The views expressed in this paper are those of the writer(s) and are not necessarily those of the ARJ Editor or Answers in Genesis.
Abstract
The cross-bedded Coconino Sandstone (lower Permian, Arizona) is often used as a “type” ancient eolian sandstone. Previous field and laboratory work by the author have revealed data that instead support a marine origin for this sandstone. Using primarily the COSUNA data compiled by the AAPG in the 1980s, Permian and Pennsylvanian sandstones were correlated across western and central United States with special emphasis on lower Permian formations. It was found that the Coconino could be correlated as a diachronous sand body from southern California to North Dakota, and from Texas to Idaho, an area of approximately 2.4 million km2. Formations included the Glorieta Sandstone (New Mexico, Texas, and Oklahoma), the Lyons Sandstone (Colorado), the Wood River Formation (Idaho), the Weber Formation (Utah), the Tensleep Sandstone and Casper Formation (Wyoming), the Minnelusa Formation (Montana, South Dakota) and the Broom Creek Formation (North Dakota). A literature review of these sandstones revealed many common characteristics with the Coconino including paleocurrent directions, the presence of dolomite and tetrapod footprints. Textural similarities were also recognized in the field and under the microscope including angular grains, poor to moderate sorting and mica. The sandstones are usually found below a chemically rich marine rock layer containing gypsum, phosphate, salt or limestone, and zircons show common Appalachian sources. A literature review of Permian cross-bedded sandstones in Canada, Europe, South America, and Saudi Arabia revealed similar characteristics to these western United States sandstones.
Several implications are discussed, including: 1) The similarities of other Permian cross-bedded sandstones to the Coconino imply that they are also likely marine sandstones. 2) Consistent paleocurrents are best explained by marine processes, not subaerial ones. 3) Provenance studies of the Coconino equivalents show a large portion of the sand originated from eastern North America. Combined with paleocurrent data, it appears a massive amount of sand was transported across the continent by marine currents and not by rivers or wind. 4) Some creationists have denied the validity of the geological column. While a single “blanket” sandstone is not a column, many “blankets” stacked on top of one another, are a column. 5) Similar Permian sandstones around the world not only strengthen the validity of the geological column, but they argue that these sandstones were also made by similar processes as the Coconino during a singular period of time.
Sand waves may not be an exact analogue of how the “blanket” of the Coconino and other sandstones were deposited, but we envisage something similar depositing shallow marine sands all over the Pangean supercontinent as it was underwater during the Flood.
Introduction
In the first chapter of his insightful book, The Nature of the Stratigraphical Record, Derek Ager (1981) discusses a concept which he refers to as the persistence of facies. What Ager means by this is that during certain periods of geologic time, specific facies inexplicably appear around the world over and over again. For example, he talks about the basal Cambrian sandstones, the Cretaceous chalks, the Permo-Triassic redbeds, the Carboniferous coals and the Mississippian limestones. Although Ager was clearly a catastrophist but not a creationist, he understood that his data and ideas could have implications for those who were looking for evidence to support Noah’s Flood (see p. 20 for example). This is because deposits like this imply similar events and processes occurring over widespread areas. Pennsylvanian-Permian-Triassic sandstones are a clear example of some of the persistent facies that can be found worldwide during this interval. A few examples would be the Coconino Sandstone of Arizona, the Tensleep Sandstone of Wyoming the Lyons Sandstone of Colorado, the Rotliegendes Sandstone of Germany, the Hopeman, Corrie, and Corncockle Sandstones of Scotland, the Penrith, Bridgnorth and Yellow Sands Sandstones of England, part of the Champenay Formation of France, the Pirambóia Formation of Brazil and the Andapaico Formation of Argentina (see Brookfield 1978; Clemmensen and Hegner 1991; Duncan 1830; Glennie 1972; Hurst and Glennie 2008; Karpeta 1990; Limarino and Spalletti 1986; Maithel, Garner, and Whitmore 2015; McKee and Bigarella 1979; Newell 2001; Steele 1983; Swezey, Deynoux, and Jeannette 1996; Waugh 1970a, 1970b). Conventional geology has usually explained the persistence of facies, not in terms of Ager’s catastrophism, but in terms of similar climatic conditions over widespread areas united by a common paleogeography (see Blakey and Ranney 2018, for example). In the case of the Pennsylvanian and Permian sandstones of the western United States, they are explained as desert sand dune deposits occurring on the western side of the Pangean continent. Or, if they have marine fossils, as many of them do, shallow marine sands proximal to the Pangean coast.
The Coconino Sandstone is one of the more well-known Permian sandstones of the western United States. It is part of familiar cliff exposures in places like Grand Canyon and Sedona, Arizona (fig. 1). It consists almost entirely of large cross-beds (fig. 2) composed of moderately- to poorly-sorted, subangular, fine-grained sand (fig. 3). Whitmore et al. (2014) gave a comprehensive report on the petrology of the formation showing that the commonly held beliefs that the Coconino contains well-rounded, well-sorted sand grains were false. Maximum thickness is reached near Pine, Arizona where it is about 300 m thick; in areas like the Grand Canyon, it averages around 90 m thick. The eminent Grand Canyon geologist, Edwin McKee was the first geologist to do an extensive study of the formation early in his career (McKee 1934). McKee maintained an eolian interpretation for the Coconino throughout his prestigious career (McKee and Bigarella 1979). The Coconino has become somewhat of a “type” example of what an ancient eolian sandstone is supposed to look like.
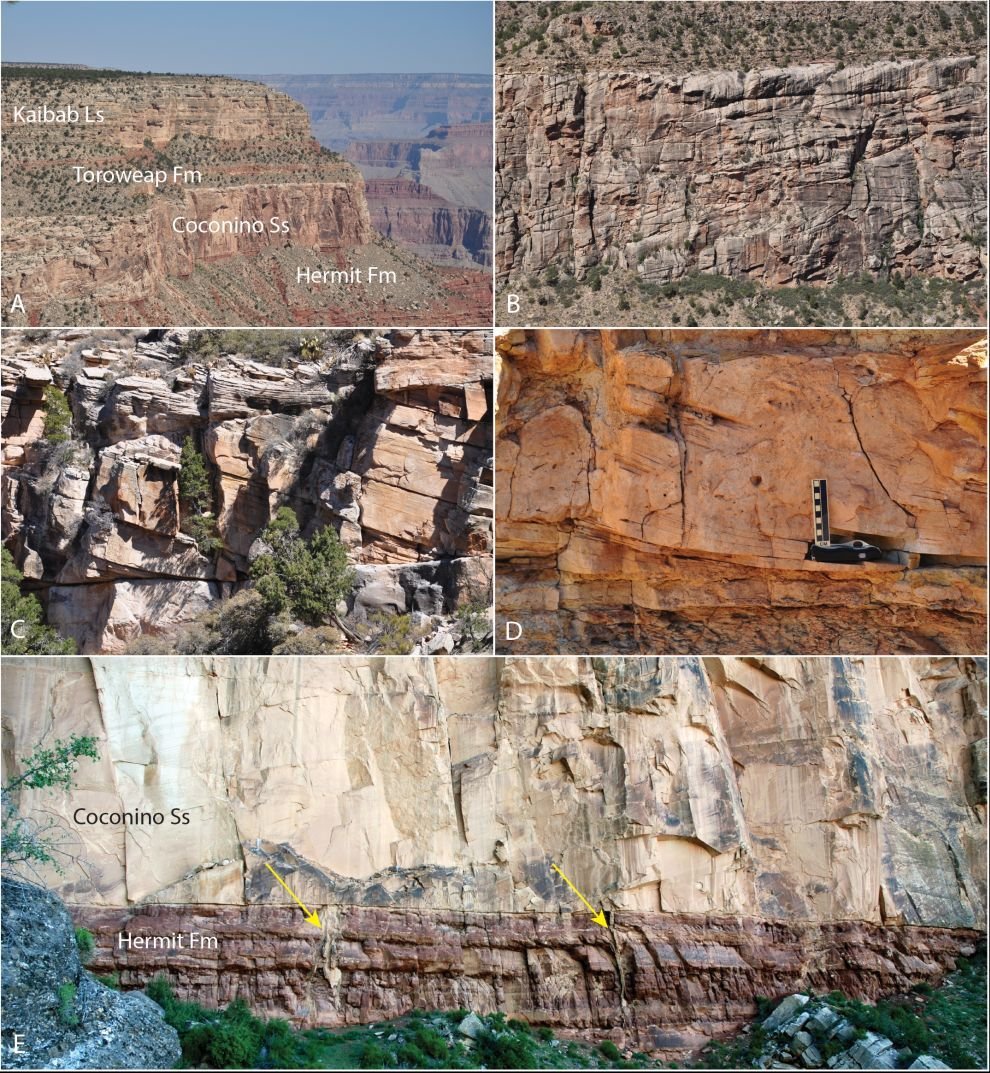
Figure 1. A–D. The Coconino Sandstone as it appears in Grand Canyon, Arizona, as viewed from Hermit Trail. The formation is about 100 m thick in this area. E. The Coconino Sandstone—Hermit Formation contact along Bright Angel Trail. Here, two large sand injectites are indicated by the yellow arrows. The one on the right penetrates about 15 m downward into the Hermit before it disappears into the talus where the vegetation begins. The Bright Angel Fault is just out of view on the left side of the photo.
