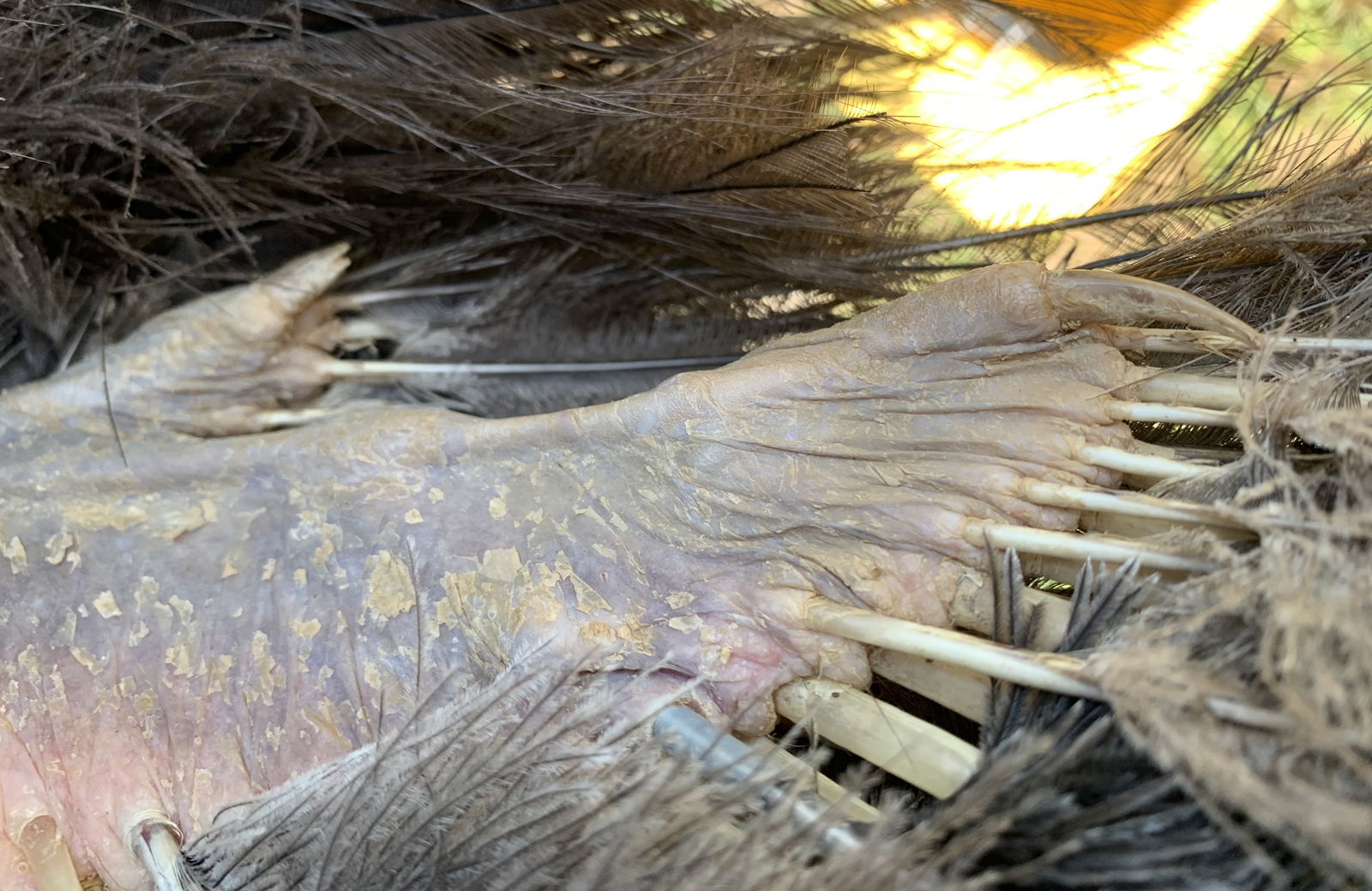
Fig. 8. Large claws on the first two digits of an extant ostrich manus. Photo taken at the Ark Encounter’s Ararat Ridge Zoo.
Claws on some extinct birds appear to be larger and more common. One of the most numerous extinct birds, Confuciusornis, had three clawed digits on its wings (Chiappe et al. 1999). Evolutionists often use this difference to call extant bird wing claws “vestigial.” However, this is an assumption and as a result it seems very little research has been done on extant bird wing claws to determine their function. From a creationist worldview, God would likely have created this feature with a function in mind, perhaps for feather or flight stabilization or self-defense.
Some evolutionists claim that flight evolved as a result of animals like Microraptor climbing trees and then gliding down (Norberg 1985). Biblical creationists, however, need no such explanation for the origin of flight, so the idea of birds using their wings to climb seems improbable since these claws were likely hidden by feathers as in extant adult birds that do not use their claws to climb (fig. 8).
Predatory birds like Microraptor may have used their wing claws to strike prey during a mounting type of attack (Fowler et al. 2011). A similar mounting behavior can be seen in extant eagles and hawks today. Some have claimed that ostriches use their wing claws for self-defense or when fighting over a mate, but surprisingly few studies have been completed about the function of wing claws on extant birds.
Paleoartists often show fingers and claws as clearly exposed on the wings of Microraptor. However, as has been shown, a proper understanding of avian anatomy reveals that the second and third digits are “inside” the wing, covered with feathers, so at least the second and third digits’ claws were likely hidden. The claws on the first and second digits may have been visible from some angles as drawn for Microraptor (fig. 9) and as in ratites (fig. 8), but they also may have been completely hidden by contour feathers most of the time as in extant birds.
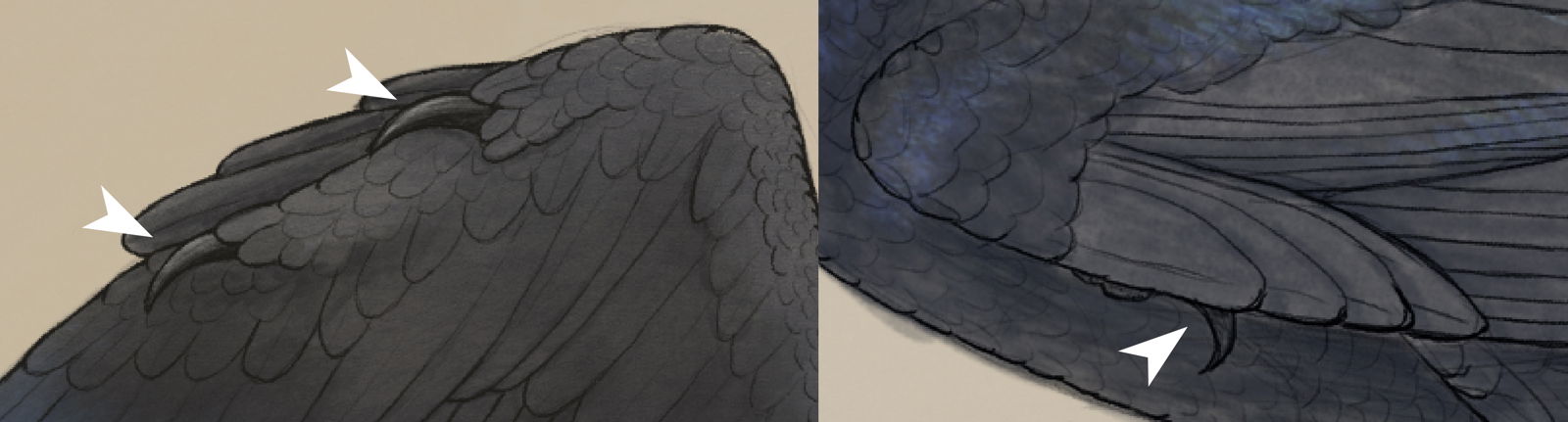
Fig. 9. First and second digit claws may have been visible on the ventral side of the wing of Microraptor or (more likely) may have been completely hidden by feathers as in extant birds.
Flight Feathers
Feduccia (2020) called avian flight feathers “the most sophisticated of the vertebrate integument” and “perfections of aerodynamic engineering.” Extant avian wing flight feathers are arranged into two main groups based on their attachment points. Primary feathers attach in a row (spaced apart but overlapping) to the trailing edge of the phalanges of the second digit of the manus. Secondary feathers are attached in a row (spaced apart but overlapping) along the trailing edge of the ulna. The feathers are arranged in a fan-like shape, with the proximal-most secondaries almost parallel to the body and the distal-most primaries angled more perpendicular to the body when the wing is open. The length of each feather is designed to create a unique wing shape that serves the primary function of the wing for each taxon. The feathers are individually rotated, carefully overlapped, with slightly curved quills to create an airfoil to maximize aerodynamic function in birds with powered flight (fig. 10).
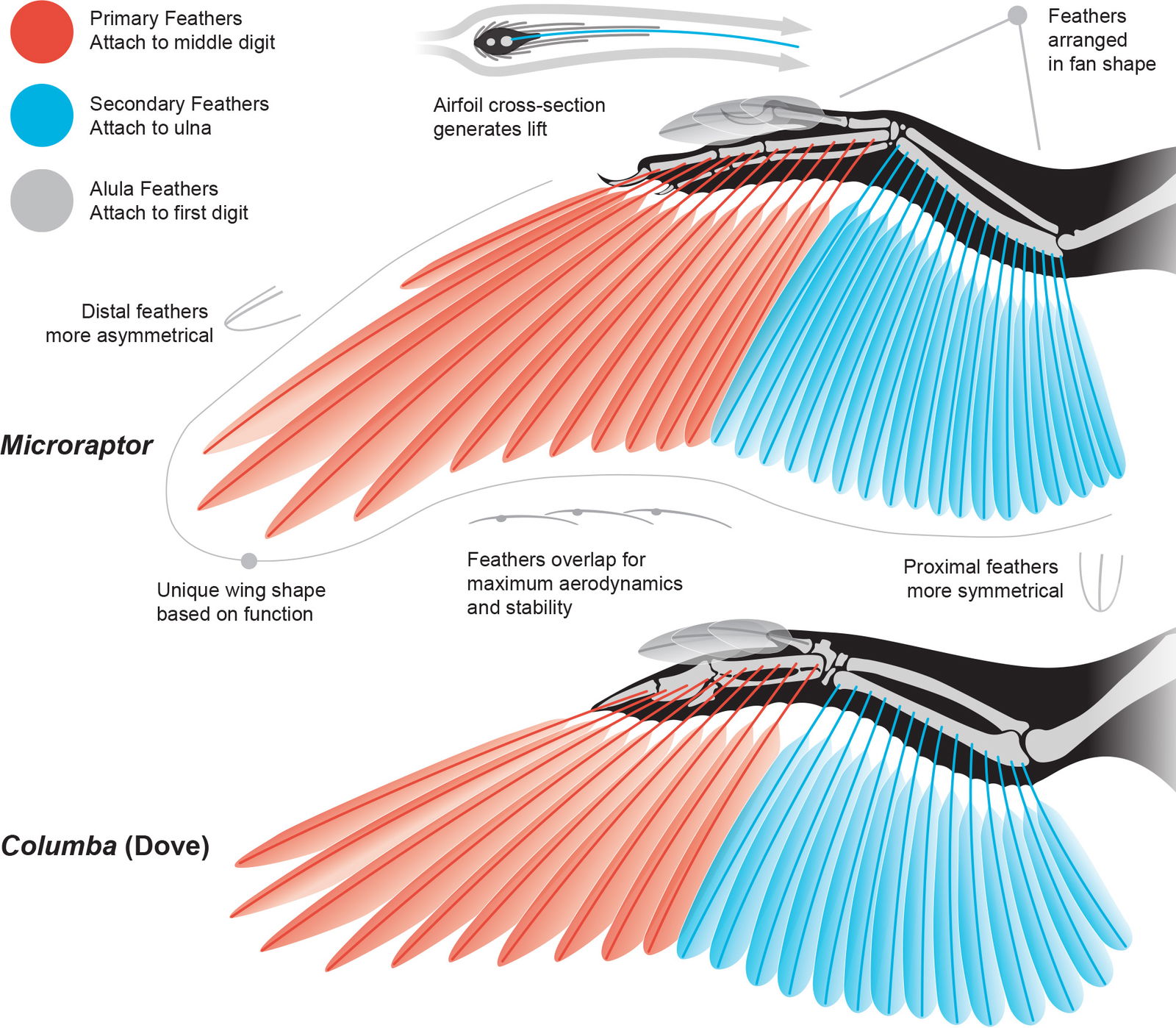
Fig. 10. Microraptor had pennaceous primary and secondary wing feathers indistinguishable from extant birds. Individuals are scaled to the same forelimb length. Microraptor skeleton adapted from Hartman (n.d.). Microraptor feather reconstruction based on Li et al. (2012) and Chatterjee and Templin (2007). Columba (dove) anatomy based on Wellnhofer (2009, figure 6.36), Hieronymus (2016), and Lingham-Soliar (2015).
In addition, when the wing folds against the body, the feathers automatically change their attachment angle and overlap each other more, collapsing the large airfoil into a compact package that hugs the trunk. This keeps the feathers safe and protects the body.
This design is not seen anywhere in creation other than the avian wing. Certainly, God could have created dinosaurs with some type of feathery integument. However, the feathers found on Microraptor forelimbs are clearly wing feathers that match the elaborate engineering of those on extant birds as confirmed by Hone et al. (2010) using ultraviolet light.
Lü and Brusatte (2015, 6) confirm this avian feather arrangement in Microraptor when discussing the dromaeosaur, Zhenyuanlong:
Both the primaries and secondaries are more than twice the length of the humerus, as in Microraptor and modern birds . . . the secondaries are oriented approximately perpendicular to the ulna whereas the primaries are positioned at an acute angle to the manus, which becomes less acute and approaches 90 degrees as the feathers progress proximally up the arm. A very similar arrangement is also seen in Microraptor.
Xu et al. (2003, 338) provide a detailed description of the avian wing feathers on Microraptor:
The primaries (approximately 12 in number) are significantly longer than the secondaries (approximately 18 in number); the most distal primaries are more or less parallel to the manus, and the others are at angle to the manus, with the angle increasing from distal to proximal. The longest primaries (incompletely preserved) are 2.7 times as long as the humerus or 2.3 times as long as the femur. Some primaries on the holotype display asymmetry, with the leading vane much narrower than the trailing vane. The secondaries are longer than the humerus and more or less perpendicular to the ulna. The proximal ones have symmetrical vanes and the distal ones display weak asymmetry (Fig. 2h). The presence of a few relatively small feathers attached to the manual digit I (Fig. 2i) on the holotype is noteworthy. These display well-organized pennaceous vanes, and might be the precursor to the alula, which is associated with flight control and which is present in most birds.
Kiat et al. (2020) present evidence that Microraptor’s wing feathers molted sequentially. This functionality is part of the advanced wing feather system that God designed so volant birds can continue to fly even during their molting season. Microraptor’s wing feathers appear to be part of such a system found only in birds.
Kiat and O’Connor (2024) report that the number and arrangement of primary and secondary flight feathers corresponds with flight ability in extant birds. They state that “Mesozoic birds and Microraptor have remex morphologies consistent with extant volant birds.”
The evolutionary bias for short “proto-wing” feathers is prevalent in early reconstructions of Microraptor and others. But as shown by later Microraptor specimens found with well-preserved feathers, this is inaccurate. This bias can be seen by comparing the Sinornithosaurus illustration from Paul (2002, Figure 6.1) (Paul classified Microraptor as part of the Sinornithosaurus genus at the time) with the illustration of Sinornithosaurus from Paul (2016). Before feathers had been found on Microraptor it was drawn climbing in trees with short fiber-like protofeathers and a hairy lemur-like tail. After feathers were discovered, this dramatically changed the reconstructions (Supplementary fig. 3).
Creationist reconstructions should pay careful attention to feather counts, lengths, and arrangement to accurately represent Microraptor’s wings as avian (see Appendix).
Alula Feathers
The alula in birds helps with flight by improving lift and preventing stall at low speeds. In extant birds the alula is composed of two to six feathers attached to the first digit (Lee et al. 2015).
Digit I possesses flight feathers on all extant flying birds. While it is possible that some extinct birds lacked an alula, this should not be assumed. A lack of alula feather preservation is not conclusive evidence of the feature being completely absent. The manus of Microraptor has a mobile joint on the first digit very similar to that of extant birds. The absence of alula feathers in some pre-Flood birds may be due to a preservation bias or an artifact of degradation in the Flood waters. Evolutionists see the alula as a stand-alone feature which had to evolve separately, so their methodology tilts them toward not showing an alula on animals they consider more basal or primitive. In artwork they often take the opportunity to also add scales (scutes) to the fingers to make them appear more reptilian. Depictions should avoid this evolutionary phylogeny-based thinking.
Xu et al. (2003) claim that the pennaceous feathers found on the first digit of Microraptor’s manus may be a “precursor to the alula.” Agnolin and Novas (Agnolin and Novas 2013; Agnolin et al. 2019) interpret these feathers as an alula in Microraptor (fig. 11). From a biblical worldview this is most likely a fully-functioning alula. Zheng et al. (2017) includes a diagram that shows the presence of alula feathers in Microraptor, a confuciusornid, Eoenantiornis, and Archaeornithura.
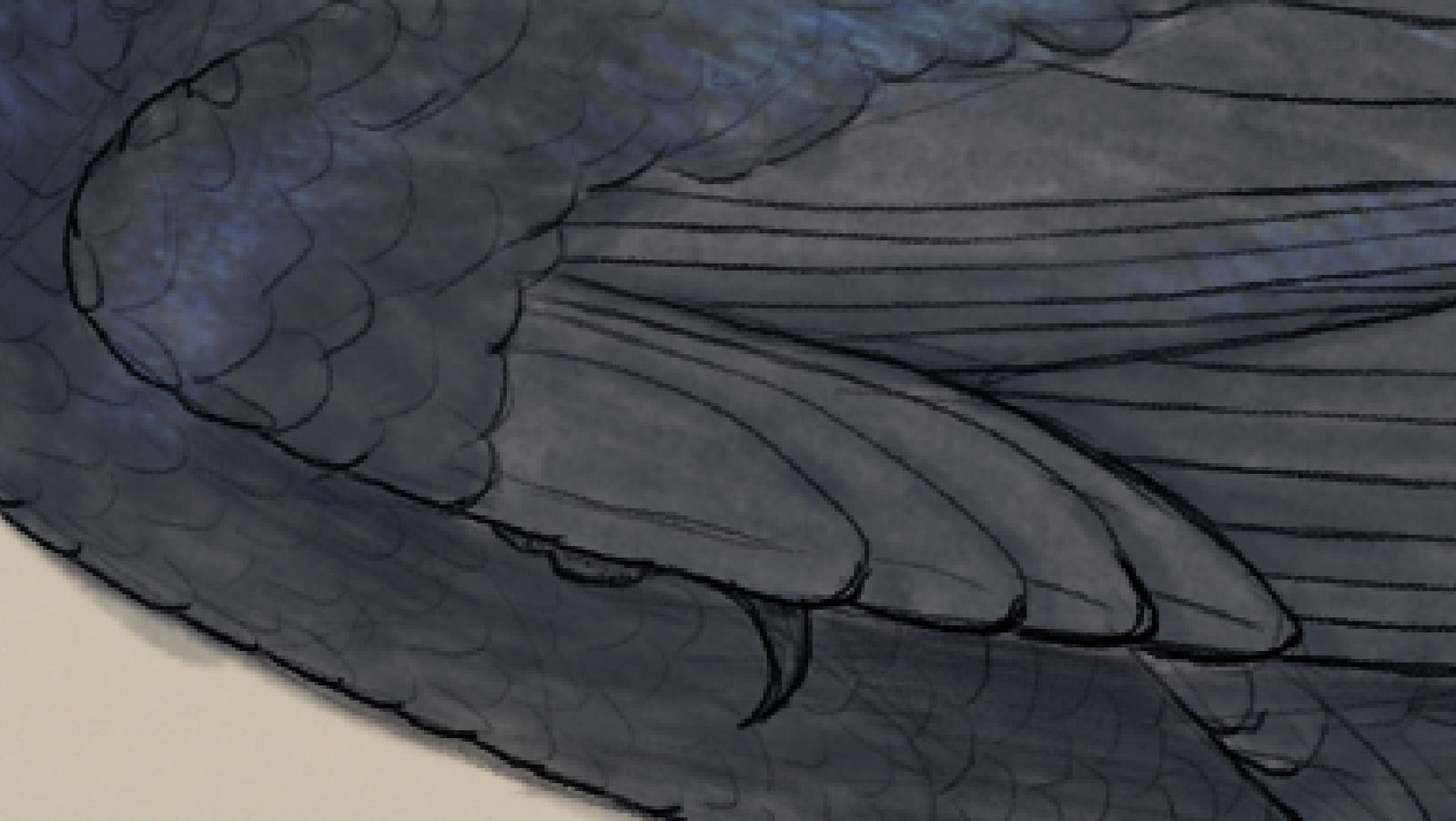
Fig. 11. Alula feathers based on specimen photos and Agnolin et al. (2019).
Creationist reconstructions need not shy from showing modern avian features like the alula just because some evolutionists believe it hadn’t evolved yet. Microraptor should be shown with alula feathers because they have been found on the fossil specimens and are an important part of a functioning wing.
Wing Folding
Carpenter (2002) provides a description of the complex motion of bird wing extension and flexion. It is a remarkable design that shows God’s handiwork in engineering.
Some claim that the dromaeosaur wrist could not fold like a bird’s does because of a lack of range of motion (Senter 2006; Sullivan et al. 2010). However, these estimates are performed with bone-on-bone manipulation. Range of motion is very difficult to predict accurately without soft tissue such as cartilage and ligaments. In smaller animals, such as Microraptor, even the dimensions of the bones are estimated to be inaccurate by between 10 and 30% due to non-preserved cartilage (Bonnan et al. 2010; Holliday et al. 2010). Paul (2002, 58) also discusses the effects of joint cartilage:
The hindlimbs of dino-avepods could be tightly folded. The ossified condyles of most dino-avepod astragalocalcaneums are not developed well enough to allow the ankle to collapse (unlike in mature birds), so cartilage caps probably formed large roller surfaces (as in immature birds).
As noted above, these cartilage caps also occur on immature bird forelimbs, so if this feature existed on extinct bird hindlimbs, then this lower degree of ossification was likely present on the forelimbs as well, as is seen in extant juvenile Gallus (personal observation).
Bird wing range of motion is particularly difficult to predict because the wrist swivel adduction is increased by the radius sliding distally during wing flexion and proximally during wing extension (fig. 12). This feature was likely present on dromaeosaurs as well (Gishlick 2001, Fig. 7). Some claim that the folding wing did not “evolve” until avialans, but this is difficult to prove. There appear to be differing opinions on which osteological features enable this auto-folding capability. It may be asymmetric distal condyles of the humerus (Paul 2002, fig. 4.2, Gishlick 2001), a trough-like notch on the distal end of the ulna (Carpenter 2002), shape of the radiale (Sullivan et al. 2010), asymmetry on the proximal end of the ulna (Motta et al. 2020), or some other feature or a combination of features. It is difficult to be conclusive about the presence or absence of this feature without accounting for soft tissue such as cartilage.