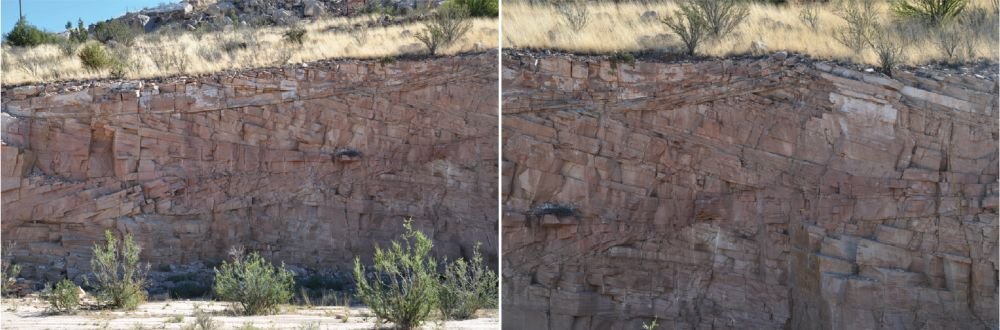
Figure 2. The large cross-beds typical of the Coconino Sandstone, Ash Fork, Arizona.
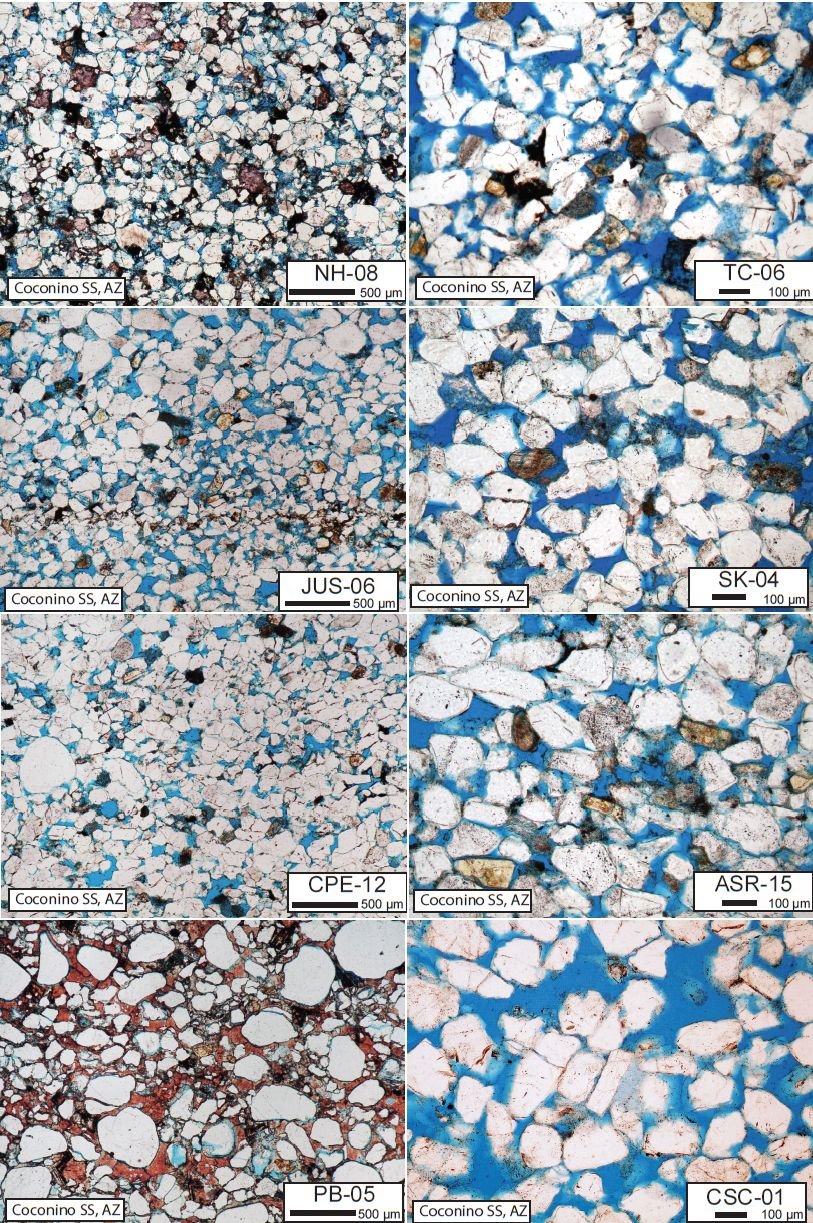
Figure 3. Typical Coconino Sandstone as seen under thin section. The sandstone is often poorly sorted and subangular. NH New Hance Trail, TC Trail Canyon, JUS Jumpup Spring, SK South Kaibab Trail, CPE Chino Point East, ASR American Sandstone Ridge, PB Picacho Butte, CSC Cave Spring Campground.
The goal of this particular project was to correlate the Coconino Sandstone from Arizona to surrounding western states. It is well known that many Pennsylvanian to lower Permian sandstones occur in this area; but understanding how they correlate with one another has sometimes proved difficult primarily because of their lack of fossils (see the discussion in Baars 1979 and Dunbar et al. 1960). The problems with correlation of Permian units not only occur in the western United States, they occur worldwide (Baars 1979). With that in mind, the lithological equivalents in this paper should be seen as general trends based on contained fossils (which are sparse), presumed conventional age, and the presence of these sandstone formations often directly below rocks of chemical origin (most commonly limestone, dolomite, gypsum, salt, and phosphate). At their edges, these sandstones often change in character quickly and often grade into various siltstones, mudstones, limestones, dolomites, and rocks of chemical origin. One should not interpret the sandstones contained within the maps and cross-sections of this paper as identical in nature to the Coconino. The Coconino is an end member of a continuum from a relatively pure cross-bedded quartz arenite to planar-bedded feldspathic sandstones with diverse mineralogies and intervening silts and muds.
Because the data were primarily gathered from AAPG’s (American Association for Petroleum Geology) COSUNA (Correlation of Stratigraphic Units of North America) project for the correlations (where each column in the charts is only a generalization of the stratigraphy in any one particular area), this project is only an attempt at correlating the sandstone units across the western United States. More detailed columns that are spaced closer and show the detailed stratigraphy could be gathered in the future for an extension of this project. However, this project will show there are many close relationships between the Pennsylvanian and Permian sandstones of the western United States.
Many of the relationships shown in this paper have been known for years (see for example, Blakey, Peterson, and Kocurek 1988; Dunbar et al. 1960; Mallory 1972b; McKee and Oriel 1967; Rascoe and Baars 1972). This paper attempts to:
- include some further data not mentioned in these other projects,
- show the units are widespread across the western United States as a single lithostratigraphic sand body, where other projects have simply showed relationships of sandstones mostly across state borders,
- demonstrate the consistency and importance of paleocurrents in these formations,
- interpret the results within a catastrophic framework, and
- compare the western USA sandstones with some sandstones in South America and Europe.
- Finally, a correlation project like this clearly strengthens the reality of the geological column over the area covered.
Some creationists have denied the reality of the global geological column (Oard 2010a, 2010b; Reed and Froede 2003; Woodmorappe 1981). But patterns documented in this paper and in larger projects like COSUNA, and in Clarey’s intercontinental correlations (Clarey and Werner 2018), clearly demonstrate the reality of the column, despite claims otherwise.
Methods
Stratigraphic charts and electronic data sheets compiled for the COSUNA project by the AAPG in the 1980s were used to gather the primary data for this project (Adler 1986; Ballard, Bluemle, and Gerhard 1983; Bergstrom and Morey 1984; Hills and Kottlowski 1983; Hintze 1985; Kent, Couch, and Knepp 1988; Mankin 1986). Sixty-four columns from the COSUNA data and some other sources (Adkison 1966; Dunbar et al. 1960; Hintze 1988; Langenheim and Larson 1973; Maher 1960; Mallory 1972b; McKee and Oriel 1967; Stokes 1986) were drafted for stratigraphic correlation.
Often charts from sources like this are time-stratigraphic, so these columns were redrawn to show their lithostratigraphic characteristics and rock thicknesses (instead of time duration). Within the drafted columns, lithology is shown in two different ways: by color and by symbol. Colors represent general overall lithology (yellow = sandstone, for example) and the symbols illustrate the more varied lithology and sedimentology that might occur within a particular formation. When numerous lithologies occurred in a single formation, only a generalized section was drawn to illustrate the varied facies present, as in columns 63 and 64 (Nevada) for the Pequop Formation. Columns focus on sandstones conventionally assigned to Pennsylvanian through early Permian age. Several units above and below the sandstones in this age range were usually included in the columns. To gain confidence in the correlations, not only were the lithologies and conventional “stages” of the rocks considered, but gypsum-carbonate beds, or other chemically rich units just above the sandstones, were used as marker beds and were correlated across the area as well. Priority was given to lithostratigraphic correlation over chronostratigraphic correlation when a choice needed to be made between the two. A table that compares North American and global chronostratigraphic units in use when the COSUNA project was published, with currently recognized international standards (International Commission on Stratigraphy) can be found in the Appendix 1. For consistency, chronostratigraphic stage names used with the COSUNA project (Childs et al. 1988), and almost exclusively used in the literature gathered for this project, were maintained. No attempt was made to make conversions between the two conventions because some of the differences are quite large. The stratigraphic columns were “hung” on the Pennsylvanian-Permian boundary. Some of the units were examined in the field (fig. 4) and sampled during previous studies by the current author (Whitmore et al. 2014; Whitmore and Garner 2018) and his team. Primary literature on global Permian sandstone units was collected, but more attention was paid to those in the western United States. Cross-bed dips and azimuths for some of these formations were measured in the field and others were collected from various sources in the literature (Brand, Wang, and Chadwick 2015; Knight 1929; Lawton, Buller, and Parr 2015; Opdyke and Runcorn 1960; Peterson 1988; Poole 1962; Reiche 1938).
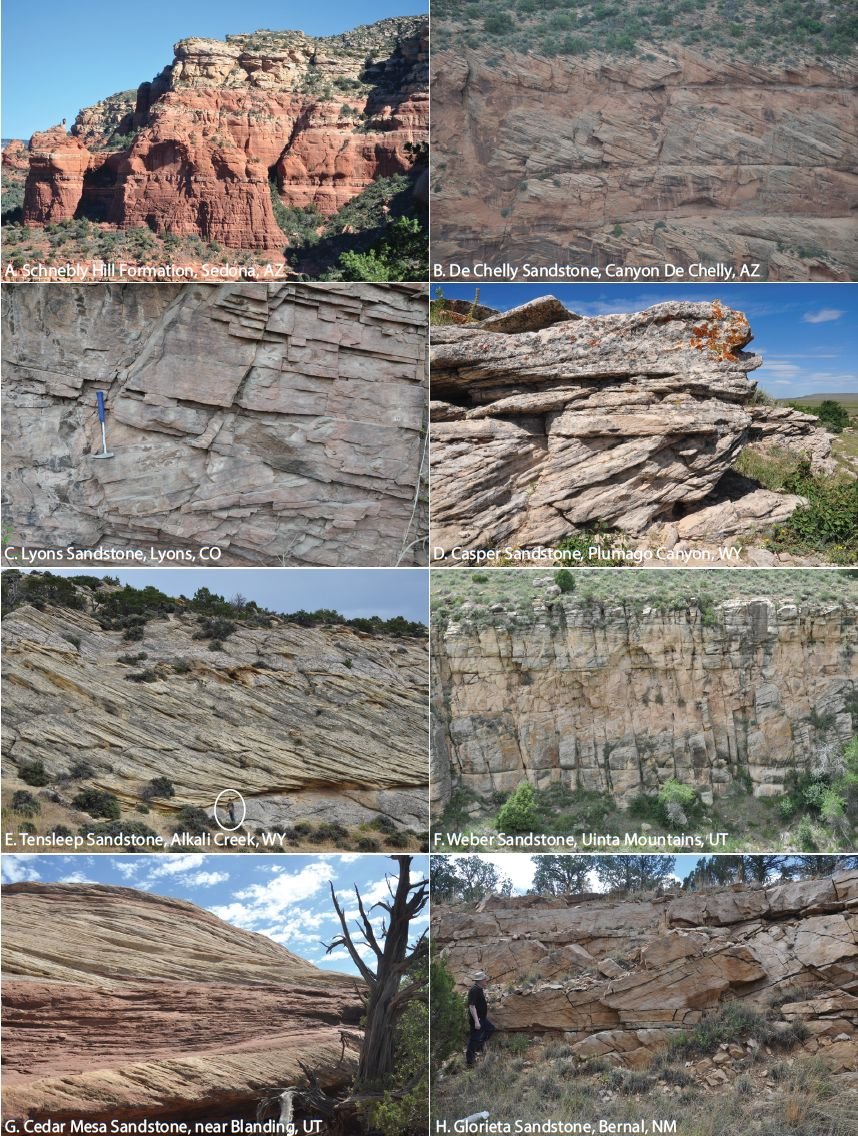
Figure 4. Outcrops of some of the sandstones referenced in this paper. A. Schnebly Hill Formation, Sedona, Arizona. The Schnebly Hill Formation is the reddish unit; the Coconino is lighter colored unit near the top of the cliff. The two units have a transitional contact. The cliff is about 125 m high. B. De Chelly Sandstone, Canyon De Chelly, Arizona. The thickest cross-bed set is about 15 m thick. C. Lyons Sandstone, Lyons, Colorado. Rock hammer for scale. D. Casper Sandstone, Plumago Canyon, Wyoming. The exposure is about 2 m high. E. Tensleep Sandstone, Alkali Creek, Wyoming. Ten-year-old boy for scale. F. Weber Sandstone, Uinta Mountains, Utah. The thicker cross-bed sets are about 10 m thick. G. Cedar Mesa Sandstone near Blanding, Utah. Red planar-bedded set is about 1 m thick. H. Glorieta Sandstone, Bernal, New Mexico. Paul Garner for scale.
Results
It was found that the Coconino could be correlated with sandstones occurring on both the east and west sides of the Rocky Mountains and into the Great Basin of California, Nevada, and Utah. Units could be traced from California to the Dakotas and from Texas to Idaho (fig. 5). Outcrops were absent in the general area of the Ancestral Rocky Mountains (Uncompahgre Uplift). Some details on many of the correlated sandstone units are reported in Appendix 2. Details on some other sandstone units in Canada, South America, Europe and Saudi Arabia are reported in Appendix 3. Eastern European sandstones could potentially be correlated with each other in a separate project. Cross-bed dips for some of the formations are illustrated in fig. 6. Paleocurrent patterns for some of these sandstones are shown in fig. 7. Paleocurrent directions for most Pennsylvanian and Permian sandstones in the United States are consistently to the south (fig. 7). Detrital zircon studies have been completed on some of these formations showing that much of the sand for these formations originated in the Appalachian area; those results are discussed below.
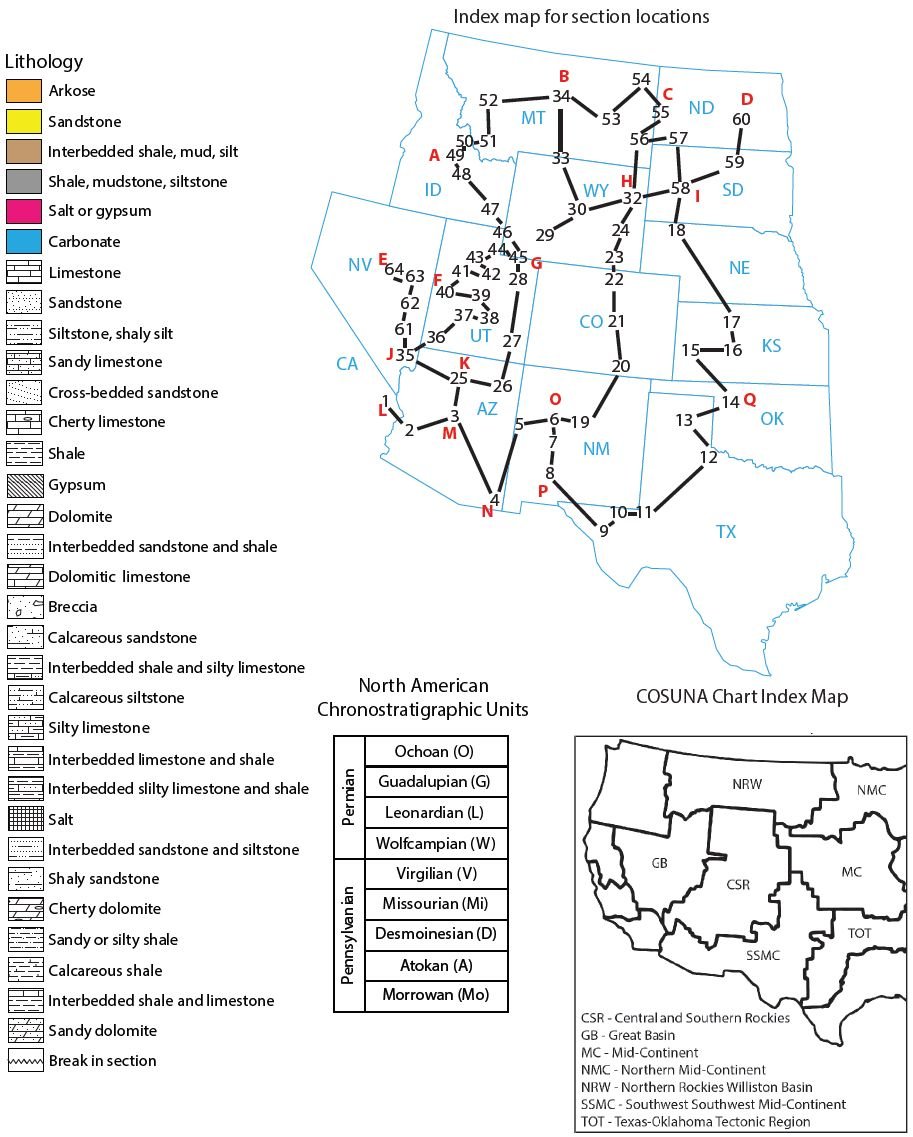
Figure 5A. Correlation of Pennsylvanian and Permian sandstones in the western United States. Legend showing lithology colors and symbols used in the correlations. Correlation map showing the physical location of the sections. North American Chronostratigraphic Units used in the correlations (also see Appendix 1). COSUNA chart index map and abbreviations.