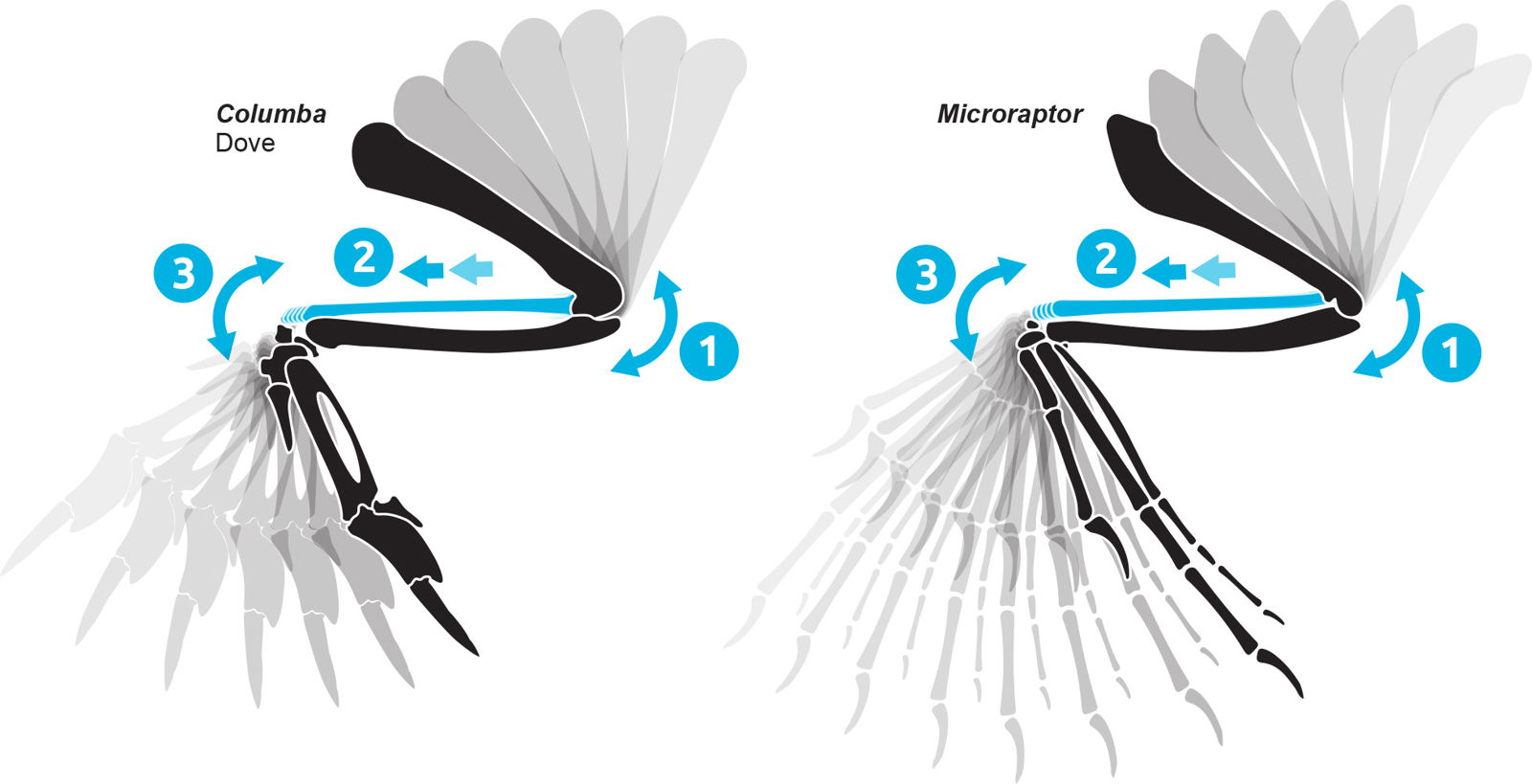
Fig. 12. Wing auto flexion/extension with sliding radius. When the elbow flexes (1), the radius is pushed distally sliding along the ulna (2), which makes the wrist auto-flex (3). Based on Carpenter (2002) and Gishlick (2001). Individuals not to scale.
However, Agnolin and Novas (2013, 52) confirm this feature in Microraptor:
As Vasquez (1992, 1994) pointed out, the modern avian wrist possesses the ability to synchronize flexion of extension of the elbow and wrist joints automatically. This kind of automatic mechanism of the wing is widely accepted (see Vasquez 1994) as an indispensable requirement for the powered and well-controlled flight seen in all modern birds. Vasquez (1994) indicates two main osteological features as indicative of automatic wing coordination: the presence of a groove at the distal-dorsal surface of ulna, and a well-developed extensor process on metacarpal I for the insertion of the M. extensor metacarpi radialis. The presence of a relatively well-developed extensor process on metacarpal I is corroborated in Sinornithosaurus, Microraptor, Anchiornis, and Archaeopteryx, suggesting that the presence of an automatic mechanism for flight may be traced back to the base of Averaptora.
There is also evidence of a propatagia, the soft tissue feature discussed previously, in Microraptor that helps make this system operational (see fig. 6). This is yet another indicator that Microraptor had a wing auto-folding mechanism similar to extant birds.
Extant birds can fold their wings to the degree that the manus is within the body contour (personal observation). While some extant birds fold their wings very tightly, this is often not required to hold the wing inside the body contour, especially once contour feathers are accounted for. Many evolutionary reconstructions show the wing hanging partway down and in front of the body or even held out sideways from the body. This not only looks unnatural, but it is unnecessary from the evidence. On the contrary, holding wings down in front of the body would cause the feathers to drag on the ground (fig. 13) which would cause the wing feathers to become soiled and damaged, reducing function.
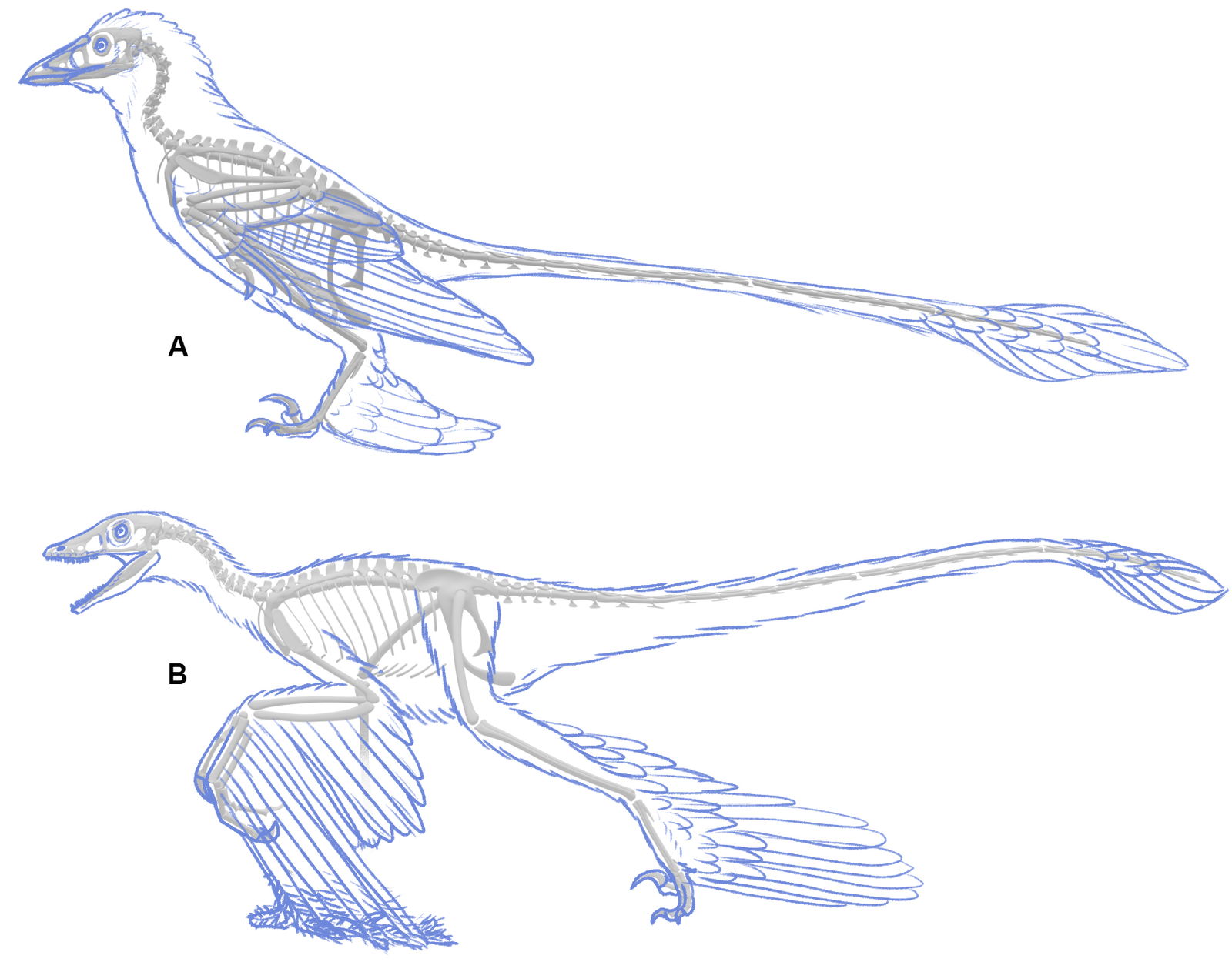
Fig. 13. (A) Microraptor forelimb folded as a bird wing is far more functional and consistent with the evidence. (B) But many Microraptor reconstructions hang the arms below or in front of the body. This would soil and damage the primary feathers. Also note that the large primary feathers attached to the middle digit cancel out any selective advantage from the large forelimbs by reducing grasping ability.
Feduccia (2020, 22) summarizes this problem when describing a reconstruction of the dromaeosaur Zhenyuanlong:
With little evidence [Zhenyuanlong] was reconstructed tearing through the Cretaceous forest with spread wings ready to rip the guts out of anything that it might find, so unlike modern terrestrial birds (e.g., turkey) that keep their wings folded tightly against the body to reduce drag.
This is merely an evolutionary trope that abuses artistic license to communicate an evolutionary idea. This is an example of how evolutionary presuppositions can lead to the idea of non-functional anatomical features. Creationists, however, know that God created a fully-functioning design and can do the work to discern how the design functioned in life.
The choice was made here to reconstruct Microraptor with wings in a resting position folded against the body. This is consistent with the evidence and with extant avian behavior. Creationists should be careful in their reconstructions to avoid the evolutionary view showing wings hanging below the body contour. Instead, wings at rest may be posed similar to modern birds, neatly folded against the body.
Wing Flapping
Most living birds (with the exception of some flightless variations) have the ability to flap their wings. This ability is obviously a prerequisite for flight and God equipped birds with specially engineered shoulders to support this.
Microraptor had a large quadrangular coracoid that articulated with the scapula to form an L-shape when viewed perpendicular to the body axis (Brusatte et al. 2014; Xu et al. 2017). The coracoid attached to the sternum (sometimes referred to as “sternal plates” when found unfused) which, along with the furcula, provided a reinforced shoulder joint to support wing flapping (fig. 14). This feature is not found in theropod dinosaurs whose coracoid is oval shaped and fused to the scapula, creating a continuous arc (Brusatte et al. 2014).
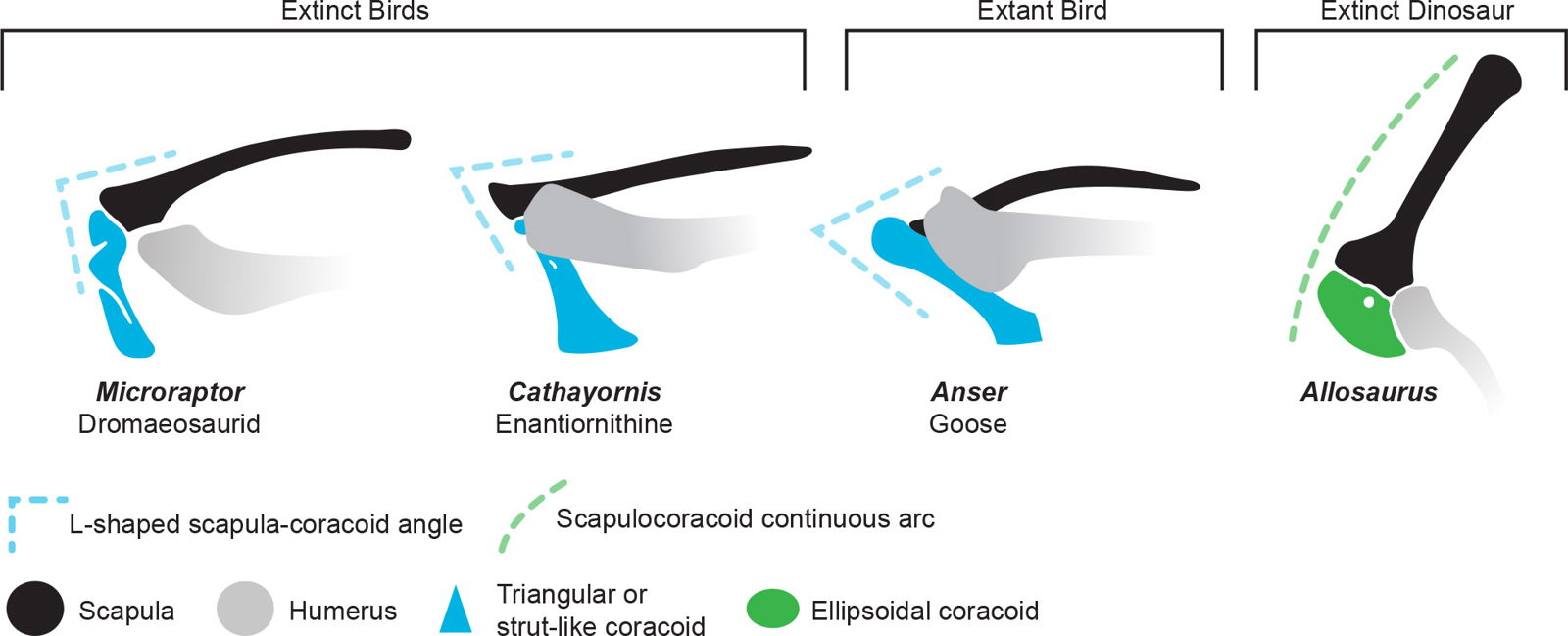
Fig. 14. Shoulder girdle of Microraptor contrasted with extinct bird (Cathayornis), extant bird (Anser), and a theropod (Allosaurus). Note the different shape and angle of the coracoid. Individuals not to scale. Anser from specimen photos, and others adapted from Hartman (n.d.) reconstructions.
This L-shaped scapula-coracoid joint of Microraptor is very similar to that of extant birds (Feduccia 2020). Extant flying birds often have a strongly angled scapula and coracoid (less than 90°) while flightless birds like ratites have a more obtuse scapulocoracoid angle. Chatterjee and Templin (2007) mention “a scapulocoracoid whose ends are oriented at an acute angle to each other” as an anatomical feature that suggests Microraptor could become airborne.
In addition to the sharp coracoid angle, the coracoid shape in Microraptor is elongated. These two features vary in modern birds and would be expected to vary in extinct birds as well.
Zheng et al. (2010, 215) observed the coracoid in the short-armed dromaeosaur Tianyuraptor. They state:
The significantly shortened forelimb of Tianyuraptor suggests a lack of aerodynamic function, which is further supported by the transversely wide coracoid (in microraptorines and birds, the coracoid is long along the axial direction) and the small furcula (in microraptorines and birds, the furcula is much more robust.
Evolutionists often associate these features with the supposed transition from a theropod dinosaur forearm to bird wing. However, in a biblical worldview these features can be explained as a functional design related to the flight ability of these birds. It appears that stronger flying birds have a sharper scapulocoracoid angle and elongated coracoid to provide a more stabilized wing for stronger flapping. In contrast, what an evolutionist would consider more “primitive” features may be explained by a wing that is more similar to extant flightless birds (fig. 15). These birds may have been created flightless or may have lost their flight ability over generations of adaptation. Change like this is a loss of information so is not considered evolution.
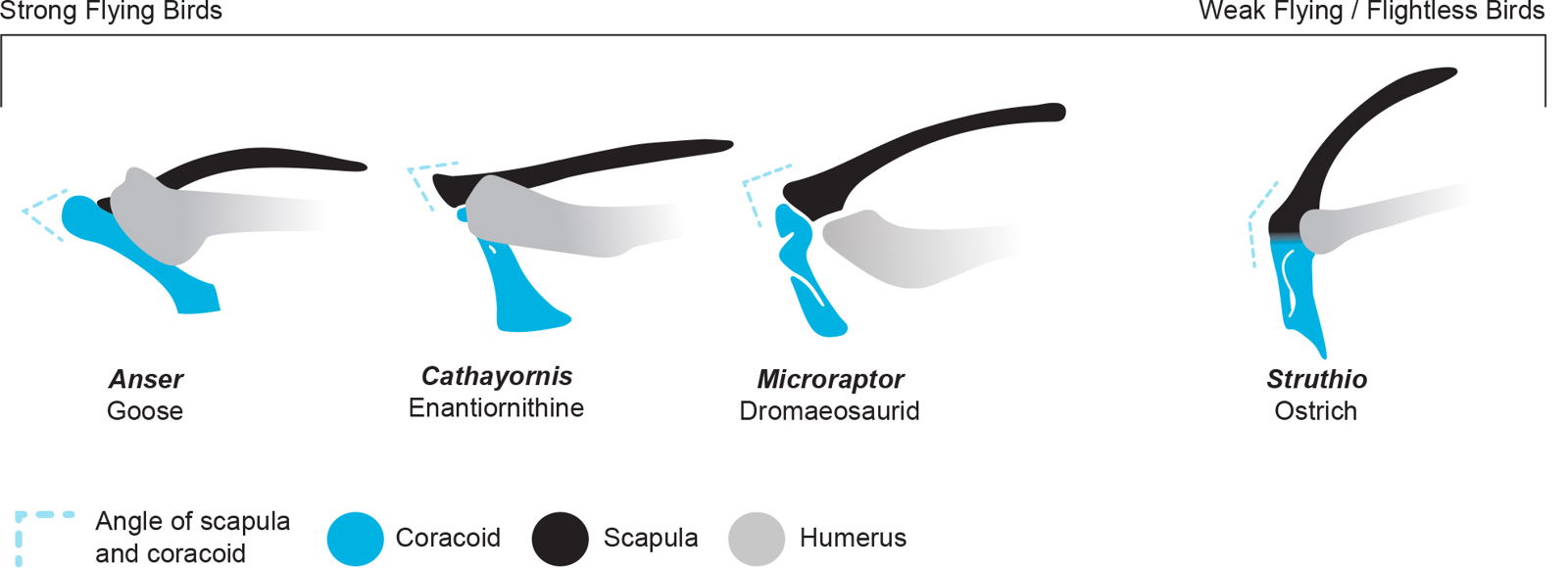
Fig. 15. Note that this diagram does not represent evolution over time. Most of these taxa likely belonged to separate created kinds. Instead, it illustrates the differences in shoulder designs that were engineered by God because of functional differences in the locomotion of these animals. Variation in coracoid and scapula angles appears to be related to flight ability in extinct and extant birds. Skeletal drawings adapted from Hartman (n.d.) or traced from specimen photos. Individuals not to scale.
The idea of anatomical differences that match varying flight ability also fits with what would be expected as a result of the global Flood. The stronger fliers would survive longer and be buried in higher rock layers, while the flightless and short-range fliers would be buried lower in the strata and earlier. Hence, we find bony-tailed birds in the Flood year, in Late Jurassic strata (Archaeopteryx).
From a creationist worldview, the tripod-like engineering created by the angles of the coracoid, scapula, and furcula create a reinforced shoulder joint that can handle the rigors of flapping. Flapping not only requires a reinforced shoulder, but also requires a shoulder joint that has enough range of motion for flapping to be productive.
The glenoid is the articular pocket formed by the scapula and coracoid where the proximal end of the humerus is seated fig. 16). The position, angle, and shape of the glenoid are part of what determines the range of motion for the forelimb.
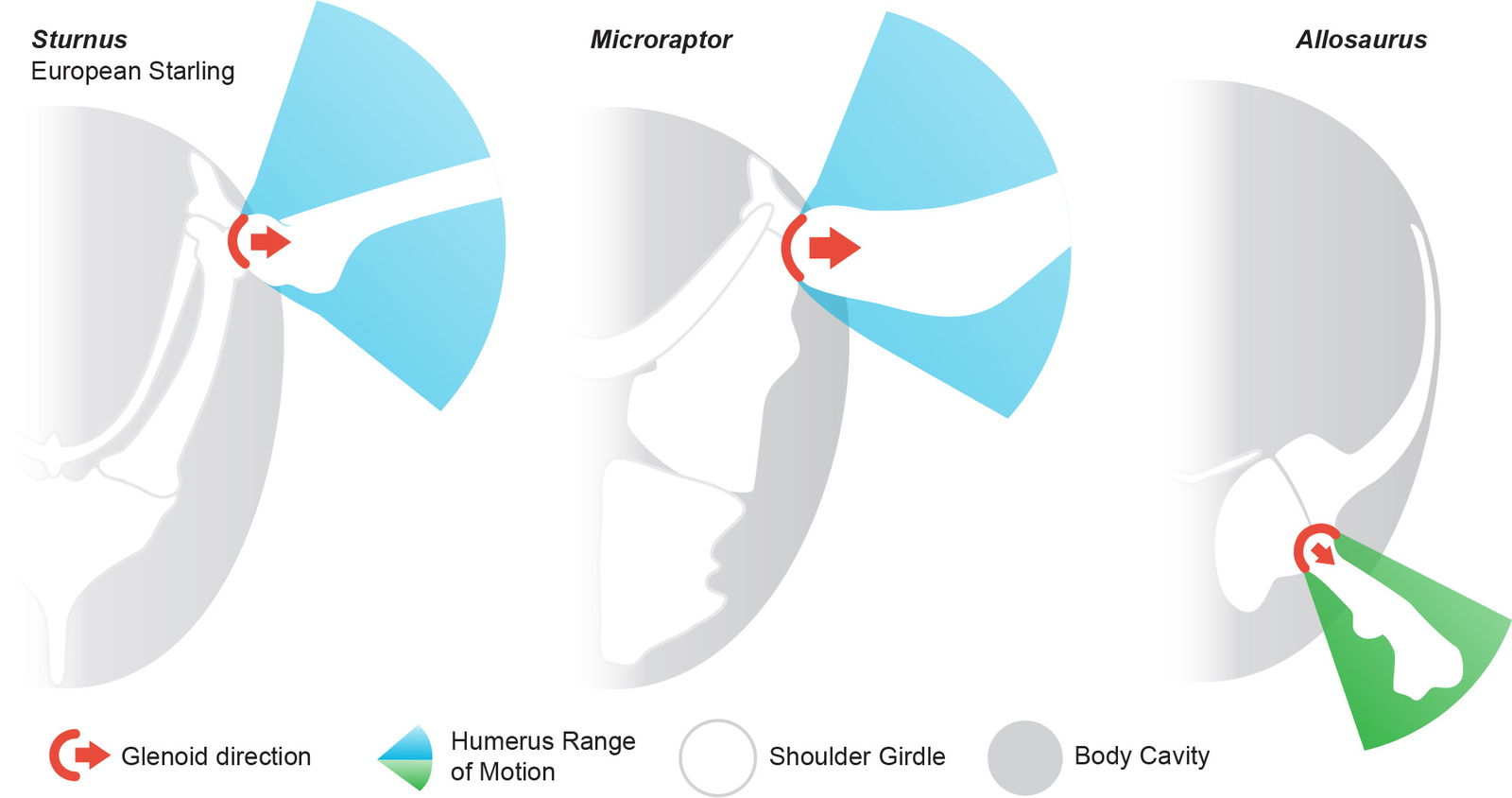
Fig. 16. Shoulder range of motion estimates for a Sturnus, Microraptor, and Allosaurus in cranial view. The standard theropod forelimb (exemplified by Allosaurus) has a downward facing glenoid and is significantly smaller and more limited in motion than that of Microraptor which has a lateral facing glenoid as in extant birds. Allosaurus skeletal and range of motion from Carpenter (2002). Sturnus skeletal and range of motion adapted from Senter (2006) and specimen photos. Microraptor skeletal adapted from Hartman (n.d.), and (O’Connor et al. 2019: specimen STM5-32) and range of motion from Paul (2002) and the dromaeosaurs Bambiraptor (Senter 2006) and Deinonychus (Gishlick 2001). Individuals scaled to same trunk size.