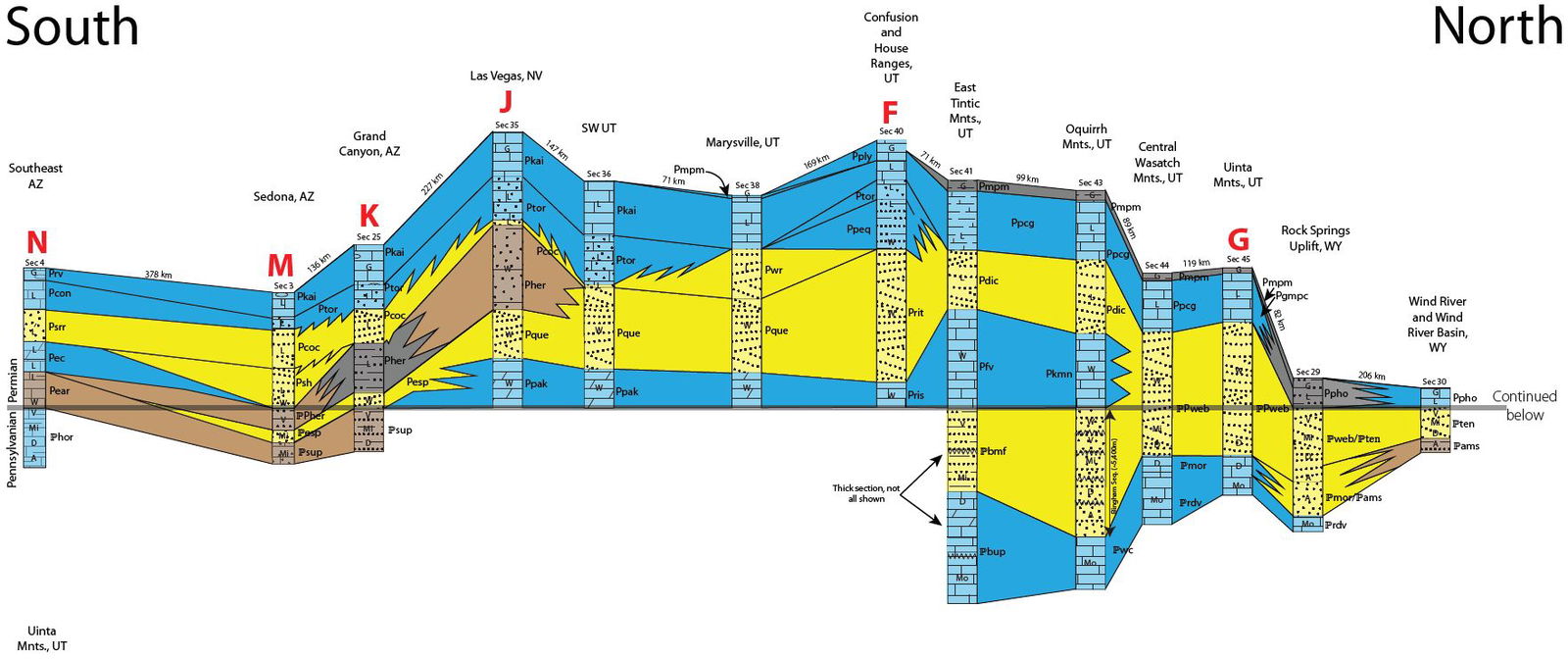
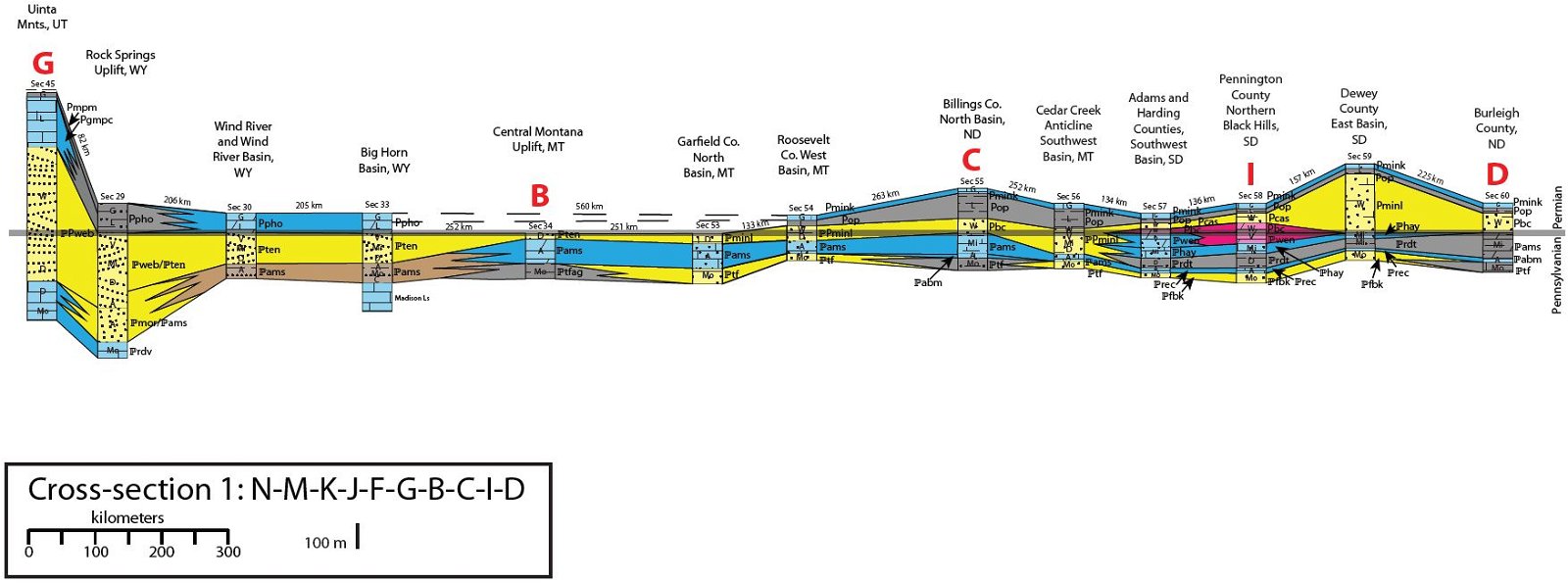
Figure 5B. Cross-section 1: N-M-K-J-F-G-B-C-I-D (letters and column numbers on map in Fig. 5A).