Chatterjee and Templin (2007) mention “a laterally facing glenoid for gentle dorsoventral movement of the wing” as an anatomical feature of Microraptor that suggests it could become airborne. Brusatte et al. (2014) records that a lateral facing glenoid is a character of chickens (Gallus), ducks (Anas), curassows (Crux), and is a shared trait with Microraptor. Chatterjee and Templin (2012) recognize in maniraptorans a “shoulder girdle showing the laterally facing glenoid for dorsoventral wing excursion.”
Despite Microraptor having the same character value for glenoid direction as birds, some evolutionists have claimed that dromaeosaurs had wings that could not elevate above a sub-horizontal position (Senter 2006). They validate this claim by positioning the scapula and coracoid lower on the trunk, at a transitional position between the common avian and dinosaurian condition (fig. 17). This reveals their presuppositions as they interpret these animals as transitional forms between dinosaurs and birds. This is also seen in previous conclusions by some (Carpenter 2002; Gishlick 2001; Senter 2006) who, lacking much of the rich evidence from Chinese feathered specimens that Xu et. al. had access to, tended to conflate dromaeosaur function and range of motion with that of larger animals which are clearly theropod dinosaurs.
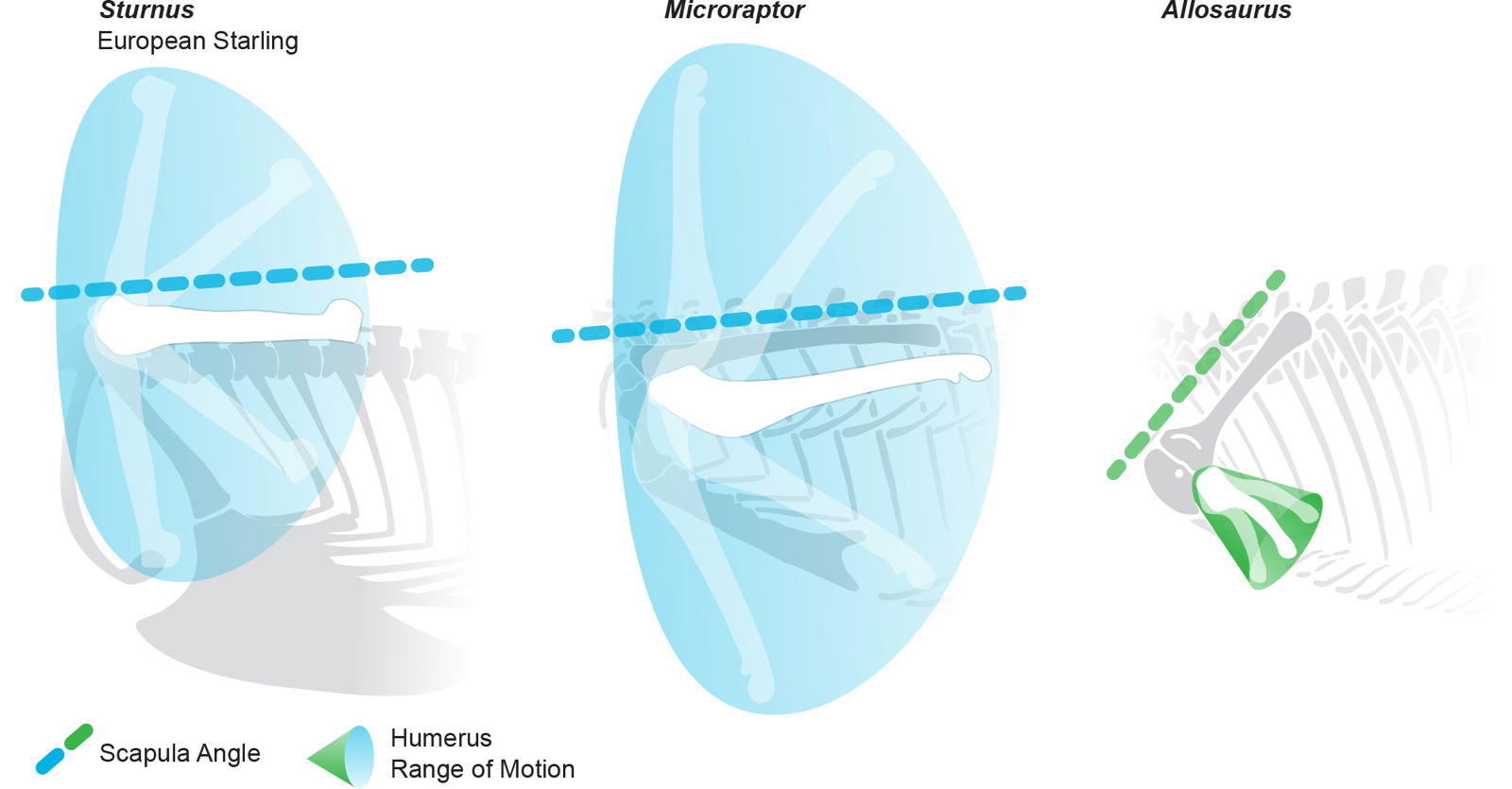
Fig. 17. Shoulder range of motion in lateral view for a Sturnus, Microraptor, and Allosaurus. Microraptor shared with the modern starling an orientation when at rest of its scapula that was parallel to the body axis. It also shared a dramatically larger range of motion than that inferred for Allosaurus. compared to the more angled scapula in Allosaurus. Sturnus and Microraptor skeletals adapted from Hartman (n.d.). Sturnus skeletal from specimen photos. Allosaurus range of motion from Carpenter (2002). Sturnus range of motion from Senter (2006). Microraptor range of motion from Paul (2002) and other dromaeosaurs Bambiraptor (Senter 2006) and Deinonychus (Gishlick 2001).
The exact shoulder position can be difficult to conclusively determine from fossil material. (Novas et al. 2020, 346) admit:
The location of the scapula on the thorax is still a matter of discussion among of early-diverging paravians (e.g., Senter, 2006) with a high degree of uncertainty.
Paul (2002, 126) says that “Gishlick seems to have underestimated the degree of action of the arm joints, especially the strong dorsal rotation of the humerus.” Paul also states that (page 47):
The pectoral girdle is set rather posteriorly in on the rib cage, with most of the scapula blade overlapping the chest ribs. This posterior position is in accord with and confirmed by neural anatomy and is the usual avian condition. In specimens in which the pectoral girdle complex is not properly articulated, the dislocation may have been caused by bloating of the carcass.
He goes on to explain that the postmortem bloating can cause the shoulder girdle to be displaced ventrally. He describes how the usual theropod dinosaur arrangement has a more vertical scapula, no sharp angle between the scapula and coracoid, and the scapula and coracoid face primarily sideways. He adds (page 49):
The great majority of researchers have assumed that the general tetrapod pectoral arrangement also applied to the scapulocoracoids of dromaeosaurs . . . This assumption is a major error—one that has obscured the phylogenetic position and flight heritage of these avepectoran [maniraptoran] dinosaurs—because they actually possessed fully avian shoulder girdles, as I was the first to explain and illustrate.
Agnolin and Novas (2013, 53) summarize the wing range of motion this way:
The lateral orientation of the scapular glenoid in unenlagiids (and probably also in other basal averaptorans), together with the absence of acute ridges delimitating the glenoid cavity, suggest that the humerus in these taxa was able to be elevated close to the vertical plane.
Baliga, Szabo, and Altshuler (2019) concluded that wing range of motion is even more strongly associated with flight behavior than wing shape is. If Microraptor’s shoulder girdle is positioned dorsally as in extant birds, the dorsoventral range of motion seems within the normal avian range, as seen in fig. 16.
The most recent objection to a birdlike shoulder girdle is related to the angle of the glenoid. Novas et al. (2021) claim that the wing in pennaraptors (dromaeosaurs, oviraptorids, troodontids, and avialans) flapped anterodorsally to posteroventrally, which according to them precludes powered flight. However, many who use this argument place the shoulder girdle angled ventrally as a transitional form between dinosaurs and birds instead of parallel to the dorsal vertebrae as seen in birds (fig. 17).
This objection also goes against other researchers’ findings. Burnham (2008, 38) says:
It is apparent from the skeletal construction of Microraptor that this skeletal anatomy was powered by a strong muscular system in the chest and upper arms. In fact, the body outline in the pectoral area and forelimbs far exceeds that of the pelvic area and hindlimbs. The pectoral girdle is built strongly and the shoulder socket was open with no prominent ridge surrounding the glenoid to restrict motions. The glenoid was positioned high on the back and forward. This places the center of gravity in a position giving the animal a high center of mass at the shoulders. The articular surface of the humeral head, allowed the arms to reach overhead. This allowed a range of motion for this animal to reach forward only with its lower arms. Similar to Bambiraptor, it could not adduct the humeri towards the midline of the body, but the lower arms could be adducted. The long forelimbs were also powerful as indicated by the large pectoral crest and large shaft diameter of the humerus.
Xu et al. (2017, 5) observe that:
Each scapula is preserved with its long axis angled at ~40° to the dorsal series as in most articulated specimens of non-avialan theropods. However, in articulated specimens of long-armed paravians, the scapula is nearly parallel to the dorsal series.
Certainly, the specific glenoid angle may impact the flight stroke direction. However, much more research likely needs to be done on extant bird glenoids to come to a solid conclusion on this. For example, Novas et al. (2020) compare the glenoid angle [of a Microraptor?] to a ratite and vulture. While both are birds, ratites are flightless, and vultures spend the majority of their time in flight. This lacks insight into the numerous bird species between those two extremes on the spectrum of flight ability. They were also unclear what specific angle the shoulder girdle was reconstructed at when measuring the glenoid angle against the vertebral column. Rather than speculating about the supposed evolution of flight, time would be better spent observing the measurable function of extant birds to compare detailed metrics of extinct animal glenoids in a broader study.
Sternum
The major flight muscles (M. pectoralis and M. supracoracoideus) in extant birds attach primarily to the sternum. In many extant flying birds, the sternum is keeled to increase surface area for the large muscles. While a keeled sternum is unique to birds, not all birds have one. Some may conclude that the supposed lack of a keeled sternum makes Microraptor less avian and more dinosaurian. However, ratites do not have a keeled sternum and they are clearly birds, therefore the keeled sternum appears to be a design feature associated with function, not a product of evolution or a requirement to be considered avian.
Even flying birds have significant variety in the mass of their primary flight muscle. Flight muscles in White Throated Rail only account for 7.8% of body mass as opposed to 36.7% in Cassin’s Dove (Degernes and Feduccia 2001).
The sternum is rarely preserved in fossil specimens. It is rare enough that Foth (2014) proposed that it may have been completely cartilaginous in some genera.
Carpenter (2002) observes that “in young Anas, the sternum is a pair of plates reminiscent of the sternal plates of some theropods, especially because the plates are relatively flat and lack a keel.” In his paper he shows the similarities of the sternum between young Anas and that of a dromaeosaur.
Bradley et al. (2019) record that Microraptor had two fused sternal elements, although Hwang et al. (2002) and Burnham (2008) document two separate sternal plates. Burnham (2008) states about microraptorine pectoral anatomy that “the sternum and furcula provide ample surface area for the attachment of pectoral musculature.” Chatterjee and Templin (2012, 602) agree, observing that Microraptor had:
a single, enlarged sternum for attachment of flight muscles, ossified sternal ribs and well developed uncinate processes for resisting compressive forces on the thoracic cavity imposed during downstroke.
A large muscle attachment area on the sternum is consistent with what Burnham (2008, 38) observed about the humerus:
The long forelimbs were also powerful as indicated by the large pectoral crest and large shaft diameter of the humerus.
It is possible that in addition to having a large sternum, Microraptor also possessed a cartilaginous keel. This is consistent with the condition seen in juvenile extant birds (see fig. 18) as noted by Feduccia (2020) and Carpenter (2002). This would enable an even larger attachment surface for the major flight muscles. Paul (2002, 47) stated:
The keels represent ossifications of the central sternal component (described in bird embryos by Fell 1939). It is therefore possible that a cartilaginous keel was present on incompletely ossified Dino-avepod [maniraptoran] sterna, including those of archaeopterygiforms. These keels were probably shallow although a deeper one cannot be ruled out in Archaeopteryx.
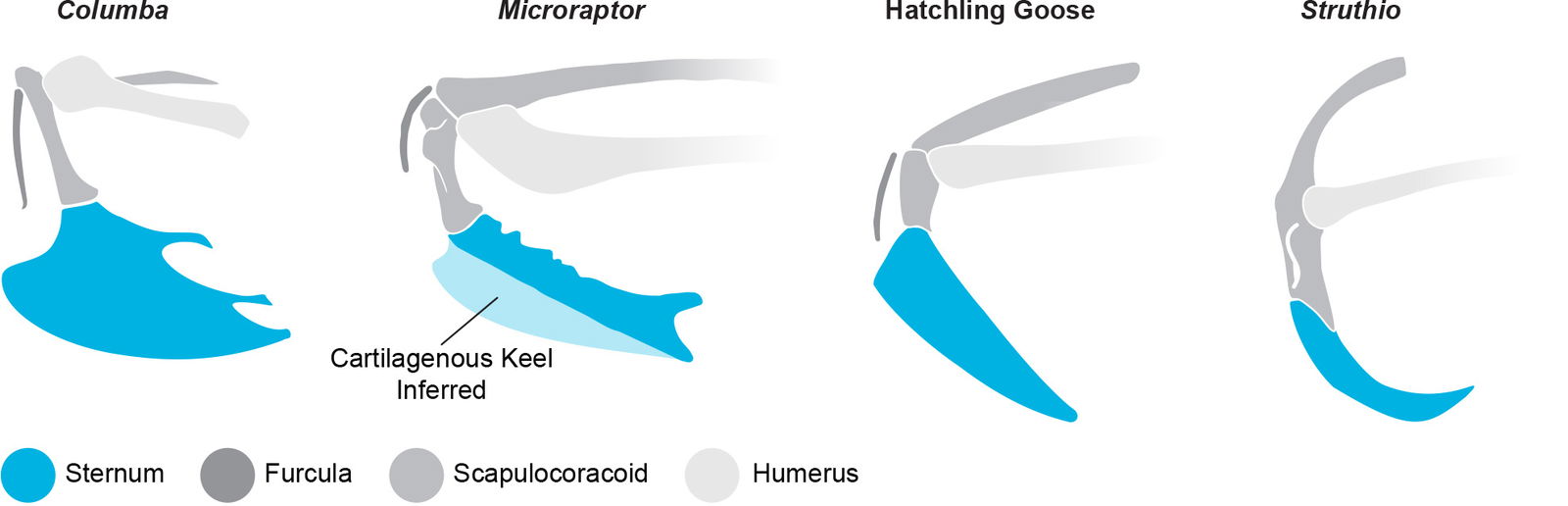
Fig. 18. Various avian sterna compared. Note the lack of sternal keel on Struthio and the hatchling goose, and the possible cartilaginous keel on Microraptor. Columba adapted from Bock (2013). Microraptor reconstruction adapted from Paul (2016). Hatchling Goose from Paul (2016) and Carpenter (2002). Struthio adapted from van Grouw (2013).
An ossified keel is more commonly found on birds such as enantiornithines that have more ossification and bone fusion in other areas of the skeleton as well, such as the synsacrum, tarsometatarus, and carpometacarpus. A possible explanation for this is an increase in ossification and bone fusion in the bird kinds found higher in the Flood strata as discussed above. Microraptor appears to have had many of the same functional features but expressed with less ossification and bone fusion in a sort of arrested development. Comparing the anatomy of a young extant bird to the fossilized remains of Microraptor reveal striking similarities such as the unfused carpometacarpus and lack of an ossified keel (see figs. 5 and 18).
Connected to the sternum is the rib cage. (Paul 2002, 46) notes about the trunk anatomy that:
Ossified uncinates and sternal ribs seem to be present in Microraptor . . . The sternal articulations of avepectoran sternal ribs also possess the hinge joint configuration observed in birds, which means that avian-style sternocostal joints were present.
The sternum and rib cage of Microraptor appears to be very similar to the range of normal characteristics seen within extant flying birds.
Supracoracoideus Muscle
Another anatomical feature unique to the avian wing flapping is the supracoracoideus muscle pulley system that aids in elevating the wing. Poore (2008, 279) summarizes this feature:
The muscle takes its origin from the sternal keel, and its tendon of insertion traverses through the triosseal canal (composed of the furcula, scapula, and coracoid) to its dorsal insertion on the humerus. The organization of this muscle allows for humeral rotation and elevation with muscle contraction.
Mayr (2017, 860) describes the function of this muscle:
The main function of this muscle is the elevation and supination of the wing during the upstroke and it is particularly well developed in birds which are capable of rapid takeoffs, such as tinamous and landfowl.
As early as the 1970s, some researchers concluded that “early” birds could not fly because they had not evolved a triosseal canal to enable the supracoracoideus muscle to power wing elevation (Poore, Sánchez-Haiman, and Goslow 1997; Walker 1972).
Even Feduccia came to this conclusion based on the evidence available at that time. However, he still defended the flight ability of Archaeopteryx (Olson and Feduccia 1979). Experiments were performed that transected the supracoracoideus in extant birds and it was discovered that flight ability was retained even without a functioning supracoracoideus (Degernes and Feduccia 2001).
This debate over flight ability has continued for 50 years, but much has been learned in that time. Novas et al. (2021) published an anatomical study on the paravian shoulder girdle. Here’s an excerpt from their conclusion (page 11, emphasis added):
We found that the m. supracoracoideus was much more developed in paravians more derived than troodontids, and that the existence of the tendon of m. supracoracoideus was probably diagnostic of eumaniraptorans.
The triosseal canal, as defined on the basis of bony contacts, was acquired in euornithine birds, but a foramen for the passage of the m. supracoracoideus was probably operative earlier (probably at the base of Pennaraptora, as shown by oviraptorosaurs with a cranially turned, pencil-like acromial process of the scapula) and bounded by bone (the acrocoracoid process), ligaments (the acrocoraco-acromial ligament bridging above the m. supracoracoideus), and eventually cartilage (the procoracoid process of the coracoid and the proximal end of the furcula).
This conclusion is fascinating because it seems to contradict all the conclusions about “primitive” shoulder girdle evolution. It appears that Microraptor and his kin had shoulder anatomy quite similar to extant birds, but with some slight modifications and less ossification. It is remarkable that over 160 years after Archaeopteryx was named, observations of this magnitude are still being made. Wang et al. (2022) came to a similar conclusion about the partially enclosed triossial canal. They even noted some similarities between “non-avialan pennaraptorans” and some extant birds such as paleognaths.
Two decades prior, Paul (2002) hypothesized that M. supracoracoideus looped over the acrocoracoid process to create the bird-like pulley system. However, he had the muscle originate from the coracoid instead of the sternum as in extant birds in an attempt to show a transitional form. The latest research contradicts this conclusion for Microraptor, but evolutionists still insist that, “in the course of avian evolution, the main origin of this muscle shifted from the coracoid onto the sternum” (Mayr 2017). So according to evolutionists, chance random processes and natural selection helped cause this shoulder muscle to shift, looping its tendon through a canal, creating a pulley system that reversed the function of the muscle and if that was not difficult enough, somehow moving the attachment point from the coracoid to the sternum. Novas et al. (2021) even admit that “explaining the reasons for such modifications is difficult.” They are essentially admitting that they have no idea what could cause this to happen, but apart from allowing a divine foot in the door, evolutionists are given no other choice.
Fig. 19 shows a modified version of Paul’s diagram to show the avian-style muscle attachment as Novas et al. (2021) concluded. It shows M. supracoracoideus partially attaching to a cartilaginous keel which would increase its attachment surface for more power, but this is not required for the muscle to function.
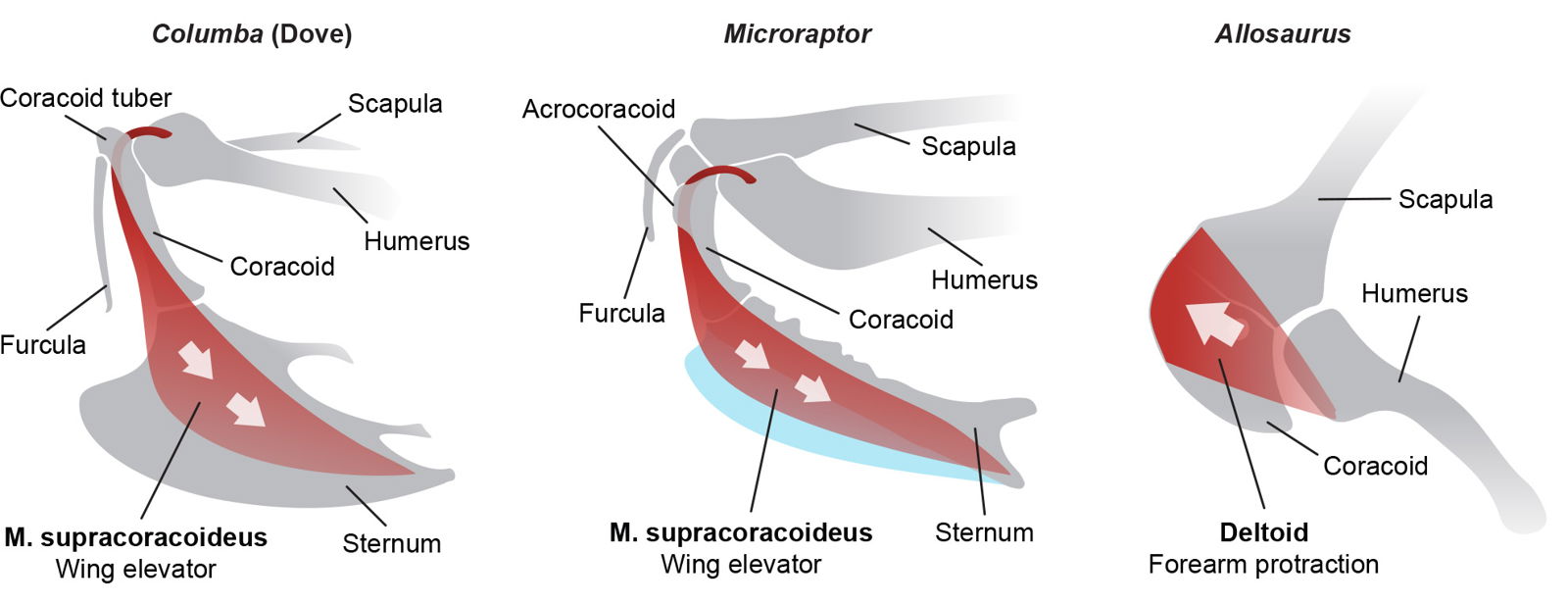
Fig. 19. The anatomy and function of the supracoracoideus muscle in Microraptor is very similar to modern birds like Columba (dove). Birds are the only living animals to have this pulley system to elevate the humerus. Allosaurus typifies the very different theropod dinosaur shoulder anatomy. Adapted from Novas et al. (2021), Paul (2002), O’Connor et al. (2015), Mayr (2017), and Bock (2013). Individuals not to scale.
The combination of wing folding, reinforced shoulder, lateral facing glenoid, supracoracoideus muscle, and strong pectoral musculature may have well given Microraptor the ability of powered flight. With so many factors it is difficult to estimate how strong Microraptor could fly, but it is clear that paleoartists can represent Microraptor doing more than merely gliding.