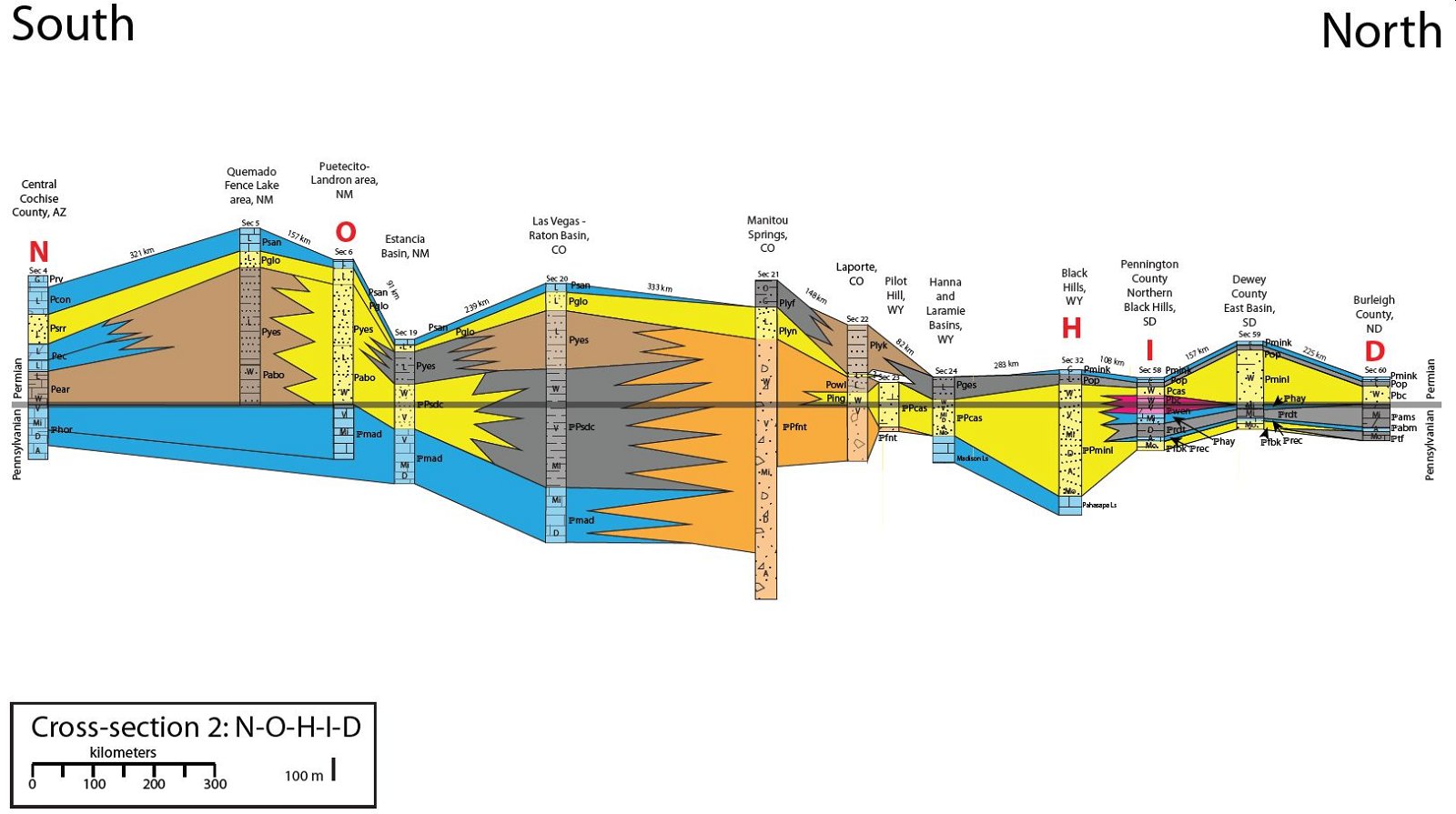
Figure 5C. Cross-section 2: N-O-H-I-D (letters and column numbers on map in Fig. 5A).
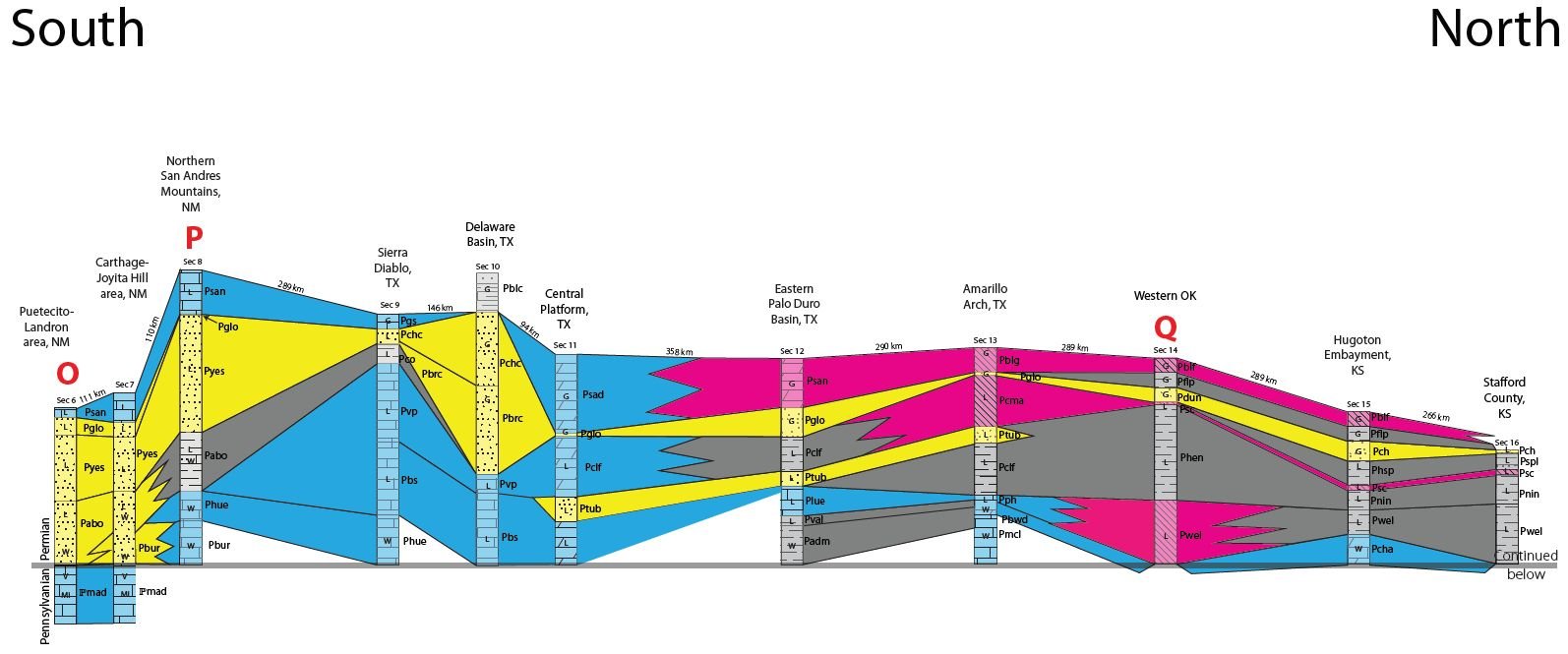
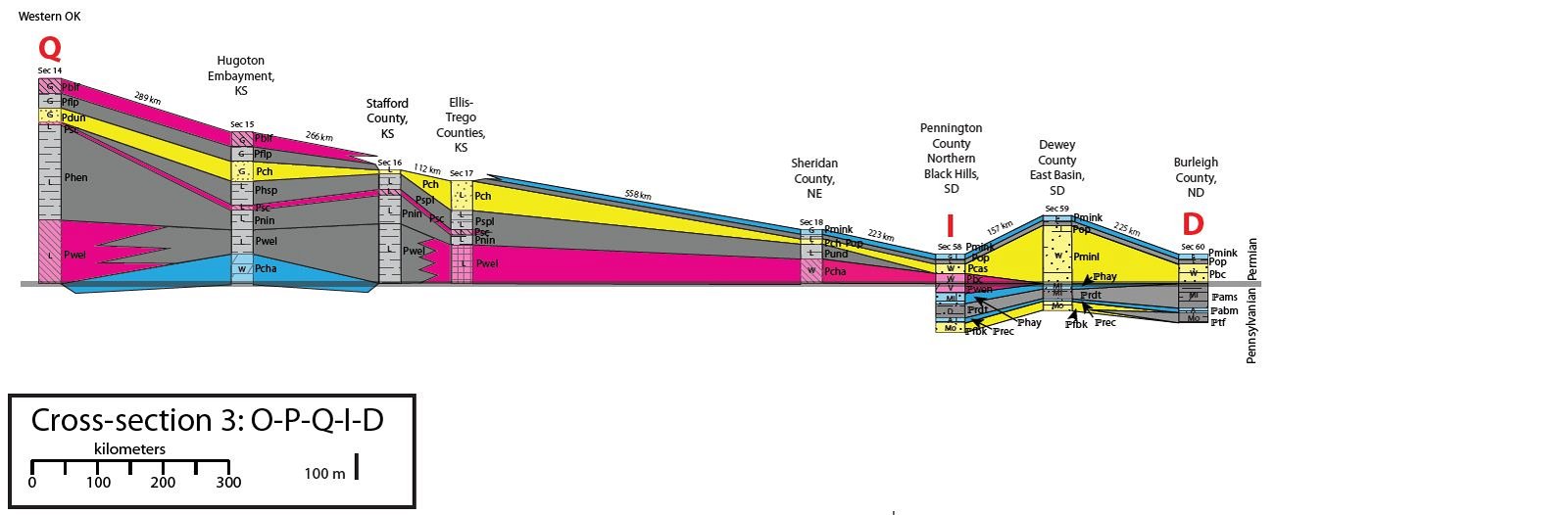
Figure 5D. Cross-section 3: O-P-Q-I-D (letters and column numbers on map in Fig. 5A).