Hindlimbs and Tail
An avian reconstruction of Microraptor’s wings has been shown to be consistent with the evidence. However, the features and pose of the hindlimbs and tail are equally unique in extant avian kinds. Allen et al. (2013, 104) summarize this well when they state:
Locomotion in living birds (Neornithes) has two remarkable features: feather-assisted flight, and the use of unusually crouched hindlimbs for bipedal support and movement.
They also provide the following report from their study on locomotion (page 104, emphasis added):
we find evidence of an accelerated change within the clade Maniraptora (birds and their closest relatives, such as deinonychosaurs). In addition, whereas reduction of the tail is widely accepted to be the primary morphological factor correlated with centre-of-mass position and, hence, evolution of hindlimb posture, we instead find that enlargement of the pectoral limb and several associated trends have a much stronger influence. Intriguingly, our support for the onset of accelerated morpho-functional trends within Maniraptora is closely correlated with the evolution of flight. Because we find that the evolution of enlarged forelimbs is strongly linked, via whole-body centre of mass, to hindlimb function during terrestrial locomotion, we suggest that the evolution of avian flight is linked to anatomical novelties in the pelvic limb as well as the pectoral.
Birds walk with a unique posture that is very different from theropod dinosaurs. The following traits appear to work together to cause these animals to walk like birds:
- Pygostyle (fused or unfused): short, thin, stiff tail (caudal count ~30 or fewer)
- Large forelimbs (wings)
- Anterior center of mass
- Knee driven locomotion (subhorizontal femur)
- Three locomotor modules (forelimb, hindlimb, and tail operate independently)
- Small or absent fourth trochanter (attachment site for the M. caudofemoralis longus)
- Rear facing pubic bones
These traits work together as a single complex system of interdependent features, just as the wing features do. These traits also make a large difference when reconstructing these animals as to whether they look like birds. These traits are described in more detail below.
Over two decades ago, Hutchinson and Gatesy (2000) observed many of the changes listed above associated with Eumaniraptorans. The pubic reversal, femoral changes, tail changes, and hip changes noted would have required a large number of locomotor system changes at all at the same time. Evolution has no mechanism to produce this kind of irreducibly complex change.
Allen et al. (2013, 107) recognize the beautifully functional design relationship between various parts of the body when they state:
The proposed relationship between novel hip control mechanisms and more-crouched pelvic limbs, and the linkage proposed here between pectoral limb size, [center of mass] position and hindlimb posture, suggest that the evolution of both aerial and terrestrial locomotor anatomy were highly interconnected. Aerially adapted pectoral limbs and terrestrially adapted pelvic limbs belong to the same body, and the physical characteristics of one cannot logically be changed without affecting the mechanical functioning of the other. This reinforces the importance of whole-body biomechanical analysis in interpreting morpho-functional data from the fossil record.
This “whole-body biomechanical analysis” that Allen et. al. (2013) refer to is virtually absent from the processes that build phylogenies.
Unfused Pygostyle
Avian tail anatomy is unique. Most modern avialans have a pygostyle. A common definition of a pygostyle is a group of fused bones at the distal end of the caudal series. However, many fossil birds such as Microraptor have a series of caudal vertebrae which, while not fused, still function as a unit with the distal portions being stiffened by bone fusion, partial fusion, tendons, or enlarged prezygyphyses and haemal arches (fig. 20). Microraptor shows the latter two and evidence of stiffening in other taxa can be seen by the lack of tail curvature in many fossil specimens (personal observation).
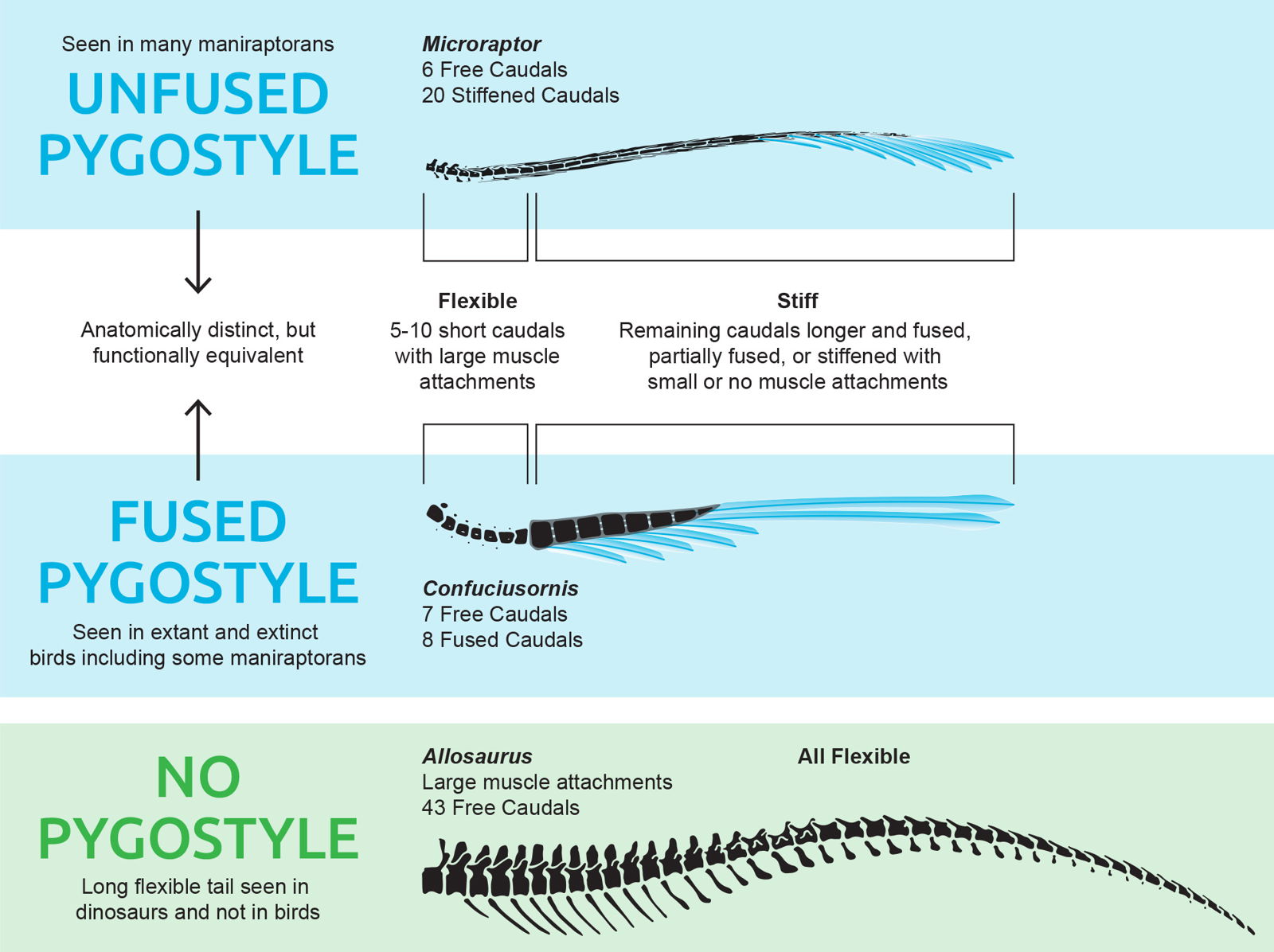
Fig. 20. The muscular Allosaurus dinosaur tail is of significantly greater length and thickness than the Microraptor tail. The avian tail bends most across the first 7 or so caudal vertebrae and remains relatively stiff across the distal caudals. Skeletal drawings adapted from Hartman (n.d.) reconstructions. Confuciusornis pygostyle fusion based on Rashid et al. (2018). Individuals scaled based on caudal count.
This means that, unlike in dinosaurs, the tail primarily flexed from the base. When seen in life, it would likely be hard to distinguish how a Microraptor tail differs from other birds which have long tail feathers attached to the pygostyle that flexes at the base of the tail. The term “unfused pygostyle” is used here to refer to this feature because of its functional equivalence with the fused pygostyle in extant birds (Haynes 2023).
Paul (2002, 45) states:
In dromaeosaurs, the articular surfaces of the proximal caudal zygapophyses are vertically oriented and the same appears to be true in Archaeopteryx. This adaptation allowed the tail to flex 90 degrees dorsally immediately behind the sacrum; this flexion is observed in some fully articulated tail bases.
In addition to the stiffening of the tail, Microraptor has a lower caudal count (24–26 caudals) than dinosaurs (30+ caudals). And the muscle attachments for movement of the tail exist almost completely on the first six caudals. Some paleoartists make the mistake when reconstructing Microraptor of giving it a thick, dinosaur-like tail. Even skeletal reconstructions often exaggerate the thickness of the tail for this animal. Viewing the actual fossil specimens makes it clear how thin Microraptor’s tail was. It appears more like a thin rod than a theropod tail (Supplementary fig. 2). It likely did have some flexibility in the distal areas as seen in some dromaeosaurs (Norell and Makovicky 1999), but likely less flexible than some of the larger dromaeosaurs (Hwang et al. 2002). Some flexibility is expected because God would have designed these animals with the ability to reach and preen their tail feathers. So, based on the scientific reasons presented above, paleoartists should represent Microraptor’s tail as thin and stiff.
Many extant bird tails have long feathers attached to a short pygostyle. Observing an extinct bird with short feathers attached to a long, unfused pygostyle in life would not appear that unusual since the silhouettes would not differ significantly from many extant birds.
The tail can be particularly challenging because it is often not completely preserved. Paleoartists need to carefully study the research to discover how much of the tail was preserved to represent it accurately. In the absence of fossil data, rather than using the phylogenetic tree, creationists should use other members of the same family or subfamily group as a best guess estimate, as the family likely includes animals of the same created kind (Lightner et. al. 2011).
Knee Walking
Extant birds have a center of mass well in front of the hip joint. To stay balanced on two legs their femur is held sub-horizontally to position the knee close to the center of mass (see fig. 21).
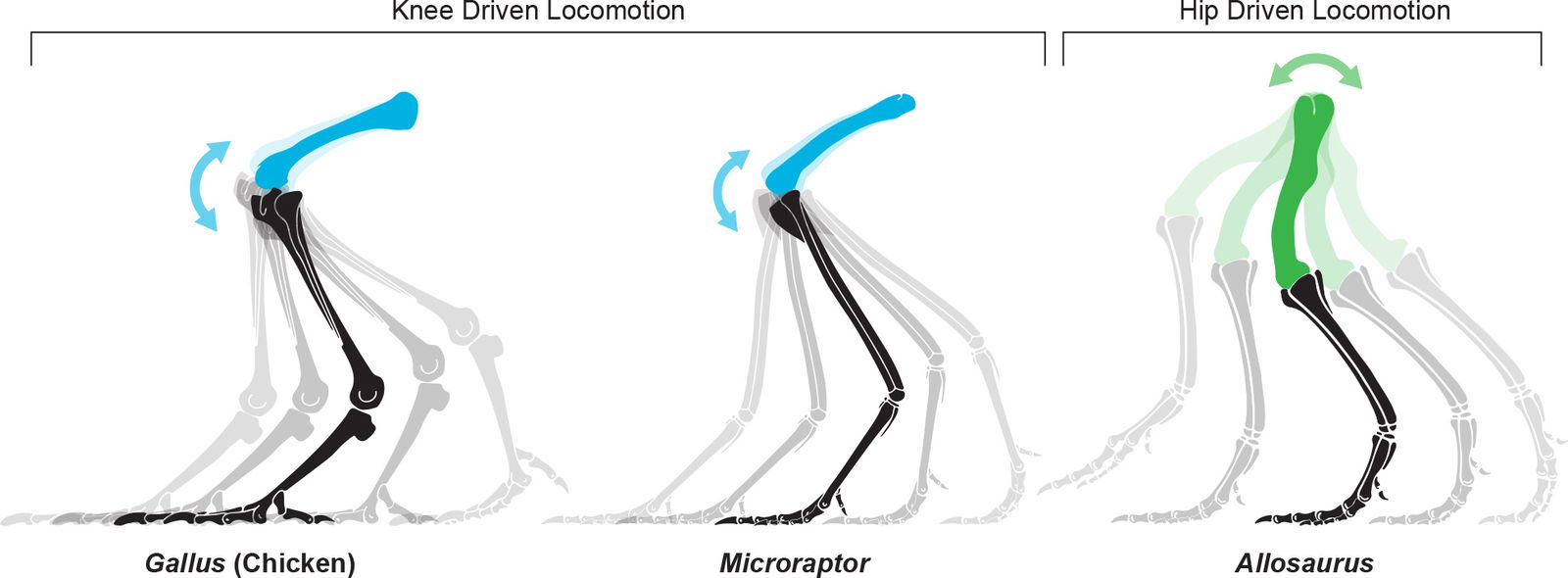
Fig. 21. Locomotion for Microraptor and Allosaurus based on the conclusion from Allen et al (2013). Skeletal reconstructions adapted from Hartman (n.d.). Individuals scaled to equal leg length.
Calculations of center of mass for extinct animals can vary widely based on assumptions and the amount of soft tissue which is often not preserved. Soft tissue presents so many unknowns that the center of mass calculations should be treated as tentative at best. For example, a Hadrosaurus with excellent soft tissue preservation was found in North Dakota and written about by National Geographic (Roach 2007). In that piece, Phillip Manning is reported as stating that they found 25% more soft tissue than expected in the “rear end” of the animal. This degree of difference would have a very large impact on center of mass calculations. Two years later Manning and others concluded that “any soft tissue reconstruction of an extinct taxon inevitably represents a best estimate model with an unknown level of accuracy” (Bates et al. 2009).
Another complicating factor is that smaller animals such as Microraptor are often preserved in a flat slab of rock, almost entirely two-dimensionally. This makes depth information very difficult to discern, inferred at best, and requires assumptions to complete mass calculations.
Evolutionists often associate the anterior shift in center of mass with the reduction in tail size. As shown above, Microraptor’s tail is significantly thinner, shorter, and lighter than in dinosaurs. In addition, a study by Allen et al. (2013, 106) came to a conclusion about the shift in center of mass that differs from the typical evolutionary idea:
Rather than being a phenomenon associated with or driven by tail reduction, we instead find that enlargement of the pectoral limb into the ‘raptorial’ forelimbs (and, ultimately, wings) of many eumaniraptorans is the strongest associated morphological trend. However, a more cranially biased pelvic limb CoM [center of mass] and perhaps increased head and neck mass were also involved. Note that this is also without considering the added mass of pectoral plumage (the geometry of which is too uncertain to model rigorously), particularly the large primary or primary-like feathers of Maniraptoriformes and later bird-line taxa, which would only strengthen the relationship of cranially shifted body CoM and pectoral mass.
The poorly known soft tissue in the pectoral girdle could also have a significant impact on the center of mass. As stated above, between 7.8% and 36.7% of extant bird body mass is in their pectoral muscles (Degernes and Feduccia 2001). Therefore, poorly known sternal elements such as a possible cartilaginous keel would increase pectoral muscle mass, shifting the center of balance even further forward.
Burnham (2008, 38) describes the center of mass in Microraptor specifically:
It is apparent from the skeletal construction of Microraptor that this skeletal anatomy was powered by a strong muscular system in the chest and upper arms. In fact, the body outline in the pectoral area and forelimbs far exceeds that of the pelvic area and hindlimbs. . . . The glenoid was positioned high on the back and forward. This places the center of gravity in a position giving the animal a high center of mass at the shoulders.
All the evidence points to Microraptor having a high, forward center of mass. This is consistent with living, volant birds so should be expected. This feature enables what some call “knee driven locomotion” or “knee walking.”
Hutchinson and Allen (2009) provide a helpful description of the differences between knee and hip driven locomotion. Dinosaurs use hip driven locomotion which is the “rotation of the entire pelvic limb with appreciable power input from large, extrinsic tail-based musculature.” Birds, on the other hand, use knee driven locomotion which is the “rotation of the elongate lower limb by flexors of the strongly flexed knee” (see fig. 21).
Hutchinson and Allen point out that the femur does have some movement in birds especially at higher running speeds, but still very little compared to that of dinosaurs.
Some assume that the femur needs to be short and thick as in ratites for knee driven walking. However, many extant birds have femur lengths similar to those of dromaeosaurs (Doube et al. 2012).
The fossil evidence makes it very difficult to conclusively determine the standing femur angle. Many researchers base the femur angle on the estimated center of mass which is of little help given its challenges discussed above. There is much room for interpretations to be influenced by presuppositions. Gatesy, Bäker, and Hutchinson (2009) concluded that “skeletal information alone has limited value for discerning mid-stance poses.”
Despite the challenges, specimens do preserve enough detail to make reasonable anatomical inferences. Hutchinson and Gatesy (2000, 747) recognized a difference in knee flexion in eumaniraptorans even before the explosion of new bird fossils in China. They stated that:
In these characteristics [Eumaniraptora] is more similar to extant birds than to [Dinosauromorpha]. Basal birds such as Archaeopteryx inherited this complement of features from [Eumaniraptora] relatively unchanged.
Christiansen and Bonde (2002, 360) state that:
Advanced non-avian theropods had evolved a set of advanced (that is, bird-like) characters relating to locomotion that were not present in plesiomorphic forms, and in advanced forms such as oviraptorosaurs, troodonts or dromaeosaurs many of the modifications towards an avian style of walking had already taken place.
Allen et al. (2013, 104) recognized the change in posture and state that this change was not a:
rapid transition from more-upright postures occurring around the base of Avialae. Our models explicitly yield the strongest support for a locomotor transition within the clade Maniraptora, and, perhaps more conservatively, Eumaniraptora (by which time the trend is well under way, in which considerable cranial [center of mass] migration and concomitant strong reduction in CFL mass occurred . . .)
Lack of Fourth Trochanter
Since dinosaurs are hip walkers, it makes sense that they would have large muscle attachments to enable moving their body weight by rotating the femur. Much of the large muscular tail discussed above contributes to these strong locomotory muscles.
The fourth trochanter on the femur of dinosaurs is the attachment site for the caudofemoralis longus (CFL), the primary locomotor muscle in dinosaurs (Persons and Currie 2011a, b; Cau and Serventi 2017).
In extant birds, however, the iliotibialis muscles are the most active upper leg muscles during hindlimb locomotion (Bishop et al. 2021). Extant birds have a very small M. caudofemoralis that acts primarily as one of the tail depressors as seen in fig. 22 (Fisher 1957; Harrison 1993). Birds do not have a large retractor for the femur because their knee-driven walking does not require it.
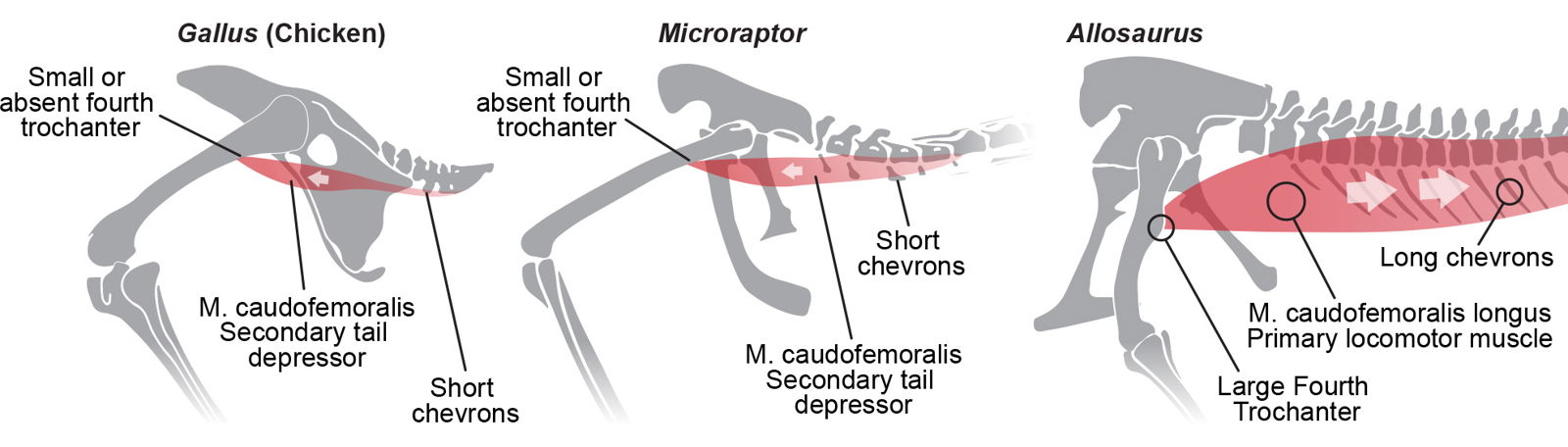
Fig. 22. CF on Gallus based on Harrison (1993), Moreno and Moller (1996), and Fisher (1957). Avian CF on Microraptor based on Allen et al. (2013) and lack of fourth trochanter from Brusatte et al. (2014). CFL on Allosaurus from Cau and Serventi (2017). Gallus, Microraptor, and Allosaurus skeletals adapted from Hartman (n.d.) reconstructions. Individuals not to scale.
Microraptor is observed to have an absent fourth trochanter (Brusatte et al. 2014; van der Reest and Currie 2017). Allen et al. (2013) corroborate this, stating, “the CFL and tail mass are strongly reduced from Eumaniraptora onwards.”
In the evolutionary worldview, the function of the CFL muscle is assumed to undergo dramatic changes. In dinosaurs the M. caudofemoralis anchors to a mostly stable tail and pulls the femur caudally as the primary means of locomotion. In birds this muscle is significantly smaller and functionally opposite, anchoring to a mostly stable femur and aiding in depressing the tail. This along with numerous other locomotor differences in the pelvic region are complex and dramatic functional differences that evolution has no undisputed mechanism to explain.
Evolutionists know this is a difficult transition to explain so they go to great lengths to make birds and dinosaurs appear similar. Grossi et al. (2014) did an experiment, raising chickens with extra weight attached to their rear end. The sensational claim was that chickens “walk like dinosaurs” if they had the weight of a long tail. However, a similar experiment was done by Carrano and Biewener (1999) and had the opposite results. In their experiment, the chicken’s femur became more horizontal, instead of more vertical. Experiments like this are just attempts to make birds look more like dinosaurs, so the idea of evolution can be told in a more sensational way.
Three Locomotor Modules
Decades ago, researchers recognized how different locomotion was between birds and dinosaurs. Gatesy and Middleton (1997) identified what they called three locomotor modules in birds (wings, legs, and tail) as opposed to one in theropod dinosaurs (leg + tail).
Dinosaur legs move primarily via large muscles attached to the tail, whereas bird legs are primarily moved by muscles attached to the hip and can move their tail independently (fig. 23). Evolutionists are still searching for a mechanism that would cause the dinosaurian leg+tail module to decouple into separate functional units.
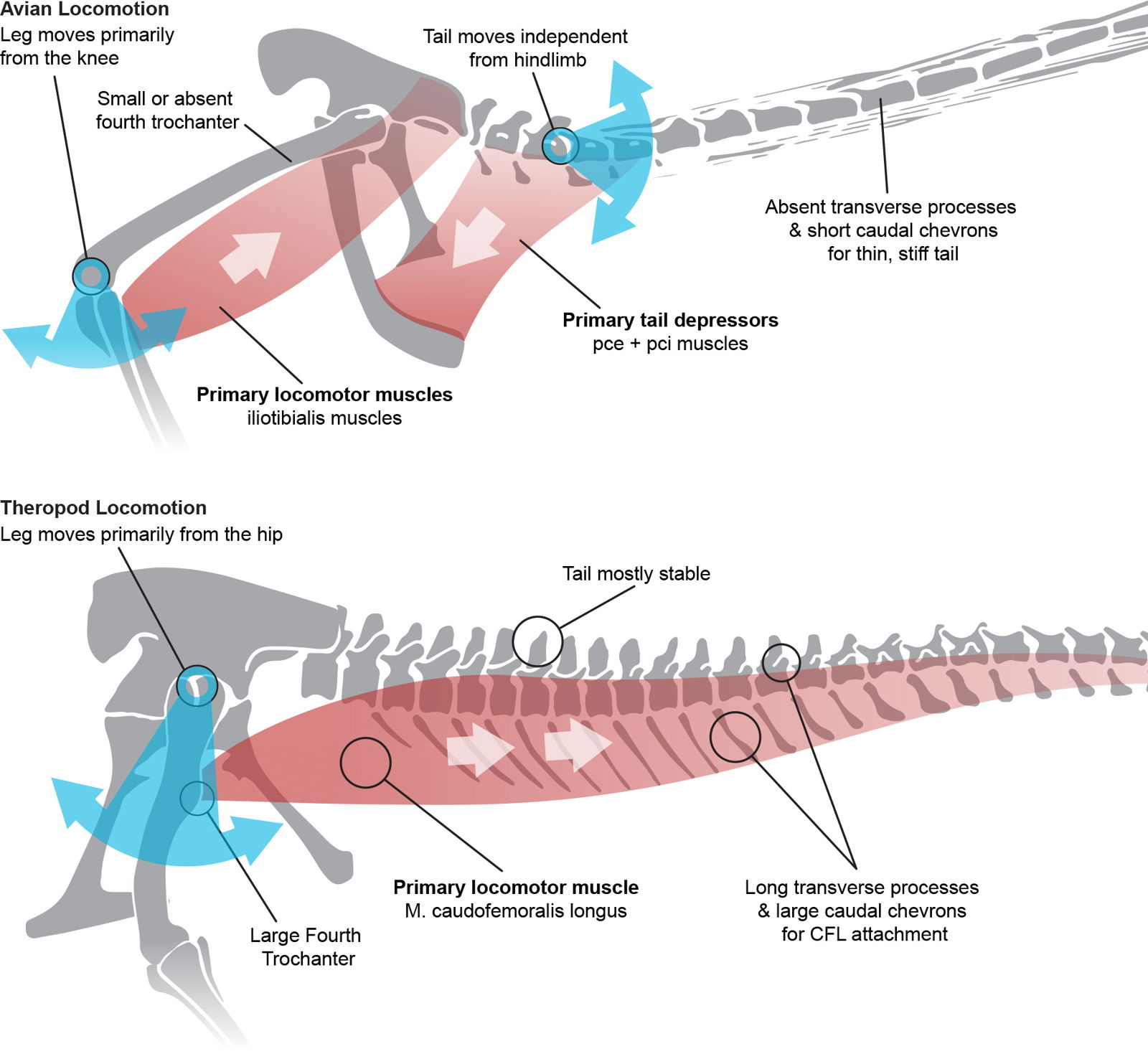
Fig. 23. Avian locomotion represented by Microraptor. Microraptor muscles inferred based on extant avian anatomy (Moreno and Moller 1996). Probable suprapubic muscles based on Ruben et al. (1997). PCI = m. pubocaudalis internus. PCE = M. pubocaudalis externus. Theropod locomotion represented by Allosaurus. m. caudofemoralis longus based on (Cau and Serventi 2017). Skeletal drawings adapted from Hartman (n.d.) reconstructions. Individuals not to scale.
Acetabulum Closure
Along with the significant differences between knee walking and hip walking one would expect to find some differences in the hip structure to support the differing movements. Some of these differences have been documented recently by Feduccia (2023, 5):
Among other modifications to the dinosaurian pelvis, femur, and axial skeleton, the combination of a vertically oriented femur with fully in-turned head articulating within a fully perforate acetabulum buttressed by a supracetabular crest allowed smooth pivoting of the hind limb about the hip joint, directly under the body, during the caudofemoralis-dominated excursion of the femur through the power and retracting strokes. It is a key morphofunctionally-integrated character complex in the “hip-driven” system of terrestrial locomotion.
The supraacetabular crest appears to be an important functional component in the theropod dinosaur hip structure and this feature is not present in birds. This difference is consistent with the idea that Microraptor walked like birds do as Allen et. al. (2013) postulate. In addition, the acetabulum of birds often has some amount of closure or medial occlusion (Feduccia 2023). This is seen in many extant and extinct birds, but not in dinosaurs (Supplementary fig. 4). Feduccia also documents that this closure is often made of thin bone or delicate soft tissue making it difficult to discern clearly in fossil specimens. But this is not the case in Microraptor which clearly has no supraacetabular crest and has clear medial occlusion of the acetabulum (Supplementary fig. 4).
Rear-facing Pubic Bones
Another obvious difference in the hip structure between birds and dinosaurs is the angle of the pubic bones as seen in fig. 24. Dinosaurs have forward-facing pubes while birds’ pubes are rear-facing.
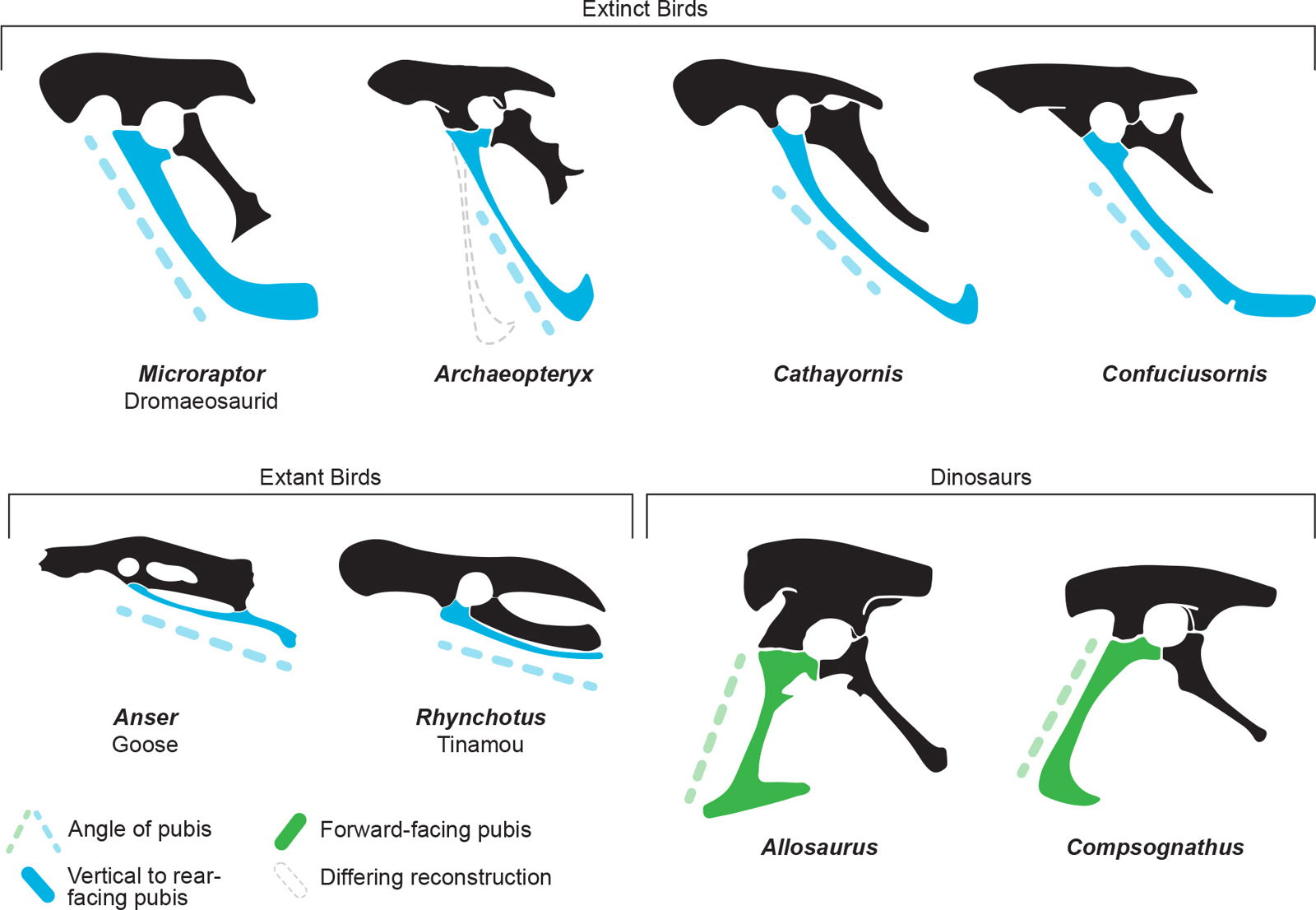
Fig. 24. Microraptor shows a departure in design from the typical theropod saurischian hip structure. Saurischian pubic bones point forward (propubic), but in birds the pubic bones point down or are fully retroverted (opisthopubic). Skeletal drawings adapted from Hartman (n.d.) reconstructions and specimen photos. Individuals scaled to similar pelvis size.
Ornithischian dinosaurs already have rear-facing pubic bones but most evolutionists claim that birds evolved from saurischian dinosaurs. This creates a problem for them because in addition to the numerous other changes, the pubes need to reverse their angle and to a great degree their function.
Allen et al. (2013, 106) state that:
Additional support for a locomotor transition within Eumaniraptora comes from the evolution of highly retroverted pubes, which, as previous studies have proposed, is likely to have fundamentally altered the moment arms (and, by inference, functions) of several major locomotor muscles.
Exact pubic angle can be challenging to determine in some taxa. Many extinct birds have less bone fusion than extant birds, so this can make pubic angle open to presuppositions when interpreting the evidence, especially in specimens that are not fully articulated. This is seen in the differing interpretations of the angle of the pubic bones between various Archaeopteryx reconstructions (Wellnhofer 2009).
Microraptor, as with other dromaeosaurs, was clearly opisthopubic. The similarity between the Microraptor pubis angle and that of other extinct birds can be seen in fig. 24.
The pelvic bones also house muscle attachments for moving the tail. The primary tail depressor muscles in extant birds are the M. pubocaudalis internus and M. pubocaudalis externus (Moreno and Moller 1996). Extant birds have thinner pubic bones than Microraptor which had more robust pubic bones. This design may have enabled Microraptor to have enough power to depress its long tail, an important aerodynamic function during flight.
As noted above, Microraptor had high caudal flexibility immediately after the sacrum, but the distal portions of the tail were less flexible. This is the opposite of the more reptilian dinosaur tails that have less post-sacral flexibility but are more flexible distally. As fig. 23 illustrates, this latter arrangement was integral to the theropod leg + tail locomotion system, as a stiffened proximal tail provided necessary strength to anchor its large caudofemoralis.
In reconstructions, Microraptor tails may be shown as having independent locomotive ability. Instead of showing the tail in line with the dorsal vertebrae, the paleoartist can make the animal more dynamic by tilting the tail up or down depending on what looks more natural. Paleoartists should use living birds as a reference for how they often position their tails.
In summary, the thin tail, forward center of mass, hip structure, muscle arrangement (inferred from the size of muscle insertion points like the trochanter shown in fig. 23), and locomotor modules are consistent with other avian features of Microraptor. Other than folded wings, these aspects are the most important in accurately representing the avian posture of Microraptor in reconstructions. Many evolutionary paleoartists draw the hindlimbs of Microraptor similar to a dinosaur’s leg with the femur vertical and the thigh and knee fully visible. But this is contradictory to the conclusions by Allen et al. (2013). Microraptor looks much more natural with the knee tucked up in the body contour, under the wing, hidden from view as in extant birds.
Hindlimb “Wings”
There have been multiple Microraptor specimens found with clear evidence of long pennaceous feathers on the hindlimb. These feathers appear to be attached to the metatarsal and extend caudally. Xu et al. (2003, 338) describe these hindlimb feathers:
In general, the leg feathers are arranged in a pattern similar to wing feathers in modern birds, suggesting the presence of a hindlimb wing. Although there is no modern analogue, our observations are concordant with some early hypotheses that there is a tetrapteryx stage in bird evolution.
Burnham (2008) reiterates this incorrect conclusion about hindlimb feathers having no modern analog when he states, “but the hindlimb wings are unusual and are not represented in any known modern analog.” To the contrary, there are several well-known birds with varying amounts of hindlimb feathers including snowy owls, and several breeds of chickens and pigeons. Van Grouw (2018) documents “vulture hocks” on chickens, and the “muffed” feet on pigeons (fig. 25).
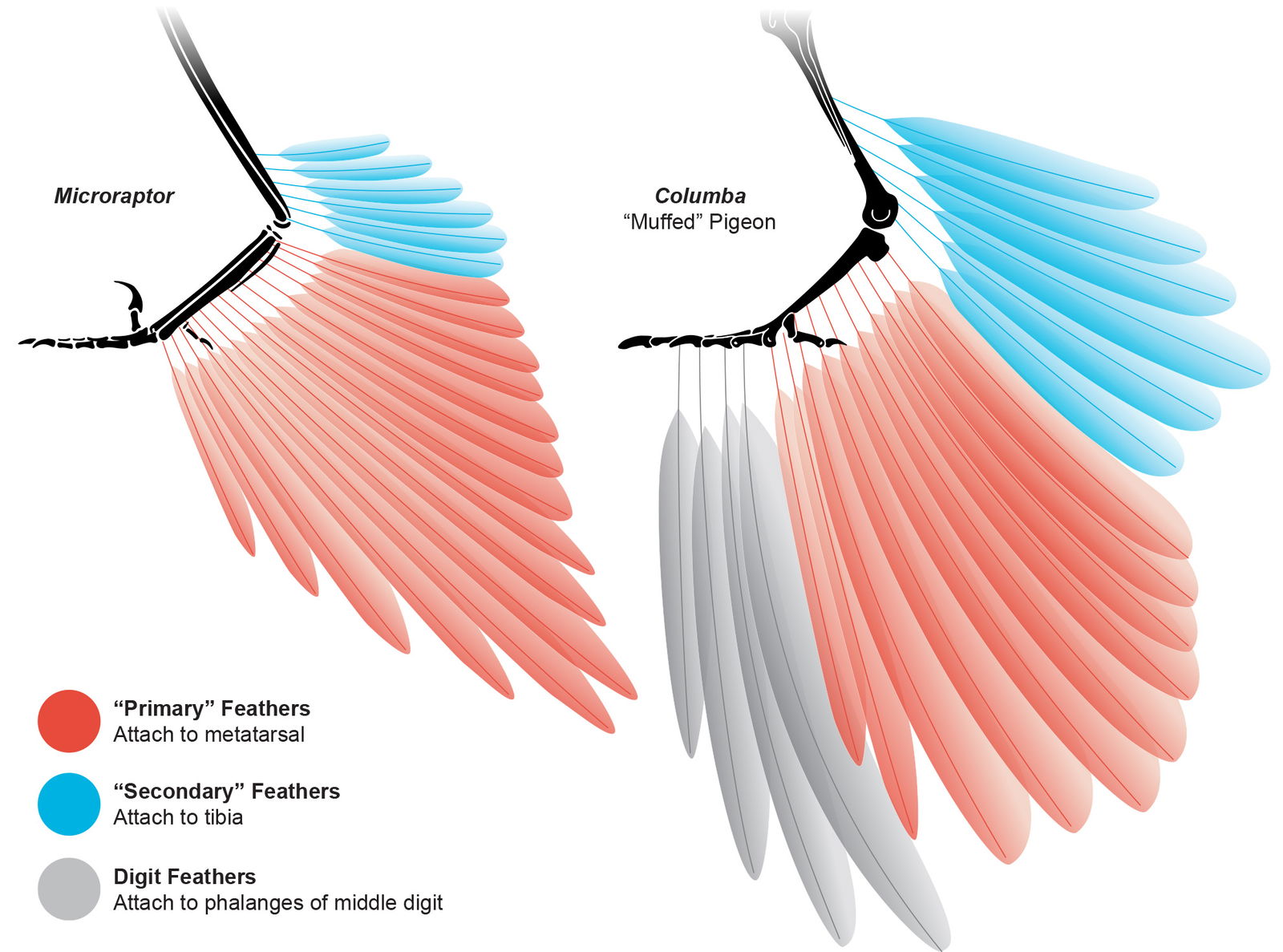
Fig. 25. Hindlimb feathers on Microraptor and Columba. Microraptor feathers adapted from Li et al. (2012) and Columba feathers adapted from van Grouw (2018). Individuals scaled to equal metatarsal length. Skeletal drawings adapted from Hartman (n.d.) reconstructions.
Instead of searching for living analogs, some evolutionists jump to conclusions about flapping hindlimbs and “tetrapteryx” modes of flight (Chatterjee and Templin 2007; Alexander et al. 2010; Dyke et al. 2013). They seem fascinated by this possibility as if evolution were somehow experimenting with the end goal of flight in mind (a reification fallacy). Despite these claims, others have concluded that Microraptor’s sprawled hindlimb model was based on poor data (Brougham and Brusatte 2010), and its range of motion would not support sprawling or flapping hindlimbs (Manafzadeh and Padian 2018).
Certainly, God may have designed Microraptor’s hindlimb feathers to contribute to its agility in flight, but comparing with similar existing birds will likely be the most informative approach, as argued above. A sprawling hindlimb would diverge from extant birds which, as discussed, use soft tissue anatomy to keep their knees tucked in a crouched position and so maintain balance while walking. This remains true for extant birds that have foot feathers also known as ptilopody. Bortoluzzi et al. (2020, 2472) describe these feathers:
Our findings indicate that in chicken and pigeon ptilopody is determined by the same set of genes that affect similar molecular pathways. . . . Feet of some birds display short and tight feathers on the metatarsus and digits, which in some cases appear like long flight-like feathers.
Domyan et al. (2016) also document the genetic mutation that causes the muffed feathers. They describe it as a “partial transformation from hindlimb to forelimb identity” that even creates asymmetrical feathers on the bird’s feet. Another interesting change with this mutation is that it causes the fibula to extend the full length of the tibia. This trait matches that of many extinct birds which do not have the partial fibula that many extant birds have.
As shown in fig. 26, some muffed pigeons develop hindlimb feathers that are larger than those on Microraptor. The pigeons also have feathers attached to the phalanges of their middle pedal digit while Microraptor appears to not have digit feathers. These feather-free toes would likely have been better for running and grasping prey.
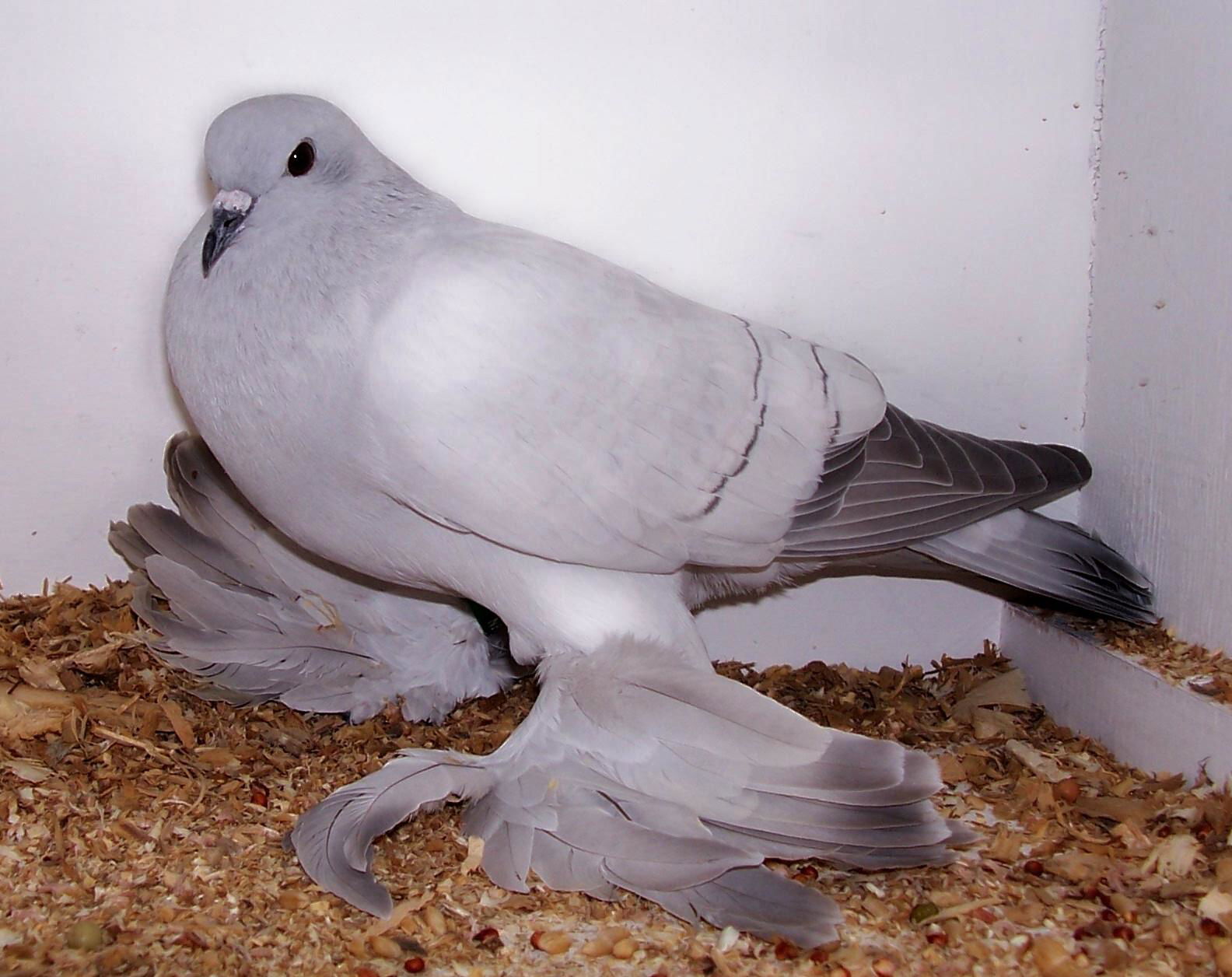
Fig. 26. Champion Ice Pigeon at Queensland State Show 2008 (Australia). Ptilopody (feathered “muffed” feet) is caused by a mutation that creates asymmetric flight feathers on the pigeon’s hindlimb very similar to those found in Microraptor. Graham Manning. “Champion Ice Pigeon at Queensland State Show 2008 (Australia). This bird is an old hen owned by Haden Walsh,” https://commons.wikimedia.org/wiki/File:Ice_Pigeon.jpg. CC BY-SA 3.0.
Feduccia (2023) observes that Microraptor’s hip socket may have allowed for medial excursion of the femur to enable the hindlimb to add to gliding efficiency. Feduccia has long been a proponent of the “trees down” hypothesis of the evolution of flight so his conclusion of gliding ability is not surprising. The gliding hindlimb model is difficult to prove or disprove without further evidence of the soft tissue.
Zheng et al. (2013) document that hindlimb feathers were quite common in fossil birds. It appears that flight feathers on the hindlimb of birds is either a trait that was part of God’s original creation with some aerodynamic or decorative purpose. It is part of the great variety we see in both extinct and extant birds.
Head and Neck
Teeth and Beak
Louchart and Viriot (2011, 663) document that:
Although all modern birds are toothless, most of the Mesozoic representatives were toothed, with various tooth shapes associated with diverse diets, showing that dentition reduction occurred after the differentiation of birds.
Despite extant birds being toothless, they do have some functionally similar structures. According to Louchart and Viriot (2011), dozens of bird families have serrations in their beak edge. Many baby birds also have an “egg tooth” (caruncle) which is an extension of the beak material (Clark 1961). This pseudo-teeth pattern is even more pronounced in some extinct birds, such as members of Odontopterygiformes which have large bony projections from the jaw bones that were likely covered with beak material (rhamphotheca). Louchart and Viviot (2011, 671) describe the function of these serrations:
Serrations represent very small, functional ‘teeth analogs’, efficient for grasping and holding, in numerous different lineages. Pseudo-teeth and odontoids are more enigmatic teeth analogs.