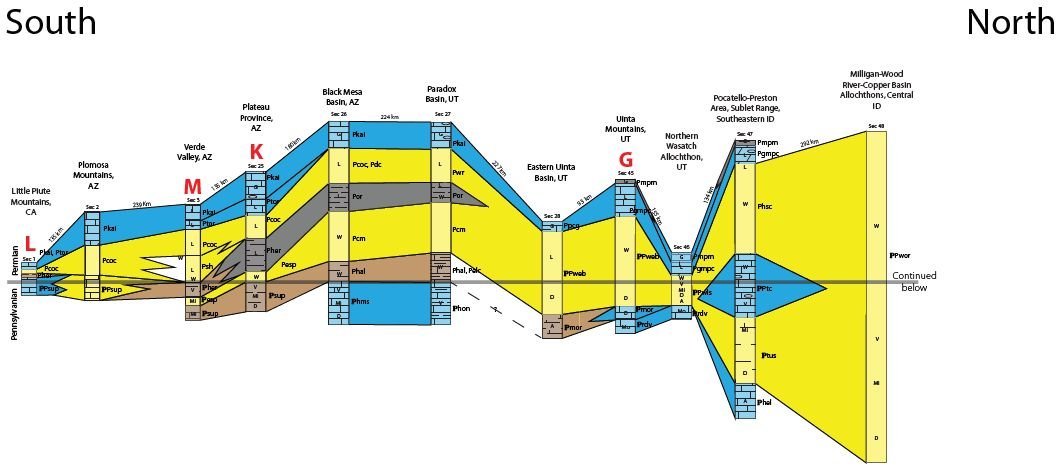
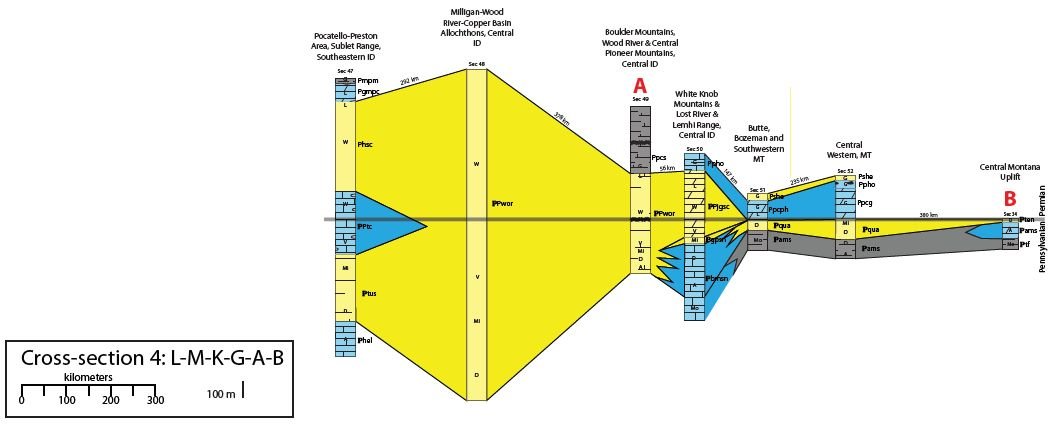
Figure 5E. Cross-section 4: L-M-K-G-A-B (letters and column numbers on map in Fig. 5A).
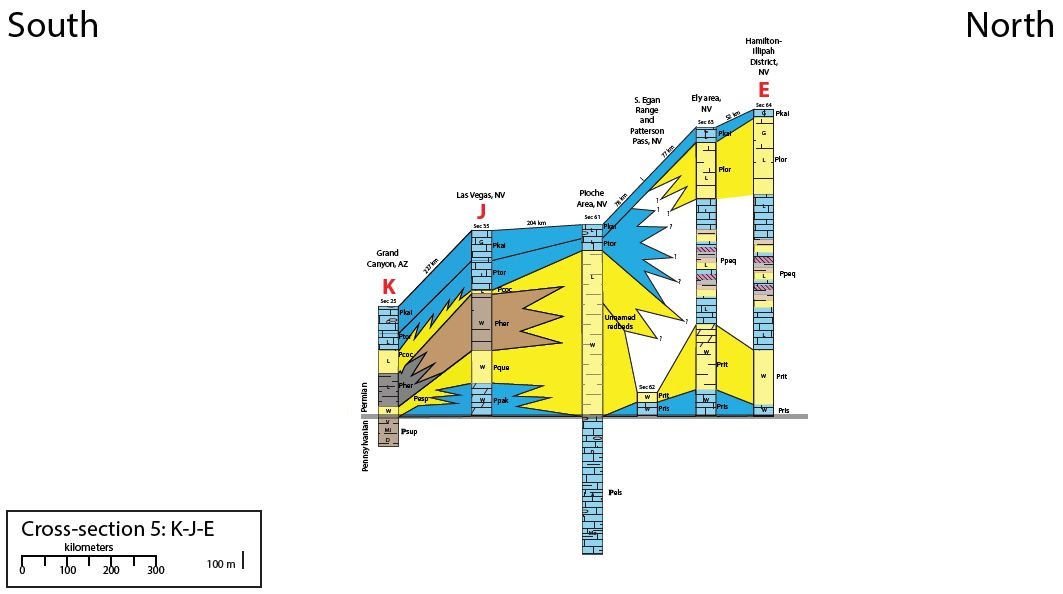
Figure 5F. Cross-section 5: K-J-E (letters and column numbers on map in Fig. 5A)
Permian (P) Units
- Pabo – Abo Fm
- Padm – Admiral Fm
- Pbc – Broom Creek Fm
- Pblc – Bell Canyon Fm
- Pblf – Blaine Fm
- Pblg – Blaine Gyp
- Pbrc – Brushy Canyon Fm
- Pbs – Bone Spring Ls
- Pbur – Bursum Fm
- Pbwd – Brown and White Dol
- Pcas – Cassa Fm
- Pch – Cedar Hills Fm
- Pcha – Chase Grp
- Pchc – Cherry Canyon Fm
- Pclf – Clear Fork Grp
- Pcm – Cedar Mesa Ss
- Pcma – Cimarron Anh
- Pco – Cutoff Sh
- Pcoc – Coconino Ss
- Pcon – Concha Ls
- Pdc – De Chelly Ss
- Pdic – Diamond Creek Ss
- Pdun – Duncan Ss
- Pear – Earp Fm
- Pec – Epitaph Fm and Colina Ls
- Pelc – Elephant Canyon Fm
- Pflp – Flowerpot Sh
- Pfv – Furner Valley Ls
- Pges – Goose Egg Fm and Satanka Sh
- Pglo – Glorieta Ss
- Pgmpc – Grandeur Mbr of Park City Grp or Fm
- Pgs – Goat Seep Ls
- Phal – Halqaito Fm
- Phen – Hennessey Sh
- Pher – Hermit Fm
- Phsc – Hudspeth Cutoff Fm
- Phsp – Harper Salt Plain Fm
- Phue – Hueco Fm
- Ping – Ingleside Fm
- Pkai – Kaibab Fm
- Pkmn – Kirkman Ls
- Pkmn – Kirkman Ls
- Plor – Loray Fm
- Plue – Lueders Ls
- Plyf – Lykins Fm and Forelle Ls
- Plyk – Lykins Fm
- Plyn – Lyons Ss and Satanka Sh
- Pmcl – Moore County Ls
- Pmink – Minnekahta Ls
- Permian (P) units, cont.