The design of true teeth is clearly a different design pattern by an all-wise Creator to provide similar functionality as a serrated beak. As Louchart and Viviot (2011) observed above, numerous extinct animals that are avian by consensus have true teeth (fig. 27). Enantiornithines, which are considered to be birds, have well defined teeth in the top and bottom of the mandible: Sapeornis, Cathayornis.
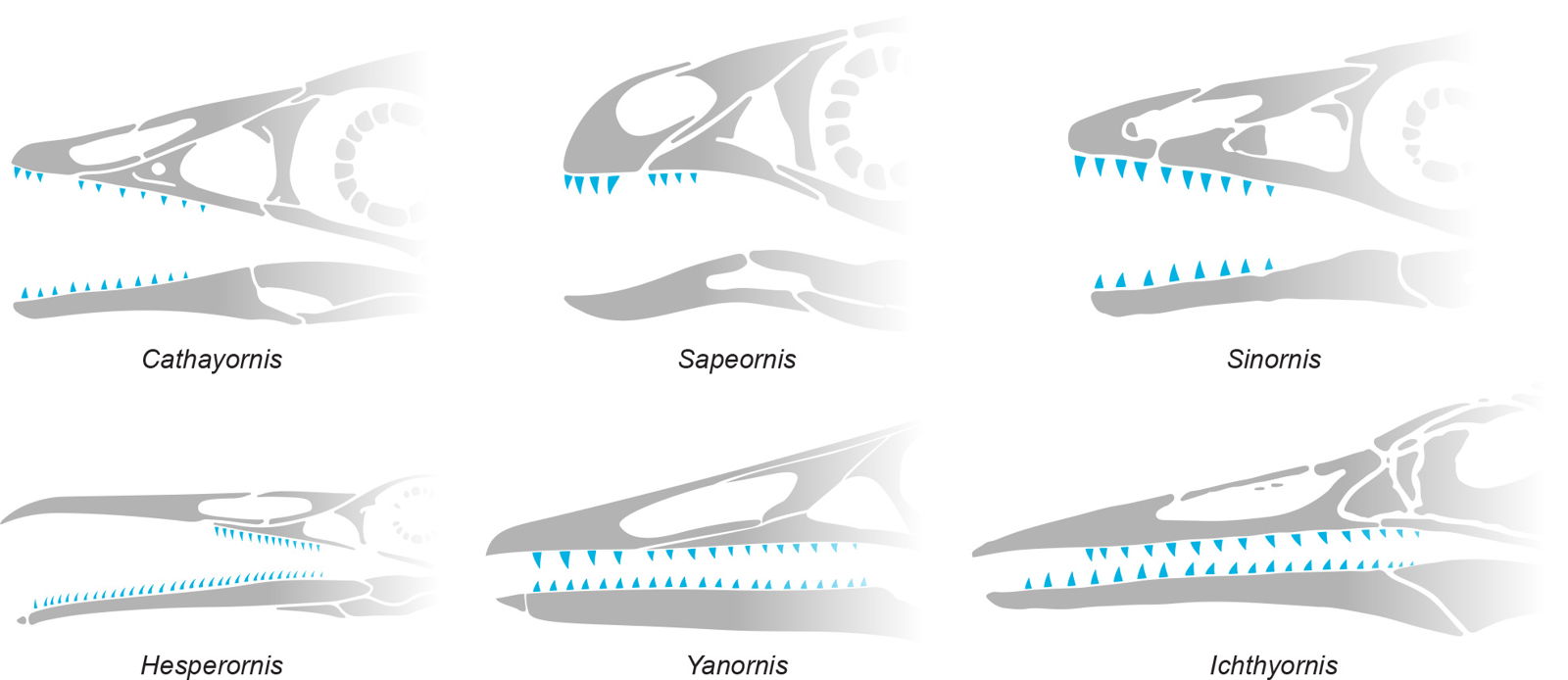
Fig. 27. Teeth are quite common in extinct birds, including Cathayornis, Sapeornis, Sinornis, Hesperornis, Yanornis, Ichthiornis, and others. Cathayornis and Sapeornis adapted from Hartman (n.d.) reconstructions and Wang et al. (2017), Sinornis adapted from Chiappe and Witmer (2002), Hesperornis adapted from Everhart (2011) Yanornis and Ichthyornis adapted from Field et al. (2018). Individuals scaled to similar snout length.
The observation of toothed and toothless forms within Aves signals no clear phylogeny, since extant and extinct Mammalia, Reptilia, and even the dicynodont therapsids also include both toothed and edentulous members. Teeth appear to be part of God’s incredibly diverse design for bird kinds.
As with wing claws, paleoartists often exaggerate the size and visibility of sharp teeth. Microraptor’s teeth were relatively small and when gum, lip, and beak tissue is accounted for would likely have been hidden unless the mouth was open.
Some evolutionists claim that Microraptor did not have a beak because no beak material has yet been found associated with a dromaeosaur. But another motivation for this conclusion is because their idea requires a mechanism for how the beak evolved and replaced the teeth in more “advanced” modern birds.
A bird’s beak is a marvelous, multi-functional, irreducibly complex design. It is used for preening, diet, display, protection, predation, sensory information, and more. Hieronymus and Witmer (2010, 590) describe it this way:
Rhamphothecae, the horny (keratinous) sheaths that cover the jaws in birds, show amazing diversity and provide some of the most compelling and easily appreciated examples of morphological adaptation in vertebrates, such as forceps for probing in sandpipers, filters in ducks and flamingos, “teeth” for gripping fish in mergansers and gannets, and nutcrackers in hawfinches.
According to (Button 2018), “Within Archosauria alone, beaks are hypothesized to have independently arisen a minimum of fifteen times,” and beaks supposedly evolved independently at least three times in birds (Knutsen 2007). Evolutionists come to this conclusion because of the phylogenetic tree. If an animal’s supposed ancestor did not appear to have a beak, then it must have evolved again. The phylogeny also leads to the conclusion that teeth and beaks could not have existed on the same area of the jaw. Their idea of beaks replacing teeth through evolution is a presupposition that affects interpretations and reconstructions.
Proving the absence of a soft tissue feature from the fossil record is very difficult. Zheng et al. (2020) admit that “very little is known about this feature in extinct organisms.” This is especially true with something as rarely preserved as the beak (rhamphotheca tissue). Confuciusornis is one of the most common fossil birds with over 600 specimens to date. Zheng et. al. (2020) document that only two of those 600 specimens (0.33%) show preserved beak material. And those that do have preserved beak material do not appear to show a pattern of why preservation occurred.
Confuciusornis has been called the earliest-known beaked bird (Feduccia 2020). However, Wang et al. (2017) document a rhamphotheca in oviraptorids, enantiornithines, and possibly jeholornithids, all of which have representatives with teeth.
Button (2018) does some interesting work in his dissertation. His work appears to confirm the idea that foramina (“porosity”) in the premaxilla is a likely indicator of the presence of a beak at least in extant birds (all of which have beaks). However, he relies heavily on phylogeny as a presupposition and confirmation of his conclusions. In the end, his model predicts that only five out of 40 extinct crown birds had beaks. His model also predicts that Confuciusornis had no rhamphotheca despite beak remains being found on multiple specimens. This shows how speculative and subject to interpretation this anatomical feature is.
Because of this, paleoartists often decide how much beak material to represent on a drawing by where an animal is on the phylogenetic tree. This can be seen by the “protobeaks” often shown on dromaeosaurs (Paul 2002; Prehistoric Planet 2022).
The idea that Microraptor did not have a beak relies heavily on the fallacious assumption that the absence of evidence is the same as the evidence of absence. But this assumption is not a conclusion based on the evidence.
Instead, the approach taken here is to evaluate the data based on a biblical worldview that opposes the notion of beak evolution. The logical process used is as follows:
- All extant birds have beaks.
- Birds use their beaks to preen and care for their feathers.
- Beaks are, therefore, part of the functional flight wing/feather system in extant birds.
- Extinct birds with flight feathers likely had a beak or something beak-like to care for their feathers.
- Microraptor is a bird with feathers.
- Therefore, Microraptor most likely had some amount of beak material (fig. 28) to care for its feathers.
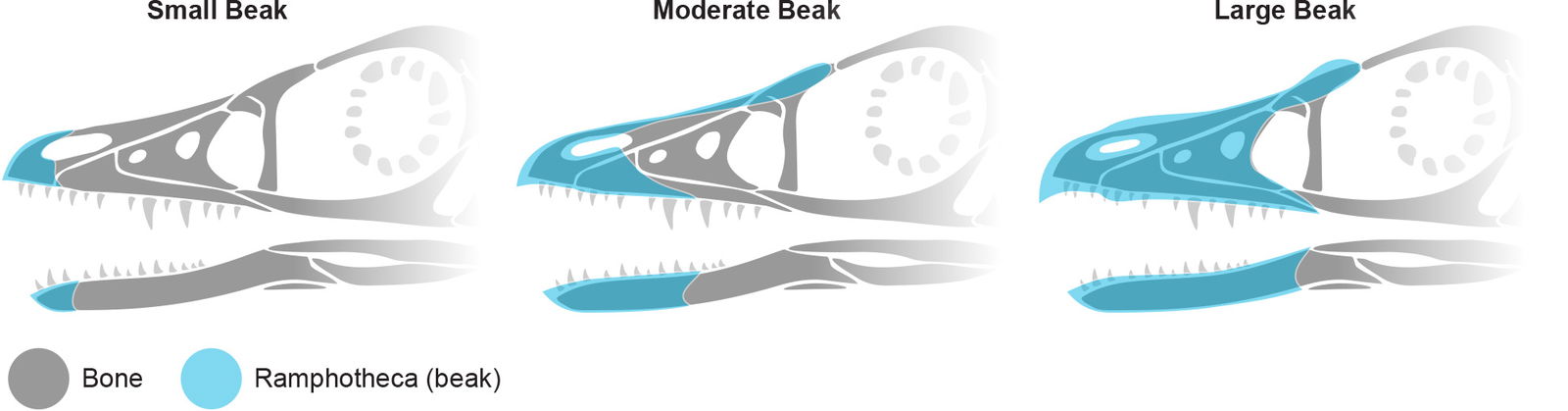
Fig. 28. Inferred ramphotheca (beak material) estimates for Microraptor. A creationist worldview negates the need to assume that beaks replaced teeth through evolution, so a beak and teeth can coexist on the same animal. Similar variety in beak size can be seen in Homing Pigeon breed variety from van Grouw (2018). Microraptor skull adapted from Hartman (n.d.) and Paul (2016) reconstructions.
This logic leads to a very different conclusion than an evolutionary worldview. Instead of assuming no beak until proof of a beak is found (evolutionary starting point), this starting point assumes birds possessed a beak structure until the evidence of soft tissues shows otherwise.
The evolutionary idea is that the beak replaced teeth through evolution. However, there are clear exceptions to this idea, as Louchart and Viriot (2011, 667) document in enantiornithine birds:
A rhamphotheca might have covered rostrolateral parts of the jaws while the dentition was still complete, in a few taxa.
The idea that a fully-toothed jaw must be beakless is an assumption based on evolutionary phylogeny. There are multiple examples of toothed animals that also possess beaks (fig. 29).
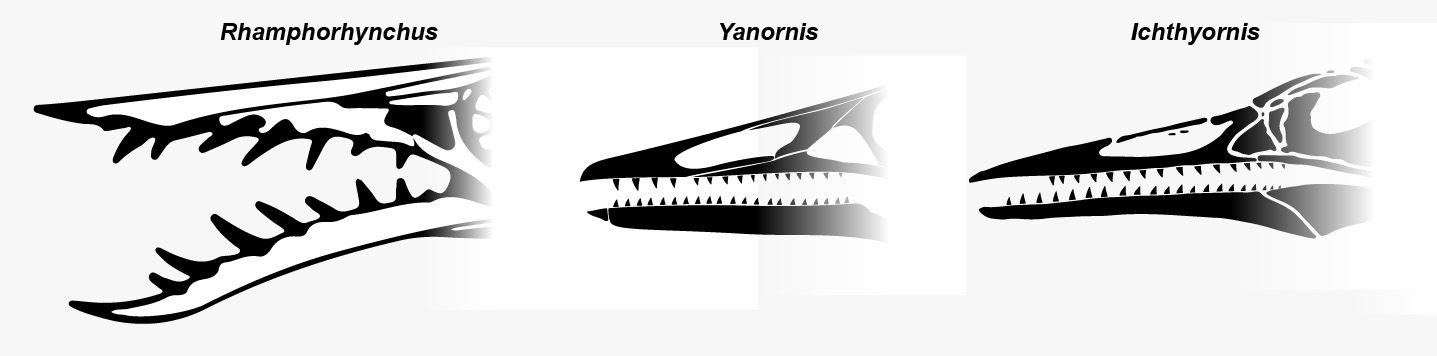
Fig. 29. Examples of animals that evolutionists believe had both teeth and a beak. This shows that beaks and teeth are not thought to be mutually exclusive by reason of observable science, but instead because of the story of evolution via the phylogenetic tree. Ramphorhyncus (lit. “beak snout”) skull adapted from Hartman (n.d.), Yanornis and Ichthyornis skulls adapted from Field et al. (2018). Individuals not to scale.
Some incorrectly claim that teeth are evidence of an animal being a dinosaur instead of a bird. However, as discussed previously, Ichthyornis, Hesperornis, Sinornis and others are considered avian, but have teeth and “were described as having osteological correlates that indicate the presence of compound rhamphotheca” (Chiappe and Dyke 2002; Field et al. 2018; Zheng et al. 2020).
Extant birds use their beak to maintain their feathers by preening using either oil from the uropygial gland at the base of the tail or the greasy powdery substance given off by powder down tracts among the feathers (Wetmore 1920). Feathers require almost constant maintenance. On average birds spend over 9% of their day caring for their feathers (Cotgreave and Clayton 1994). A toothed snout without a beak seems to be a very poor structure for feather care. Beaks, on the other hand, are well designed tools for preening feathers, removing dirt and debris, and removing harmful ectoparasites (Zhao et al. 2020). It appears that God created beaks as part of the advanced feather care system in birds (fig. 30). For animals that possessed clear pennaceous feathers it is logical to conclude that they likely had a beaklike structure to care for them.
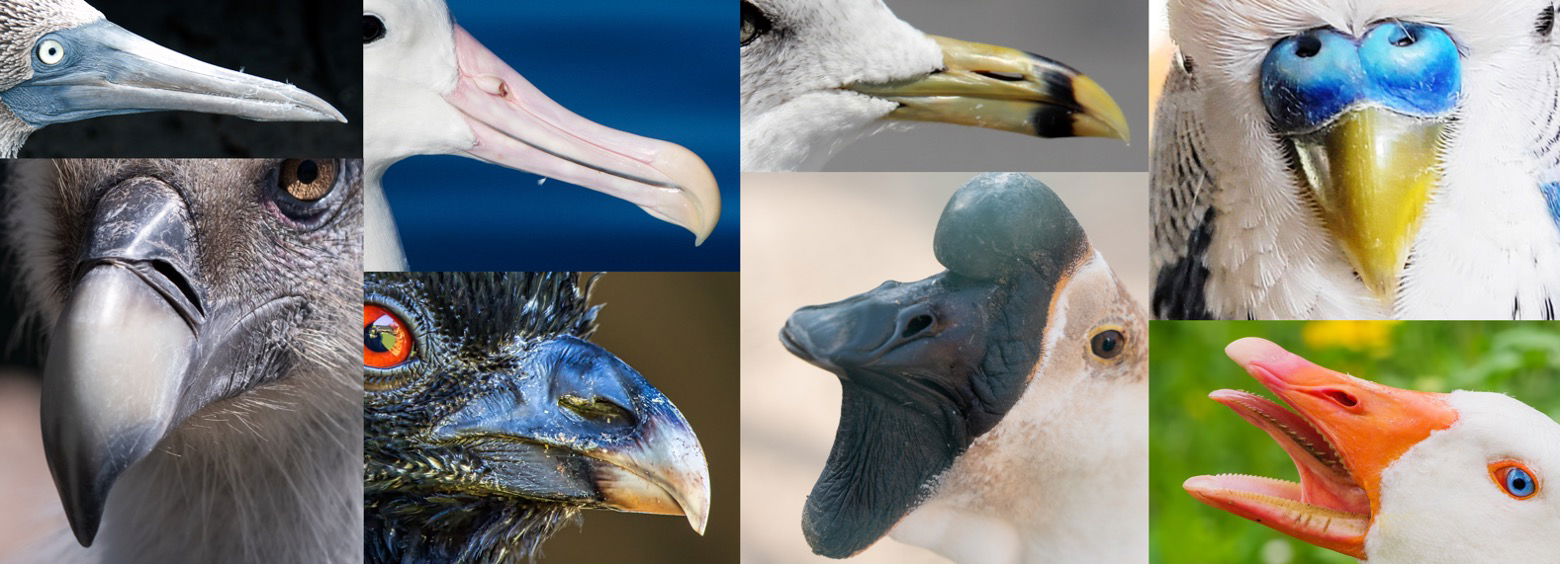
Fig. 30. Beaks are a designed structure with multiple functions including feather care. What appears to be a “beak” is often made up of multiple varying cornified parts including a keratinous nail on the tip, tough but less cornified tissue more caudally, “lip” tissue, and sometimes even featherless skin colored to match the beak. While the premaxillary nail rarely extends caudally past the nares, note how other parts of the rhamphotheca often surround the nares to varying degrees. This is true even in birds with a large naris opening in the skull such as the Ring-billed Gull (Hieronymus and Witmer 2010). Top (left to right): Blue Footed Boobie, Southern Royal Albatross, Ring-billed Gull, and English Budgie. Bottom (left to right): Griffon Vulture, Black Curassow, Lion Head Goose, and Snow Goose.
Paleoartists should use modern bird beaks as inspiration for what Microraptor’s beak may have looked like, taking note of the various cornified parts and ensuring that the naris (nostril) opening is accurately represented. Since Microraptor was carnivorous it may be that the beak was hooked to some degree, but the amount of hook is difficult to determine. It also could be that a strongly hooked beak was less necessary for birds with teeth.
Scales
Evolutionary paleoartists often represent “early” birds and supposed bird ancestors (like Microraptor) with scales (scutes) on their head. This is another decision based on an assumed phylogenetic tree that results in these animals looking more reptilian than they were.
Scale impressions have not been found on Microraptor. In fact, the opposite is true. Feather impressions are clear around the head and neck of several Microraptor specimens.
Modern birds do not have scales on their head or neck. In species where feathers are lacking such as scavenging vultures, the skin is either naked or in some cases has small filoplume feathers.
Paleoartists should use modern birds as inspiration for what the head skin and feathers may have looked like on Microraptor. Careful attention should be paid to any fossil feather impressions around the head area to accurately represent the feather length and feather distribution (see Appendix). Modern birds often have longer contour feathers on the top and back of the skull, making a smooth transition to the shoulders and sometimes even sporting a crest of some kind. The area between the eyes and the beak is often naked or only has small hairlike feathers (personal observation).
Methodology Objections
Below are some objections that may be raised against the methodology used in this paper.
Non-Phylogenetic Methodology
Some may object to the approach to reconstruction in this paper because it is primarily functional rather than phylogenetic. This objection is also seen in Christiansen and Bonde (2002) response to Jones et al. (2000). They say “Cladists have repeatedly emphasized that the function of anatomical features is irrelevant to characters in phylogenetic analyses.”
(Feduccia 2020) voices his frustration with this extreme philosophy when he states that, “if the cladogram requires irrational explanations, then surely something is wrong.”
As biblical creationists we need not be bound to an evolutionary cladogram (Sanders and Cserhati 2022). We know that God designs functional systems with complex interrelated features. We do not have to be concerned with what is derived or basal, whether a feature is a synapomorphy or convergence. We are free to study creation knowing that the Creator was intentional in the designs we find. But even in a fallen creation we see God’s functional genius displayed.
Present is the Key to the Past Approach
Some may object that the anatomy of modern animals weighed too heavily into the interpretation of Microraptor in this report. Some may argue that this approach is rooted in the very uniformitarianism that creationists have argued against for decades. However, the opposite is true. The approach used here is not rooted in millions of years of slow change. The assumption of millions of years of evolution indicates that modern creatures are too different to look for close anatomical analogs among fossils. Instead, a biblical worldview leads to the starting point that extinct animals are likely similar in many ways to modern animals since they have a common Designer. Uniformitarianism has historically been argued against in a geologic context because it conflicts with the true history recorded in the Word of God. But comparative anatomy between extinct and extant creatures can be a valuable tool.
Predictions
Critics often claim that there are no testable predictions made in creation science. If Microraptor and other feathered maniraptors are birds as some scientists claim, then there should be testable predictions about future discoveries.
Predictions about feathered maniraptors:
- More maniraptors will be found with large, fully developed wings with feathers arranged the same as in modern birds.
- Secondarily flightless maniraptors will be found with wings similar to flightless birds today, not with primitive, half-evolved wings.
- A maniraptor will eventually be found with beak tissue on the snout and none with scales on the head
- A maniraptor will be found with alula feathers and no scales on the manus
- A maniraptor larger than Zhenyuanlong will be found with a thin tail with 30 caudal vertebrae or less instead of a large transitional tail form between dinosaurs and birds
- A large maniraptor will be found with avian soft tissue such as patagia or organ structure (lungs, digestive tract, etc.).
- Maniraptors will continue to be found exhibiting avian behavior such as eggs and nesting.
Non-maniraptoran theropods will be found with:
- Filaments, and collagen fibers, but not feathers (Feduccia, Lingham-Soliar, and Hinchliffe 2005)
- Long tails with large amounts of inferred muscle and soft tissue
- Fewer clear bird-like features than expected
- Scaly skin throughout with no true feathers
- Evolutionists will continue to claim to find what many of them will call a missing link between birds and dinosaurs, but some of them will classify as bird, clearly dinosaur, or too disarticulated or poorly preserved for classification
Conclusion
Reconstructing Microraptor as a bird is most consistent with a biblical worldview because it avoids the many evolutionary-influenced decisions that make it look like a feathered dinosaur.
This paper confirms that a rigorous scientific reconstruction of Microraptor reveals that it looked like a modern bird, not a primitive, half-bird transitional form or feathered dinosaur. Based on the evidence presented here, a human observing Microraptor in life would immediately recognize it as a bird with these traits:
- Swivel wrist for folding the wing against the body
- Manual digits attached as a single unit inside the wing
- Pro- and post-patagia that gave the forelimb a wing shape
- Flight feathers arranged like extant birds
- Shoulder mobility that enabled wing flapping
- Flight muscles that powered wing flapping
- Unfused pygostyle that was thin, short, and stiffened
- Crouched legs with “knee walking” mobility
- No head scales and a beaked rostrum
Reconstructing Microraptor as a bird is most consistent with the evidence shown above. Even some evolutionists agree on the basis of comparative anatomy that these animals were birds. Feduccia (2020) states, “When I first saw a specimen of Microraptor in Beijing, I thought it had all the earmarks of a primitive bird,” and later, “In my view, Microraptor and Archaeopteryx—sinornithosaurs and allies—are likely early birds and not dinosaurs.”
There is much more research to be done on these animals. Artwork can communicate accuracy about these animals, so it should be done carefully based on the evidence interpreted from a biblical worldview.
References
Agnolin, Federico L., Matias J. Motta, Federico Brissón Egli, Gastón Lo Coco, and Fernando E. Novas. 2019. “Paravian Phylogeny and the Dinosaur-Bird Transition: An Overview.” Frontiers in Earth Science 6 (12 February). https://doi.org/10.3389/feart.2018.00252.
Agnolin, Federico, and Fernando E. Novas. 2013. Avian Ancestors: A Review of the Phylogenetic Relationships of the Theropods Unenlagiidae, Microraptoria, Anchiornis and Scansoriopterygidae. Dordrecht, Netherlands: Springer.
Alexander, David E., Enpu Gong, Larry D. Martin, David A. Burnham, and Amanda R. Falk. 2010. “Model Tests of Gliding with Different Hindwing Configurations in the Four-Winged Dromaeosaurid Microraptor Gui.” Proceedings of the National Academy of Sciences of the United States of America 107, no. 7 (February 16): 2972–2976.
Allen, Vivian, Karl T. Bates, Zhiheng Li, and John R. Hutchinson. 2013. “Linking the Evolution of Body Shape and Locomotor Biomechanics in Bird-Line Archosaurs.” Nature 497, no. 7447 (2 May): 104–107.
Baliga, V. B., I. Szabo, and D. L. Altshuler. 2019. “Range of Motion in the Avian Wing Is Strongly Associated with Flight Behavior and Body Mass.” Science Advances 5, no. 10 (11 October). https://doi.org/10.1126/sciadv.aaw6670.
Bates, Karl T., Phillip L. Manning, David Hodgetts, and William I. Sellers. 2009. “Estimating Mass Properties of Dinosaurs Using Laser Imaging and 3D Computer Modelling.” PLoS ONE 4, no. 2 (February 19). https://doi.org/10.1371/journal.pone.0004532.
Bishop, Peter J., Krijn B. Michel, Antoine Falisse, Andrew R. Cuff, Vivian R. Allen, Friedl de Groote, and John R. Hutchinson. 2021. “Computational Modelling of Muscle Fibre Operating Ranges in the Hindlimb of a Small Ground Bird (Eudromia elegans), with Implications for Modelling Locomotion in Extinct Species.” PLoS Computational Biology 17, no. 4 (April 1). https://doi.org/10.1371/journal.pcbi.1008843.
Bock, Walter J. 2013. “The Furcula and the Evolution of Avian Flight.” Paleontological Journal 47, no. 11 (19 December): 1236–1244.
Bonnan, Matthew F., Jennifer L. Sandrik, Takahiko Nishiwaki, D. Ray Wilhite, Ruth M. Elsey, and Christopher Vittore. 2010. “Calcified Cartilage Shape in Archosaur Long Bones Reflects Overlying Joint Shape in Stress-Bearing Elements: Implications for Nonavian Dinosaur Locomotion.” Anatomical Record 293, no. 12 (December): 2044–2055.
Bortoluzzi, Chiara, Hendrik-Jan Megens, Mirte Bosse, Martijn F. L. Derks, Bert Dibbits, Kimberly Laport, Steffen Weigend, Martien A. M. Groenen, and Richard P. M. A. Crooijmans. 2020. “Parallel Genetic Origin of Foot Feathering in Birds.” Molecular Biology and Evolution 37, no. 9 (September 1): 2465–2476.
Botelho, João Francisco, Luis Ossa-Fuentes, Sergio Soto-Acuña, Daniel Smith-Paredes, Daniel Nuñez-León, Miguel Salinas-Saavedra, Macarena Ruiz-Flores, and Alexander O. Vargas. 2014. “New Developmental Evidence Clarifies the Evolution of Wrist Bones in the Dinosaur–Bird Transition.” PLoS Biology 12, no. 9 (September 30). https://doi.org/10.1371/journal.pbio.1001957.
Bradley, Alexander B., Sara H. Burch, Alan H. Turner, Nathan D. Smith, Randall B. Irmis, and Sterling J. Nesbitt. 2019. “Sternal Elements of Early Dinosaurs Fill a Critical Gap in the Evolution of the Sternum in Avemetatarsalia (Reptilia: Archosauria).” Journal of Vertebrate Paleontology 39, no. 5 (5 February). https://doi.org/10.1080/02724634.2019.1700992.
Brougham, Jason, and Stephen L. Brusatte. 2010. “Distorted Microraptor Specimen Is Not Ideal for Understanding the Origin of Avian Flight.” Proceedings of the National Academy of Sciences 107, no. 40 (September): E155. https://doi.org/10.1073/pnas.1004977107.
Brusatte, Stephen L., Graeme T. Lloyd, Steve C. Wang, and Mark A. Norell. 2014. “Gradual Assembly of Avian Body Plan Culminated in Rapid Rates of Evolution across the Dinosaur-Bird Transition.” Current Biology 24 (20): 2386–92. https://doi.org/10.1016/j.cub.2014.08.034.
Burnham, David A. 2008. “A Review of the Early Cretaceous Jehol Group of Northeastern China and a Revision Concerning the Origin of Flight Paradigm.” Oryctos 7: 27–43.
Button, Khai Allen. 2018. “Soft Tissue Reconstruction and Ecomorphology of Beaks in Extant and Extinct Theropod Dinosaurs.” Ph.D. Diss., North Caroline State University.
Carpenter, Kenneth. 2002. “Forelimb Biomechanics of Nonavian Theropod Dinosaurs in Predation.” Palaeobiodiversity and Palaeoenvironments 82, no. 1 (June): 59–75.
Carrano, Matthew T, and Andrew A Biewener. 1999. “Experimental Alteration of Limb Posture in the Chicken (Gallus Gallus) and Its Bearing on the Use of Birds as Analogs for Dinosaur Locomotion.” Journal of Morphology 240, no. 3 (June): 237–249.
Cau, Andrea, and Paolo Serventi. 2017. “Origin Attachments of the Caudofemoralis Longus Muscle in the Jurassic Dinosaur Allosaurus.” Acta Palaeontologica Polonica 62, no. 2: 273–277.
Chatterjee, Sankar, and R. Jack Templin. 2007. “Biplane Wing Planform and Flight Performance of the Feathered Dinosaur Microraptor Gui.” Proceedings of the National Academy of Sciences 104, no. 5 (January 30): 1576–1580.
Chatterjee, Sankar, and R. Jack Templin. 2012. “Palaeoecology, Aerodynamics, and the Origin of Avian Flight.” In Earth and Life: Global Biodiversity, Extinction Intervals and Biogeographic Perturbations Through Time, 585–612. Dordrecht, Netherlands: Springer.
Chiappe, Luis M., and Gareth J. Dyke. 2002. “The Mesozoic Radiation of Birds.” Annual Review of Ecology and Systematics 33: 91–124.
Chiappe, Luis M., Ji Shu’an, Ji Qiang, and Mark A. Norell. 1999. “Anatomy and Systematics of the Confuciusornithidae (Theropoda: Aves) From the Late Mesozoic of Northeastern China.” Bulletin of the American Museum of Natural History 242 (November): 1–89.
Chiappe, Luis M., and Lawrence M. Witmer. 2002. Mesozoic Birds: Above the Heads of Dinosaurs. Berkeley, Carlifornia: University of California Press.
Christiansen, Per, and Niels Bonde. 2002. “Limb Proportions and Avian Terrestrial Locomotion.” Journal of Ornithology 143 (1 July): 356–371.
Clark, George A. 1961. “Occurrence and Timing of Egg Teeth in Birds.” The Wilson Bulletin 73, no. 3 (September): 268–278.
Cotgreave, Peter, and Dale H. Clayton.1994. “Comparative Analysis of Time Spent Grooming By Birds in Relation to Parasite Load.” Behaviour 131, nos. 3–4 (December): 171–187.
Degernes, Laurel A., and Alan Feduccia. 2001. “Tenectomy of the Supracoracoideus Muscle to Deflight Pigeons (Columba livia) and Cockatiels (Nymphicus hollandicus).” Journal of Avian Medicine and Surgery 15, no. 1 (March): 10–16.
Domyan, Eric T., Zev Kronenberg, Carlos R. Infante, Anna I. Vickrey, Sydney A. Stringham, Rebecca Bruders, Michael W. Guernsey, et al. 2016. “Molecular Shifts in Limb Identity Underlie Development of Feathered Feet in Two Domestic Avian Species.” ELife 5 (March): e12115. https://doi.org/10.7554/eLife.12115.
Doube, Michael, Stephanie C. W. Yen, Michał M. Kłosowski, Andrew A. Farke, John R. Hutchinson, and Sandra J. Shefelbine. 2012. “Whole-Bone Scaling of the Avian Pelvic Limb.” Journal of Anatomy 221, no. 1 (July): 21–29.
Dyke, Gareth, Roeland de Kat, Colin Palmer, Jacques van der Kindere, Darren Naish, and Bharathram Ganapathisubramani. 2013. “Aerodynamic Performance of the Feathered Dinosaur Microraptor and the Evolution of Feathered Flight.” Nature Communications 4 (18 September): 2489. https://doi.org/10.1038/ncomms3489.
Everhart, Michael J. 2011. “Rediscovery of the Hesperornis regalis Marsh 1871 Holotype Locality Indicates an Earlier Stratigraphic Occurrence.” Transactions of the Kansas Academy of Science 114, nos. 1–2 (Spring): 59–68.
Feduccia, Alan. 2020. Romancing the Birds and Dinosaurs: Forays in Postmodern Paleontology. Irvine, California: Brown Walker Press.
Feduccia, Alan. 2023. “The Avian Acetabulum: Small Structure, But Rich With Illumination and Questions.” Diversity 16, no. 1 (27 December): 20. https://doi.org/10.3390/d16010020.
Feduccia, Alan, and Stephen A. Czerkas. 2015. “Testing the Neoflightless Hypothesis: Propatagium Reveals Flying Ancestry of Oviraptorosaurs.” Journal of Ornithology 156, no. 4 (19 March): 1067–1074.
Feduccia, Alan, Theagarten Lingham-Soliar, and J. Richard Hinchliffe. 2005. “Do Feathered Dinosaurs Exist? Testing the Hypothesis on Neontological and Paleontological Evidence.” Journal of Morphology 266, no. 2 (November): 125–166.
Fell, Honor Bridget. 1939. “The Origin and Developmental Mechanics of the Avian Sternum.” Philosophical Transaction of the Royal Society of London B 229, no. 563 (20 March): 407–463.
Field, Daniel J., Michael Hanson, David Burnham, Laura E. Wilson, Kristopher Super, Dana Ehret, Jun A. Ebersole, and Bhart-Anjan S. Bhullar. 2018. “Complete Ichthyornis Skull Illuminates Mosaic Assembly of the Avian Head.” Nature 557, no. 7703 (2 May): 96–100.
Fisher, Harvey I. 1940. “The Occurrence of Vestigial Claws on the Wings of Birds.” American Midland Naturalist 23 (1): 234. https://doi.org/10.2307/2485270.
Fisher, Harvey I. 1957. “The Function of M. Depressor Caudae and M. Caudofemoralis in Pigeons.” The Auk 74, no. 4 (October): 479–486.
Foth, Christian. 2014. “Comment on the Absence of Ossified Sternal Elements in Basal Paravian Dinosaurs.” Proceedings of the National Academy of Sciences 111, no. 50 (December 5): E5334.
Fowler, Denver W., Elizabeth A. Freedman, John B. Scannella, and Robert E. Kambic. 2011. “The Predatory Ecology of Deinonychus and the Origin of Flapping in Birds.” PLoS ONE 6, no. 12 (December 14). https://doi.org/10.1371/journal.pone.0028964.
Gatesy, Stephen M., Martin Bäker, and John R. Hutchinson. 2009. “Constraint-Based Exclusion of Limb Poses for Reconstructing Theropod Dinosaur Locomotion.” Journal of Vertebrate Paleontology 29, no. 2 (June 12): 535–544.
Gatesy, Stephen M., and Kevin M. Middleton. 1997. “Bipedalism, Flight, and the Evolution of Theropod Locomotor Diversity.” Journal of Vertebrate Paleontology 17, no. 2 (June 19): 308–329.
Gishlick, Alan D. 2001. “The Function of the Manus and Forelimb of Deinonychus antirrhopus and Its Importance for the Origin of Avian Flight.” In New Perspectives on the Origin and Early Evolution of Birds. Edited by Jacques Gauthier, and Lawrence F. Gall, 301–318. New Haven, Connecticut: Peabody Museum of Natural History.
Grossi, Bruno, José Iriarte-Díaz, Omar Larach, Mauricio Canals, and Rodrigo A. Vásquez. 2014. “Walking Like Dinosaurs: Chickens with Artificial Tails Provide Clues about Non-Avian Theropod Locomotion.” PLoS ONE 9, no. 2 (February 5). https://doi.org/10.1371/journal.pone.0088458.
Harrison, Greg J. 1993. “Handbook of Avian Anatomy: Nomina Anatomica Avium.” Journal of the Association of Avian Veterinarians 7, no. 3: 156.
Hartman, Scott. n.d.. “Theropod Skeletal Reconstructions.” Dr. Scott Hartman’s Skeletal Drawing.com. https://www.skeletaldrawing.com/theropods/.
Haynes, Gabriela. 2023. “Reply to the ‘Response to “The Debate over Classification of Archaeopteryx as a Bird’”.” Answers Research Journal 16 (December 6): 595–634. https://answersresearchjournal.org/dinosaurs/reply-response-debate-classification-archaeopteryx-bird/.
Headden, Jaime A. 2006. “Microraptor Skeletons by Qilong.” DeviantArt, January 6. https://www.deviantart.com/qilong/art/Microraptor-Skeletons-27326378.
Hebert, Leo III. 2023. “Allometry and Metabolic Scaling: Arguments for Design . . . and Clues to Explaining Pre-Flood Longevity?” In Proceedings of the Ninth International Conference on Creation, Article 18. Cedarville, Ohio, July 16–19. https://publications.cedarville.edu/icc_proceedings/vol_9_2023/206/.
Heers, Ashley M., and Kenneth P. Dial. 2012. “From Extant to Extinct: Locomotor Ontogeny and the Evolution of Avian Flight.” Trends in Ecology & Evolution 27, no. 5 (May): 296–305.
Hieronymus, Tobin L. 2016. “Flight Feather Attachment in Rock Pigeons (Columba livia): Covert Feathers and Smooth Muscle Coordinate a Morphing Wing.” Journal of Anatomy 229, no. 5 (20 June): 631–656.
Hieronymus, Tobin L., and Lawrence M. Witmer. 2010. “Homology and Evolution of Avian Compound Rhamphothecae.” The Auk 127, no. 3 (1 July): 590–604.
Holliday, Casey M., Ryan C. Ridgely, Jayc C. Sedlmayr, and Lawrence M. Witmer. 2010. “Cartilaginous Epiphyses in Extant Archosaurs and Their Implications for Reconstructing Limb Function in Dinosaurs.” PLoS ONE 5, no. 9 (September 30): 1–16. https://doi.org/10.1371/journal.pone.0013120.
Hone, David W. E., Helmut Tischlinger, Xing Xu, and Fucheng Zhang. 2010. “The Extent of the Preserved Feathers on the Four-Winged Dinosaur Microraptor gui Under Ultraviolet Light.” PLoS ONE 5, no. 2 (February 15). https://doi.org/10.1371/journal.pone.0009223.
Hutchinson, John R., and Vivian Allen. 2009. “The Evolutionary Continuum of Limb Function From Early Theropods to Birds.” Naturwissenschaften 96, no. 4 (24 December): 423–448.
Hutchinson, John R., and Stephen M. Gatesy. 2000. “Adductors, Abductors, and the Evolution of Archosaur Locomotion.” Paleobiology 26, no. 4 (Autumn): 734–751.
Hutson, Joel D, and Kelda N Hutson. 2018. “Retention of the Flight-Adapted Avian Finger-Joint Complex in the Ostrich Helps Identify When Wings Began Evolving in Dinosaurs.” Ostrich 89, no. 2 (30 January): 173–186.
Hwang, Sunny H, Mark A Norell, J I Qiang, and Gao Keqin. 2002. “New Specimens of Microraptor zhaoianus (Theropoda: Dromaeosauridae) From Northeastern China.” American Museum Novitates 3381: 1–44.
James, Frances C., and John A. Pourtless IV. 2009. “Cladistics and the Origin of Birds: A Review and Two New Analyses.” Ornithological Monographs 66, no. 1. Edited by John Faaborg, 1–78.
Jones, Terry D., James O. Farlow, John A. Ruben, Donald M. Henderson, and Willem J. Hillenius. 2000. “Cursoriality in Bipedal Archosaurs.” Nature 406, no. 6797 (17 August): 716–718.
Kiat, Yosef, Amir Balaban, Nir Sapir, Jingmai Kathleen O’Connor, Min Wang, and Xing Xu. 2020. “Sequential Molt in a Feathered Dinosaur and Implications For Early Paravian Ecology and Locomotion.” Current Biology 30, no. 18 (21 September): 3633–3638.e2. https://doi.org/10.1016/j.cub.2020.06.046.
Kiat, Yosef, and Jingmai K. O’Connor. 2024. “Functional Constraints on the Number and Shape of Flight Feathers.” Proceedings of the National Academy of Sciences USA 121, no. 8 (February 12). https://doi.org/10.1073/pnas.2306639121.
Knutsen, Espen M. 2007. “Beak Morphology in Extant Birds with Implications on Beak Morphology in Ornithomimids.”
Lee, Michael S. Y., Andrea Cau, Darren Naish, and Gareth J. Dyke. 2014. “Sustained Miniaturization and Anatomical Innovation in the Dinosaurian Ancestors of Birds.” Science 345, no. 6196 (1 August): 562–566.
Lee, Sang-im, Jooha Kim, Hyungmin Park, Piotr G. Jabłoński, and Haecheon Choi. 2015. “The Function of the Alula in Avian Flight.” Scientific Reports 5 (May): Article 9914. https://doi.org/10.1038/srep09914.
Li, Quanguo, Ke-Qin Gao, Qingjin Meng, Julia A. Clarke, Matthew D. Shawkey, Liliana D’Alba, Rui Pei, Mick Ellison, Mark A. Norell, and Jakob Vinther. 2012. “Reconstruction of Microraptor and the Evolution of Iridescent Plumage.” Science 335, no. 6073 (9 March): 1215–1219.
Lightner, Jean, Tom Hennigan, Georgia Purdom, and Bodie Hodge. 2011. “Determining the Ark Kinds.” Answers Research Journal 4 (August 1): 195–201. https://answersresearchjournal.org/determining-the-ark-kinds/.
Lingham-Soliar, Theagarten. 2015. “Birds.” In The Vertebrate Integument. Vol. 2 Structure, Design and Function, 163–262. Berlin, Germany: Springer. https://doi.org/10.1007/978-3-662-46005-4_5.
Louchart, Antoine, and Laurent Viriot. 2011. “From Snout to Beak: The Loss of Teeth in Birds.” Trends in Ecology and Evolution 26, no. 12 (December): 663–673.
Lü, Junchang, and Stephen L. Brusatte. 2015. “A Large, Short-Armed, Winged Dromaeosaurid (Dinosauria: Theropoda) from the Early Cretaceous of China and Its Implications For Feather Evolution.” Scientific Reports 5 (16 July): Article 11775. https://doi.org/10.1038/srep11775.
Manafzadeh, Armita R., and Kevin Padian. 2018. “ROM Mapping of Ligamentous Constraints on Avian Hip Mobility: Implications for Extinct Ornithodirans.” Proceedings of the Royal Society B: Biological Sciences 285, no. 1879 (30 May). https://doi.org/10.1098/RSPB.2018.0727.
Mayr, Gerald. 2017. “Pectoral Girdle Morphology of Mesozoic Birds and the Evolution of the Avian Supracoracoideus Muscle.” Journal of Ornithology 158, no. 3 (March): 859–867.
Meadows, Robin. 2014. “Resolving the Flap over Bird Wrists.” PLoS Biology 12, no. 9 (September 30): e1001958. https://doi.org/10.1371/journal.pbio.1001958.
Moreno, Eulalia I, and Ape TM Moller. 1996. “Morphological Aspects of Avian Tail Movements: A Functional Approach in Hirundines.” The Auk, 113, no. 3 (July): 647–654.
Motta, Matías J., Federico L. Agnolín, Federico Brissón Egli, and Fernando E. Novas. 2020. “New Theropod Dinosaur From the Upper Cretaceous of Patagonia Sheds Light on the Paravian Radiation in Gondwana.” Science of Nature 107, no. 3 (29 May): Article 24. https://doi.org/10.1007/s00114-020-01682-1.
Norberg, Ulla M. Lindhe. 1985. “Evolution of Vertebrate Flight: An Aerodynamic Model for the Transition From Gliding to Active Flight.” The American Naturalist 126, no. 3 (September): 303–327.
Norell, Mark A., and Peter J Makovicky. 1999. “Important Features of the Dromaeosaurid Skeleton II: Information From Newly Collected Specimens of Velociraptor Mongoliensis.” American Museum Novitates: 3282.
Novas, Fernando E., Federico L. Agnolín, Federico Brisson Egli, and Gastón E. Lo Coco. 2020. “Pectoral Girdle Morphology in Early-Diverging Paravians and Living Ratites: Implications for the Origin of Flight.” In Pennaraptoran Theropod Dinosaurs Past Progress and New Frontiers. Edited by Michael Pittman and Xing Xu, 345–353. Bulletin of the American Museum of Natural History 440, no. 1 (21 August).
Novas, Fernando E., Matías J. Motta, Federico L. Agnolín, Sebastián Rozadilla, Gastón E. lo Coco, and Federico Brissón Egli. 2021. “Comments on the Morphology of Basal Paravian Shoulder Girdle: New Data Based on Unenlagiid Theropods and Paleognath Birds.” Frontiers in Earth Science 9 (25 May). https://doi.org/10.3389/feart.2021.662167.
O’Connor, Jingmai, Zheng Xiao-Ting, Xiaoli Wang, Xiao-Mei Zhang, and Zhou Zhong-He. 2015. “The Gastral Basket in Basal Birds and Their Close Relatives: Size and Possible Function.” Vertebrata Palasiatica 53 (September): 133–152.
O’Connor, Jingmai, Xiaoting Zheng, Liping Dong, Xiaoli Wang, Yan Wang, Xiaomei Zhang, and Zhonghe Zhou. 2019. “Microraptor with Ingested Lizard Suggests Non-Specialized Digestive Function.” Current Biology 29, no. 14 (22 July): 2423–2429.e2. https://doi.org/10.1016/j.cub.2019.06.020.
Olson, Storrs L., and Alan Feduccia. 1979. “Flight Capability and the Pectoral Girdle of Archaeopteryx.” Nature 278, no. 5701 (March 15): 247–248.
Ostrom, John H. 1969. “Osteology of Deinonychus antirrhopus, an Unusual Theropod from the Lower Cretaceous of Montana.” Bulletin Peabody Museum of Natural History 30 (July): 1–164.
Paul, Gregory S. 2002. Dinosaurs of the Air: The Evolution and Loss of Flight in Dinosaurs and Birds. Baltimore, Maryland: Johns Hopkins University Press.
Paul, Gregory S. 2016. The Princeton Field Guide to Dinosaurs. 2nd ed. Princeton, New Jersey: Princeton University Press.
Persons W. Scott IV, and Philip J. Currie. 2011a. “Dinosaur Speed Demon: The Caudal Musculature of Carnotaurus sastrei and Implications for the Evolution of South American Abelisaurids.” PLoS ONE 6, no. 10 (October 17): e25763. https://doi.org/10.1371/journal.pone.0025763.
Persons, W. Scott IV, and Philip J. Currie. 2011b. “The Tail of Tyrannosaurus: Reassessing the Size and Locomotive Importance of the M. caudofemoralis in Non-Avian Theropods.” Anatomical Record 294, no. 1 (January): 119–131.
Poore, Samuel O. 2008. “The Morphological Basis of the Arm-to-Wing Transition.” The Journal of Hand Surgery 33, no. 2 (February): 277–280.
Poore, Samuel O., A. Sánchez-Haiman, and G. E. Goslow Jr. 1997. “Wing Upstroke and the Evolution of Flapping Flight.” Nature 387. no. 6635 (19 June): 799–802.
“Prehistoric Planet.” 2002. Apple TV. Scientific advisor: Dr. Darren Naish https://tv.apple.com/us/show/prehistoric-planet/umc.cmc.4lh4bmztauvkooqz400akxav.