- Pminl – Minnelusa Fm
- Pmpm – Meade Peak Mbr of Phosphoria Fm
- Pnin – Ninnescah Sh
- Pop – Opeche Sh
- Por – Organ Rock Sh
- Powl – Owl Canyon Fm
- Ppak – Pakoon Ls
- Ppcg – Park City Grp or Fm
- Ppcph – Park City and Phosphoria Fm
- Ppcs – Pole Creek Sequence
- Ppeq – Pequop Fm
- Pph – Panhandle Ls
- Ppho – Phosphoria Fm
- Pply – Plympton Fm
- Pque – Queantoweap Ss
- Pris – Riepe Spring Ls
- Prit – Riepetown Ss or Fm
- Prv – Rain Valley
- Psad – San Andres Dol
- Psan – San Andres Fm
- Psc – Stone Coral Fm
- Psh – Schnebly Hill Fm
- Pshe – Shedhorn Fm
- Pspl – Salt Plain Fm
- Psrr – Scherrer Fm
- Ptor – Toroweap Fm
- Ptub – Tub Ss Mbr of Clear Fork Grp
- Pund – undifferentiated
- Pval – Valera Fm
- Pvp – Victoria Peak Ls
- Pwel – Wellington Fm
- Pwr – White Rim Ss
- Pyes – Yeso Fm
Permian/Pennsylvanian (ℙP) Units
- ℙPcas – Casper Fm
- ℙPfne – Fountain and Ingleside
- Fms
- ℙPfnt – Fountain Fm
- ℙPjgsc – Juniper Gulch Member of Snaky Canyon Fm
- ℙPminl – Minnelusa Fm
- ℙPsdc – Sangre de Cristo Fm
- ℙPsup – Supai Fm
- ℙPtc – Trail Canyon Fm
- ℙPweb – Weber Fm
- ℙPwls – Wells Fm
- ℙPwor – Wood River Fm
Pennsylvanian (ℙ) Units
- ℙabm – Alaska Bench Mbr of Amsden Fm
- ℙams – Amsden Fm
- ℙbmf – Bingham Mine Fm
- ℙbmsn – Bloom Mbr, Snaky Canyon Fm
- ℙbup – Butterfield Peaks Fm
- ℙesp – Esplanade Ss
- ℙels – Ely Ls
- ℙfbk – Fairbank Fm
- ℙfnt – Fountain Fm
- ℙgpsn – Gallagher Peak Ss, Snaky Canyon Fm
- ℙhay – Hayden Fm
- ℙhel – Helgar Canyon Fm
- ℙhms – Hermosa Fm or Grp, undivided
- ℙhon – Honaker Trail Fm
- ℙhor – Horquilla Ls
- ℙmad – Madera Ls
- ℙminl – Minnelusa Fm
- ℙmor – Morgan Fm
- ℙqua – Quadrant Ss
- ℙrdt – Roundtop Fm
- ℙrdv – Round Valley Ls
- ℙrec – Reclaimation Fm
- ℙsdc – Sangre de Cristo Fm
- ℙsup – Supai Fm or Grp
- ℙten – Tensleep Fm
- ℙtf – Tyler Fm
- ℙtfag – Tyler Fm of Amsden Grp
- ℙtus – Tussing Fm
- ℙwc – West Canyon Ls
- ℙweb – Weber Fm
- ℙwen – Wendover Fm
Fig. 5G. Stratigraphic names and abbreviations used in columns.
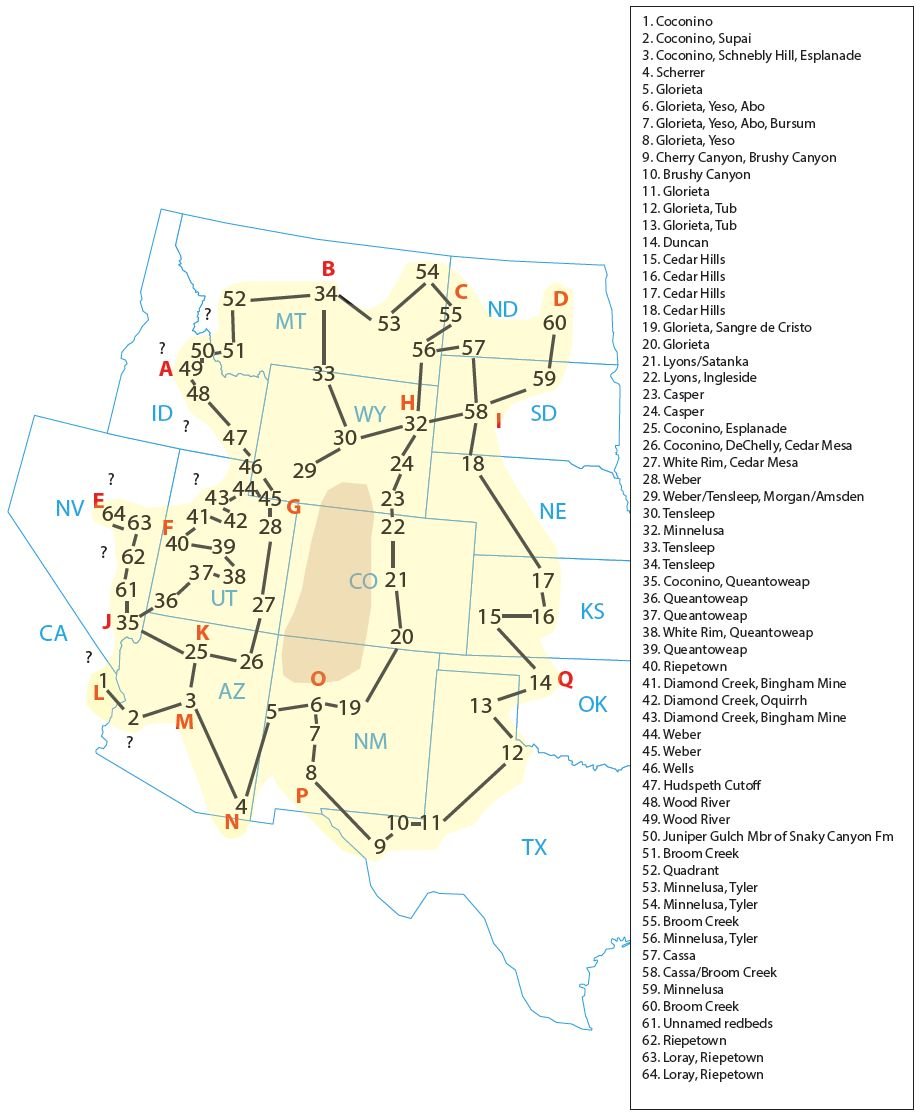
Figure 5H. Areal extent of the Coconino Sandstone and its equivalents in the western United States.
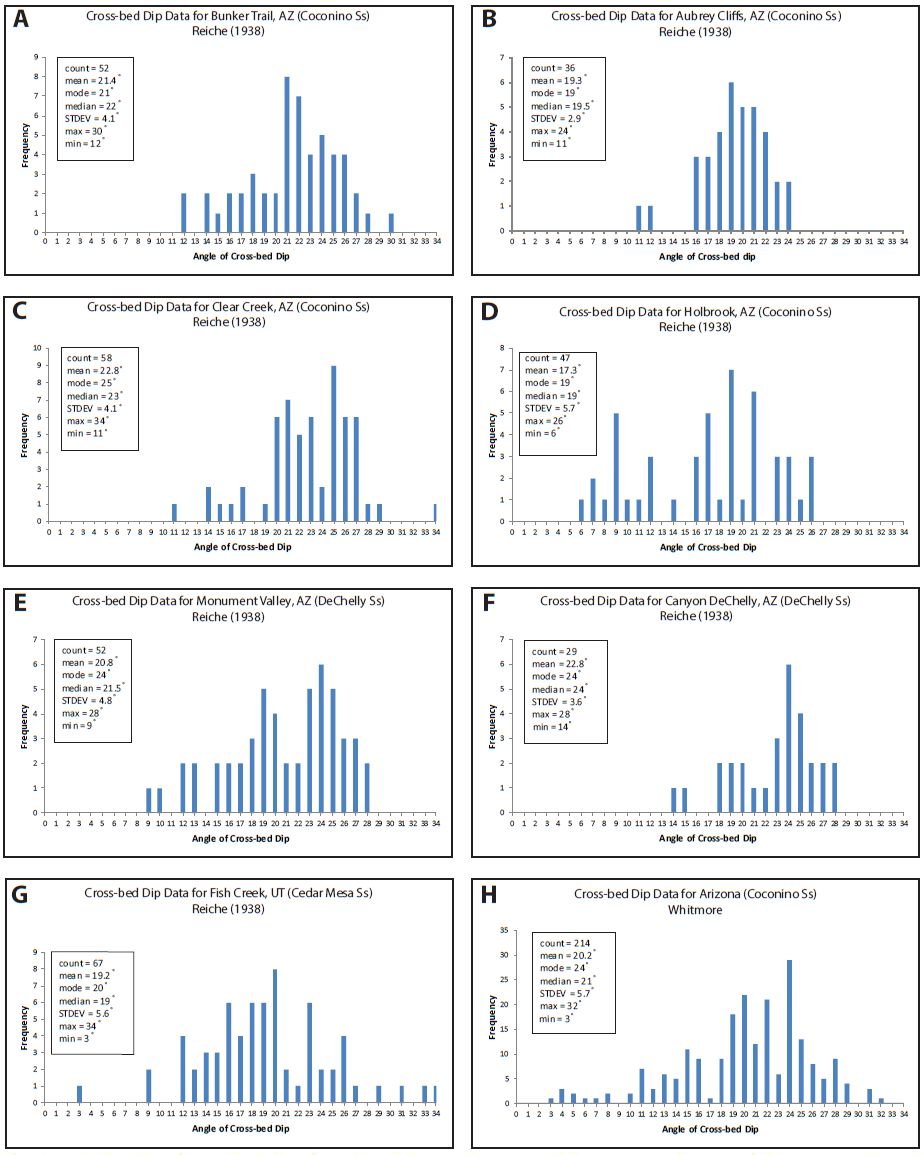
Figure 6 A–H. Graphs of cross-bed dips for selected Pennsylvanian and Permian sandstones of the western United States. Some data are plotted differently because of the way it is presented in the cited literature.