Rashid, Dana J., Kevin Surya, Luis M. Chiappe, Nathan Carroll, Kimball L. Garrett, Bino Varghese, Alida Bailleul, Jingmai K. O’Connor, Susan C. Chapman, and John R. Horner. 2018. “Avian Tail Ontogeny, Pygostyle Formation, and Interpretation of Juvenile Mesozoic Specimens.” Scientific Reports 8, no. 1 (13 June): Article 9014. https://doi.org/10.1038/s41598-018-27336-x.
Roach, John. 2007. “‘Dinosaur Mummy’ Found; Has Intact Skin, Tissue.” https://www.nationalgeographic.com/science/article/north-dakota-dinosaur-mummy.
Ruben, John A., Terry D. Jones, Nicholas R. Geist, and W. Jaap Hillenius. 1997. “Lung Structure and Ventilation in Theropod Dinosaurs and Early Birds.” Science 278, no. 5341 (14 November): 1267–1270.
Sanders, Harry, and Matthew Cserhati. 2022. “Statistics, Baraminology, and Interpretations: A Critical Evaluation of Current Morphology-Based Baraminology Methods.” Creation Research Society Quarterly 58, no. 3 (Winter): 175–192.
Senter, Phil. 2006. “Comparison of Forelimb Function between Deinonychus and Bambiraptor (Theropoda: Dromaeosauridae).” Journal of Vertebrate Paleontology 26, no. 4: 897–906.
Smithwick, Fiann. 2019. “A Taphonomic and Palaeoecological Approach to the Study of Palaeocolour.” Ph.D. diss, University of Bristol.
Sullivan, Corwin, David W. E. Hone, Xing Xu, and Fucheng Zhang. 2010. “The Asymmetry of the Carpal Joint and the Evolution of Wing Folding in Maniraptoran Theropod Dinosaurs.” Proceedings of the Royal Society B: Biological Sciences 277, no. 1690 (7 July): 2027–2033.
van der Reest, Aaron J., and Philip J. Currie. 2017. “Troodontids (Theropoda) From the Dinosaur Park Formation, Alberta, with a Description of a Unique New Taxon: Implications for Deinonychosaur Diversity in North America.” Canadian Journal of Earth Sciences 54, no. 9 (8 August): 919–935.
van Grouw, Katrina. 2013. The Unfeathered Bird. Princeton, New Jersey: Princeton University Press.
van Grouw, Katrina. 2018. Unnatural Selection. Princeton, New Jersey: Princeton University Press.
Vasquez, Rick J. 1992. “Functional Osteology of the Avian Wrist and the Evolution of Flapping Flight.” Journal of Morphology 211, no. 3 (March): 259–268.
Vasquez, Rick J. 1994. “The Automating Skeletal and Muscular Mechanisms of the Avian Wing (Aves).” Zoomorphology 114 (March): 59–71
Walker, Alick D. 1972. “New Light on the Origin of Birds and Crocodiles.” Nature 237, no. 5353 (2 June): 257–263.
Wang, Shiying, Yubo Ma, Qian Wu, Min Wang, Dongyu Hu, Corwin Sullivan, and Xing Xu. 2022. “Digital Restoration of the Pectoral Girdles of Two Early Cretaceous Birds and Implications for Early-Flight Evolution.” ELife 11 (March 31). https://doi.org/10.7554/ELIFE.76086.
Wang, Shuo, Josef Stiegler, Ping Wu, Cheng-Ming Chuong, Dongyu Hu, Amy Balanoff, Yachun Zhou, and Xing Xu. 2017. “Heterochronic Truncation of Odontogenesis in Theropod Dinosaurs Provides Insight into the Macroevolution of Avian Beaks.” Proceedings of the National Academy of Sciences of the United States of America 114, no. 41 (September 25): 10930–10935.
Wang, Xiaoli, Michael Pittman, Xiaoting Zheng, Thomas G. Kaye, Amanda R. Falk, Scott A. Hartman, and Xing Xu. 2017b. “Basal Paravian Functional Anatomy Illuminated by High-Detail Body Outline.” Nature Communications 8 (March): Article number 14576. https://doi.org/10.1038/ncomms14576.
Wang, Yan, Han Hu, Jingmai K. O’Connor, Min Wang, Xing Xu, Zhonghe Zhou, Xiaoli Wang, and Xiaoting Zheng. 2017. “A Previously Undescribed Specimen Reveals New Information on the Dentition of Sapeornis chaoyangensis.” Cretaceous Research 74 (June): 1–10.
Wellnhofer, Peter. 2009. Archaeopteryx: The Icon of Evolution. Trans. Frank Haase. Munich, Germany: F. Pfeil.
Wetmore, Alexander. 1920. “The Function of Powder Downs in Herons.” The Condor 22, no. 5 (September–October): 168–170.
Xing, Xu, and Li Feng. 2016. “A New Microraptorine Specimen ( Theropoda: Dromaeosauridae) with a Brief Comment on the Evolution of Compound Bones in Theropods.” Vertebrata Palasiatica 54, no. 4 (October): 269–285.
Xu, Xing, Philip Currie, Michael Pittman, Lida Xing, Qingjin Meng, Junchang Lü, Dongyu Hu, and Congyu Yu. 2017. “Mosaic Evolution in an Asymmetrically Feathered Troodontid Dinosaur with Transitional Features.” Nature Communications 8 (2 May). https://doi.org/10.1038/ncomms14972.
Xu, Xing, Zhonghe Zhou, Xiaolin Wang, Xuewen Kuang, Fucheng Zhang, and Xiangke Du. 2003. “Four-Winged Dinosaurs from China.” Nature 421, no. 6921 (23 January): 335–340.
Zhao, Jing-Shan, Jiayue Zhang, Yuping Zhao, Zhaodong Zhang, and Pascal Godefroit. 2020. “Shaking the Wings and Preening Feathers with the Beak Help a Bird to Recover Its Ruffled Feather Vane.” Materials and Design 187 (February): 108410. https://doi.org/10.1016/j.matdes.2019.108410.
Zheng, Xiaoting, Jingmai K. O’Connor, Xiaoli Wang, Yanhong Pan, Yan Wang, Min Wang, and Zhonghe Zhou. 2017. “Exceptional Preservation of Soft Tissue in a New Specimen of Eoconfuciusornis and Its Biological Implications.” National Science Review 4, no. 3 (May): 441–452.
Zheng, Xiaoting, Jingmai O’Connor, Yan Wang, Xiaoli Wang, Yin Xuwei, Xiaomei Zhang, and Zhonghe Zhou. 2020. “New Information on the Keratinous Beak of Confuciusornis (Aves: Pygostylia) From Two New Specimens.” Frontiers in Earth Science 8 (September). https://doi.org/10.3389/feart.2020.00367.
Zheng, Xiaoting, Xing Xu, Hailu You, Qi Zhao, and Zhiming Dong. 2010. “A Short-Armed Dromaeosaurid from the Jehol Group of China with Implications for Early Dromaeosaurid Evolution.” Proceedings of the Royal Society B: Biological Sciences 277, no. 1679 (January 22): 211–217.
Zheng, Xiaoting, Zhonghe Zhou, Xiaoli Wang, Fucheng Zhang, Xiaomei Zhang, Yan Wang, Guangjin Wei, Shuo Wang, and Xing Xu. 2013. “Hind Wings in Basal Birds and the Evolution of Leg Feathers.” Science 339. no. 6125 (15 March): 1309–1312.
Appendix
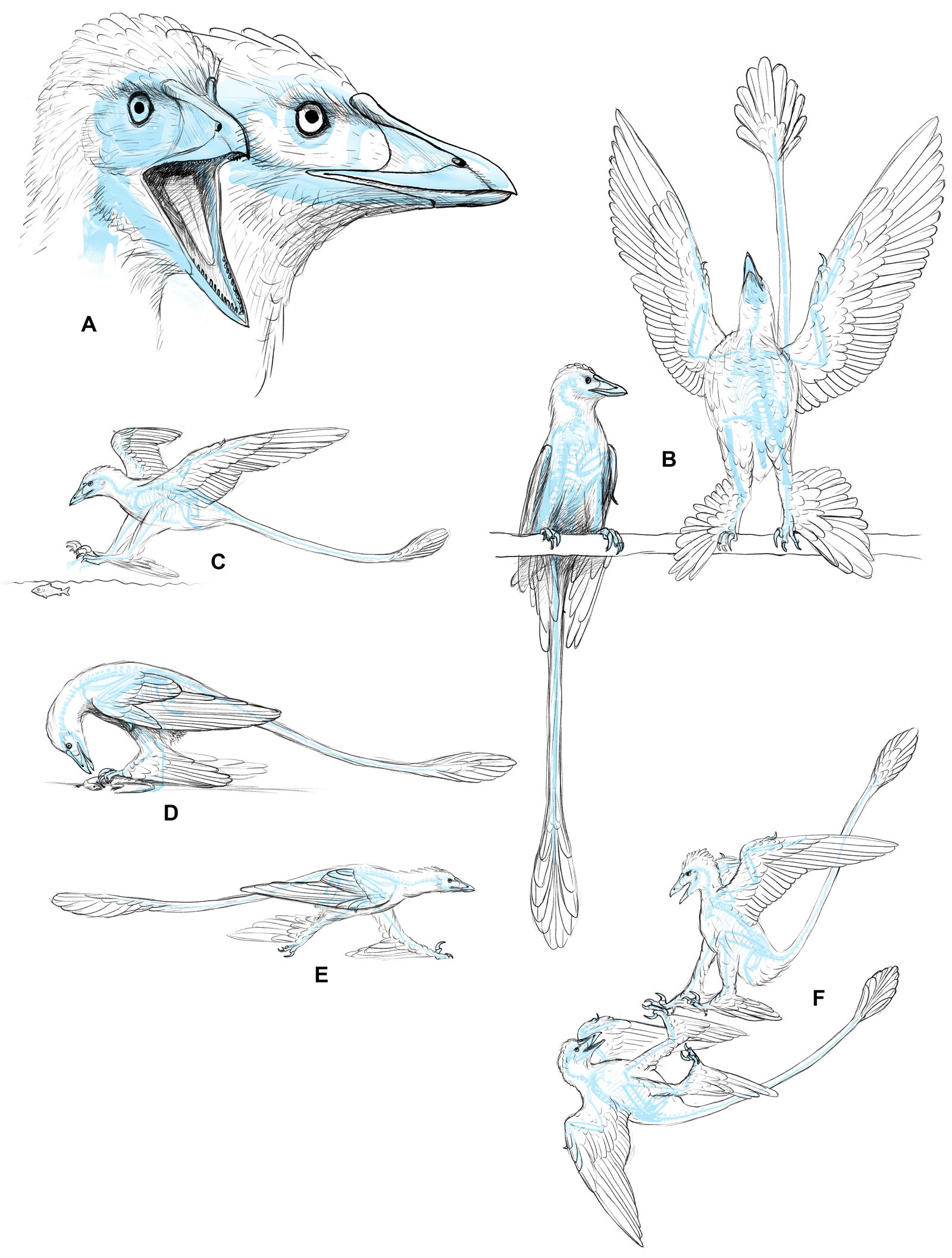
Supplementary fig. 1. Life reconstruction drawings of Microraptor in various poses with skeleton posed in blue. (A) Closeup of head from two angles showing possible beak shape and size. (B) Possible mating display performance by a male. (C) Hunting for a fish. (D) Eating a lizard. (E) Running. (F) Fighting in the air. Three-dimensional skeleton model posed using Blender and drawn over using Adobe Photoshop.
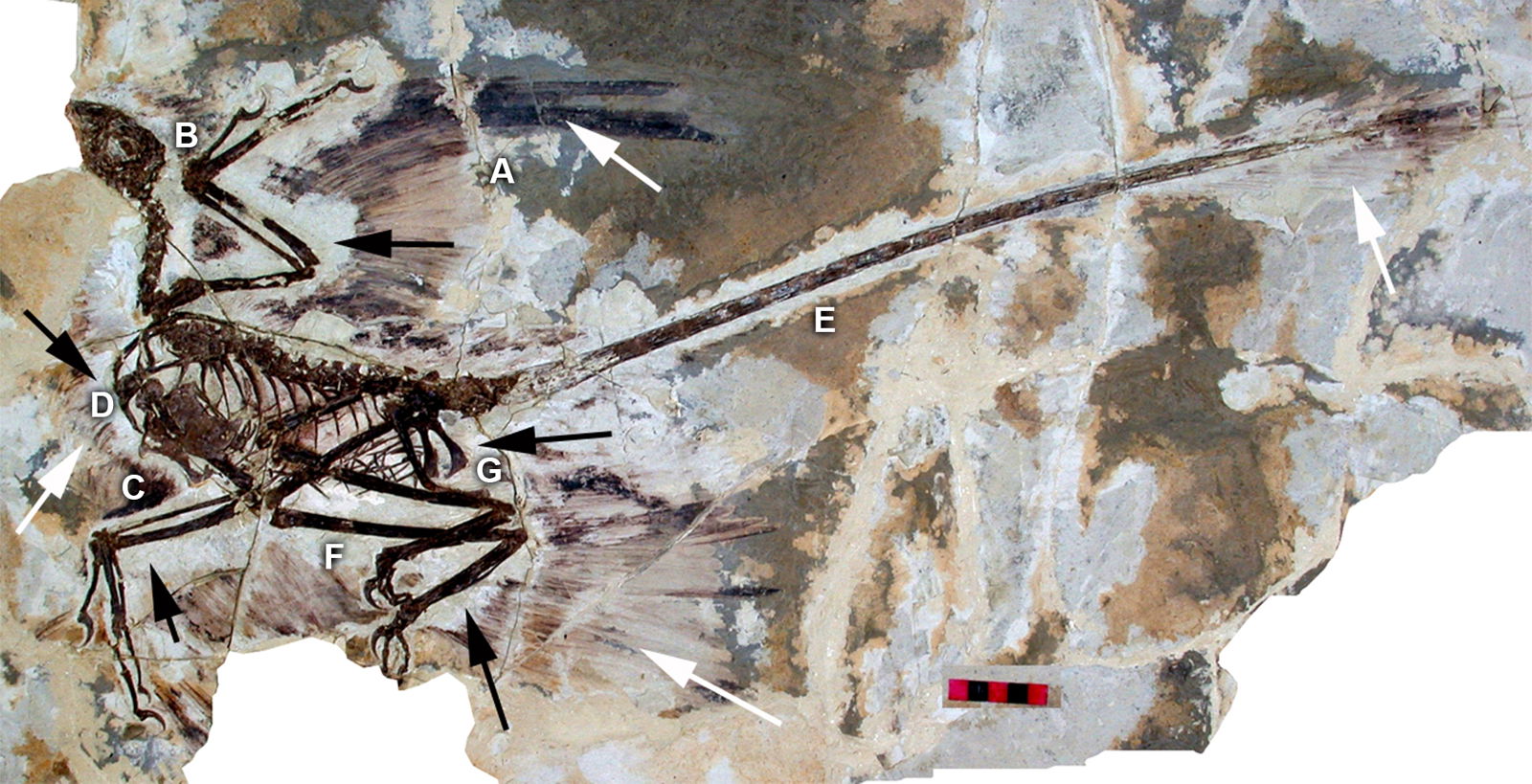
Supplementary fig. 2. Skeleton of Microraptor gui holotype (IVPP V13352) documented by Xu et. al. (2003). (A) Specimen shows multiple avian characteristics such as pennaceous wing feathers, (B) swivel wrist, (C) patagia tissue, (D) angled coracoid and large sternum, (E) thin tail with 30 caudals or less, (F) crouched legs for knee walking, and (G) rear facing pubic bones. David W. E. Hone, Helmut Tischlinger, Xing Xu, Fucheng Zhang “The holotype of Microraptor gui, IVPP V 13352 under normal light. This shows the preserved feathers (white arrow) and the ‘halo’ around the specimen where they appear to be absent (black arrows). Scale bar at 5 cm,” https://en.m.wikipedia.org/wiki/File:Microraptor_gui_holotype.png, CC BY 2.5 DEED.
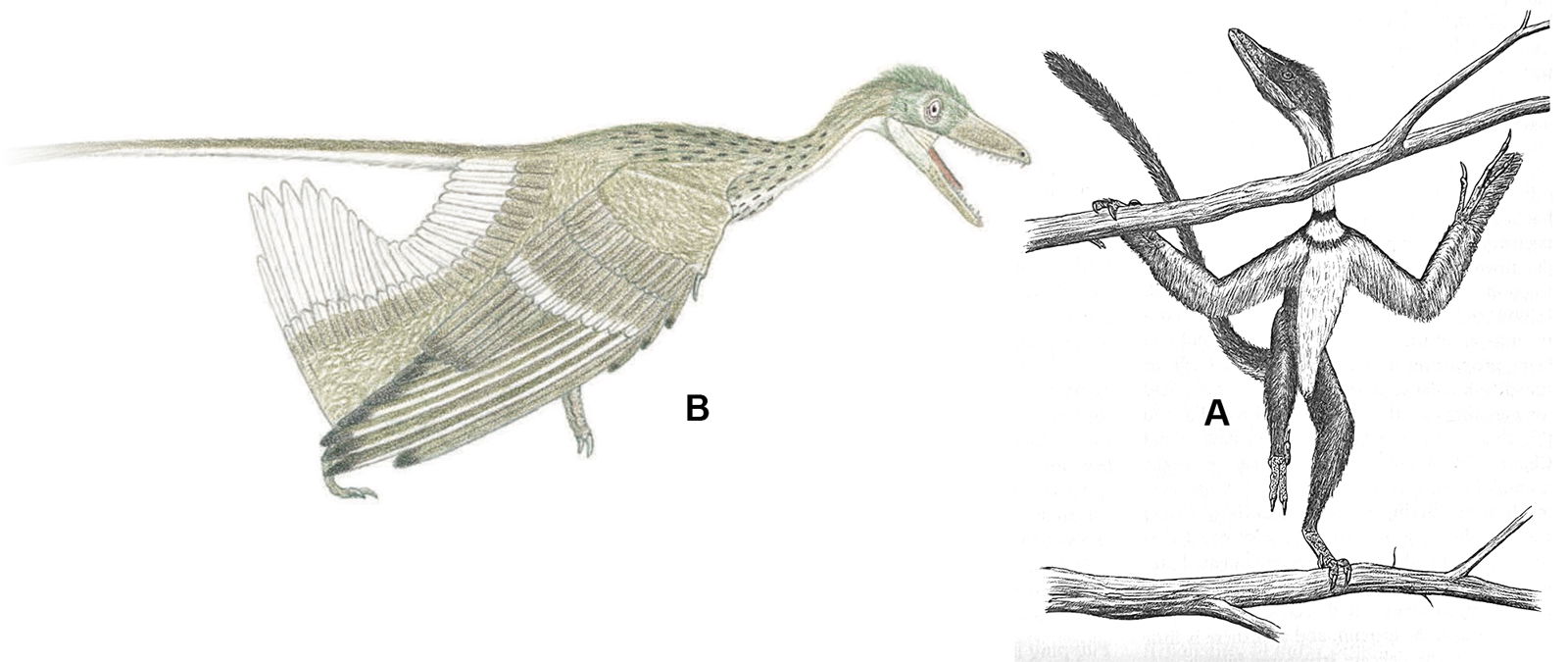
Supplementary fig. 3. Evolutionary life reconstructions often show an evolutionary bias by visualizing hypothetical features not found in the fossil. (A) Paul (2002) drew Sinornithosaurus with hypothetical “protofeathers” before winged Microraptor fossils were found. (B) Paul (2016) drew Sinornithosaurus with large wing feathers only after fellow dromaeosaur, Microraptor, was found with modern bird wings.
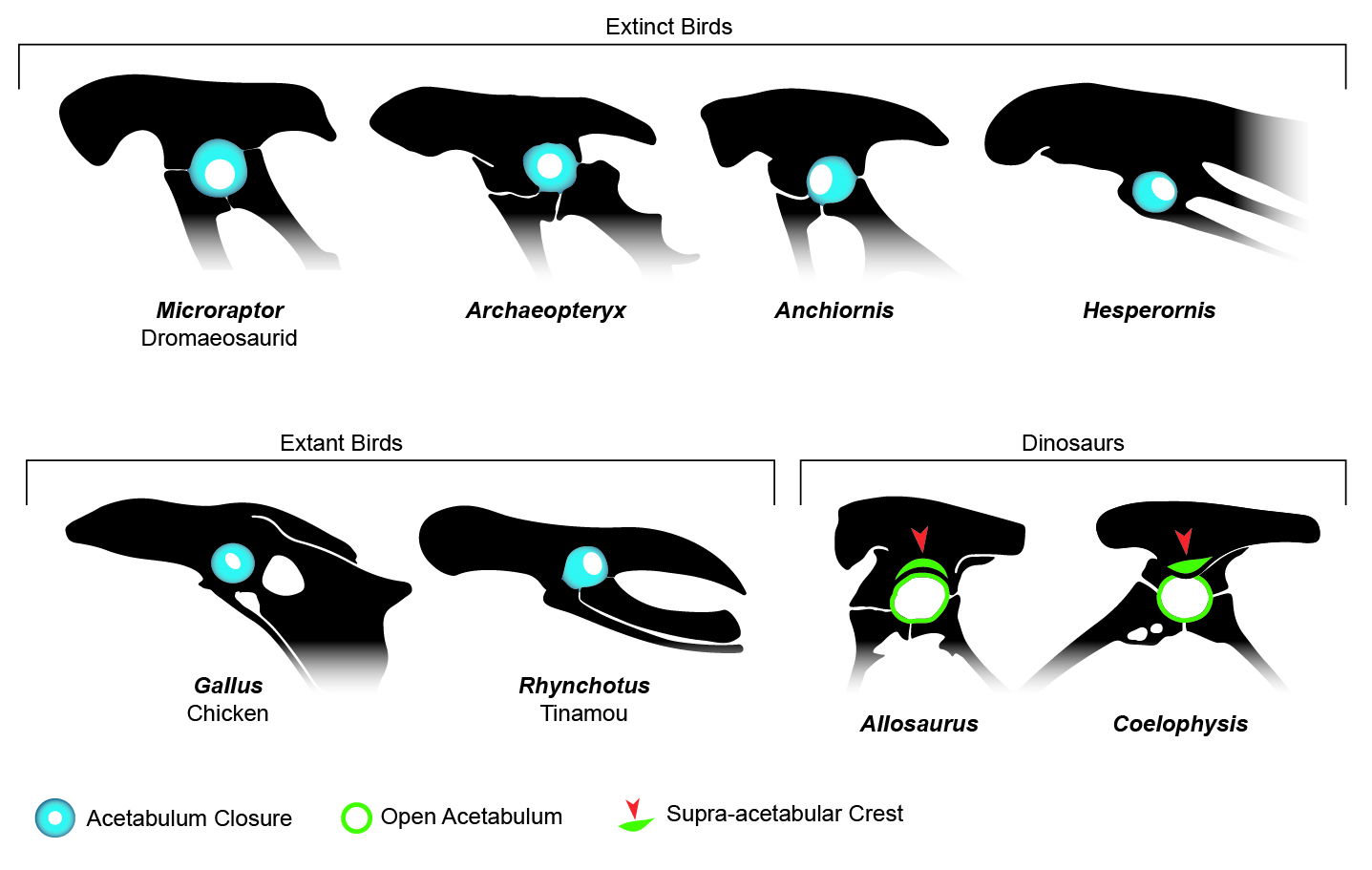
Supplementary fig. 4. Microraptor and other birds have no supraacetabular crest and often have some degree of medial occlusion of the acetabulum. Skeletal drawings adapted from Hartman (n.d.) reconstructions and Feduccia (2023). Individuals scaled to similar pelvis size.