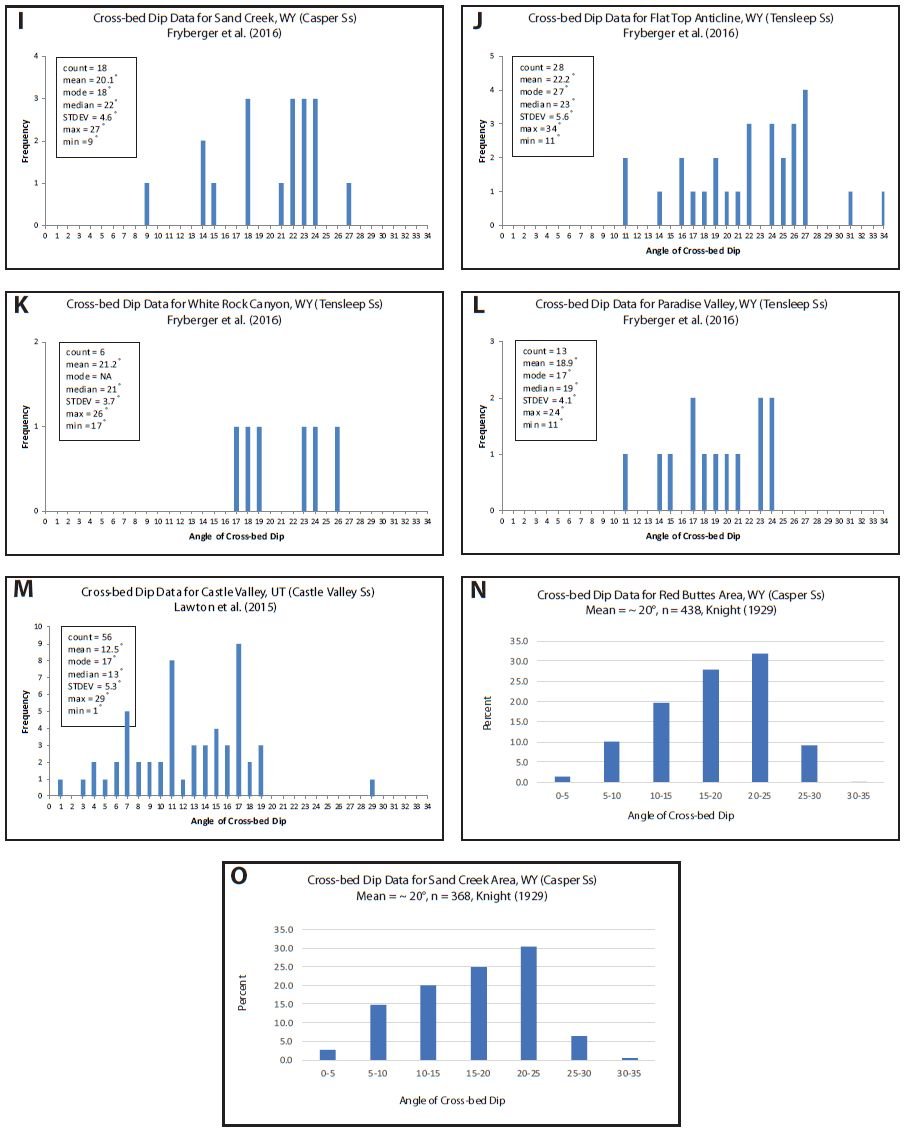
Figure 6 I–O. Graphs of cross-bed dips for selected Pennsylvanian and Permian sandstones of the western United States. Some data are plotted differently because of the way it is presented in the cited literature.
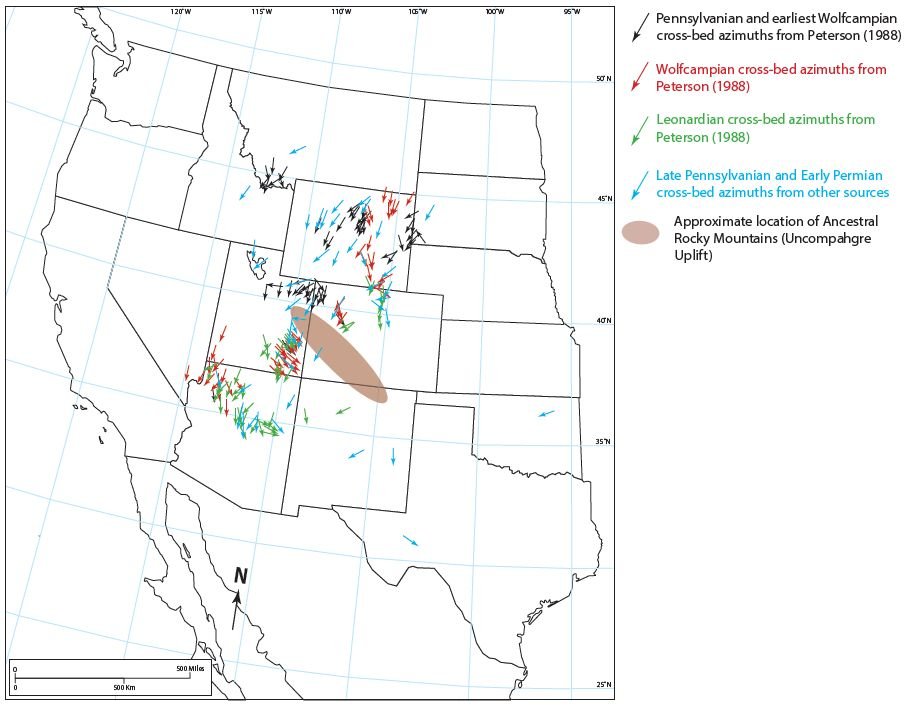
Figure 7. Paleocurrent patterns of Pennsylvanian and Permian sandstones of the western United States. See text for data citations.
Discussion
1. Depositional environment of the sandstones
The author has been part of a team that has been studying the Coconino Sandstone. In our studies we have examined many dozens of outcrops, have collected hundreds of samples (cutting corresponding thin sections for microscope work), and have studied numerous samples with XRD (X-ray diffraction) and SEM (scanning electron microscopy). In the summary of our findings (Whitmore and Garner 2018) and in other papers (Whitmore et al. 2014, 2015) we have presented numerous evidences that the Coconino is not an eolian deposit, but clearly a marine sandstone. Data that we have presented in support of a subaqueous origin of the Coconino includes:
- The sandstone is only poorly- to moderately-sorted and is only occasionally well-sorted,
- the grains of the Coconino can best be described as subangular to subrounded,
- K-feldspar is a common accessory mineral and is often more angular than the quartz, despite it being softer on Mohs Scale (see Whitmore and Strom 2017, 2018),
- muscovite, despite being extremely soft, was present in almost every thin section and our experimental results show that it disappears quickly in simulated eolian settings (Anderson, Struble, and Whitmore 2017),
- features resembling primary current lineation (parting lineation) are commonly found on most foreset surfaces,
- avalanche tongues, a feature common on steep slopes of eolian sand dunes, are missing in the Coconino; instead laminae can be followed in a straight line along exposed bounding surfaces,
- cross-bed dips average about 20° and our hundreds of measurements agree with hundreds of other measurements made by Reiche (1938) and Maithel (2019),
- large folds similar to parabolic recumbent folds (which are penecontemporaneous with cross-bed formation and cannot form from slumping dunes) are present in several places in the Sedona area,
- dolomite (we believe it is primary) occurs in many places and forms in the Coconino including as beds, ooids, clasts, rhombs and cement, and
- the expected sedimentary details commonly found in eolian sands (as far as grainfall, grainflow and ripple structures [Hunter 1977, 1981]) are for the most part absent or have been difficult to recognize in the Coconino (Maithel 2019).
- Outside of our work, Brand (Brand 1979; Brand and Tang 1991) has reported compelling evidence from vertebrate trackways in the Coconino, whose characteristics seem to be best explained by underwater origin.
Although we have not studied the other sandstones correlated in this paper to the degree that we have studied the Coconino, similar features are present in many of them that also point toward a subaqueous origin. We describe muscovite and angular K-feldspars in some of these sandstones in the United States along with Permian sandstones in the United Kingdom (Borsch et al. 2018; Maithel, Garner, and Whitmore 2015; Whitmore and Strom 2018). Fig. 8 illustrates just a small sampling of the mica and angular K-feldspar present in these sandstones. Mica is an indicator of aqueous processes because it deteriorates in a matter of days with continuous eolian activity (Anderson, Struble, and Whitmore 2017) and angular K-feldspars are important because they become quickly rounded only with hundreds of meters of eolian transport (Whitmore and Strom 2017).
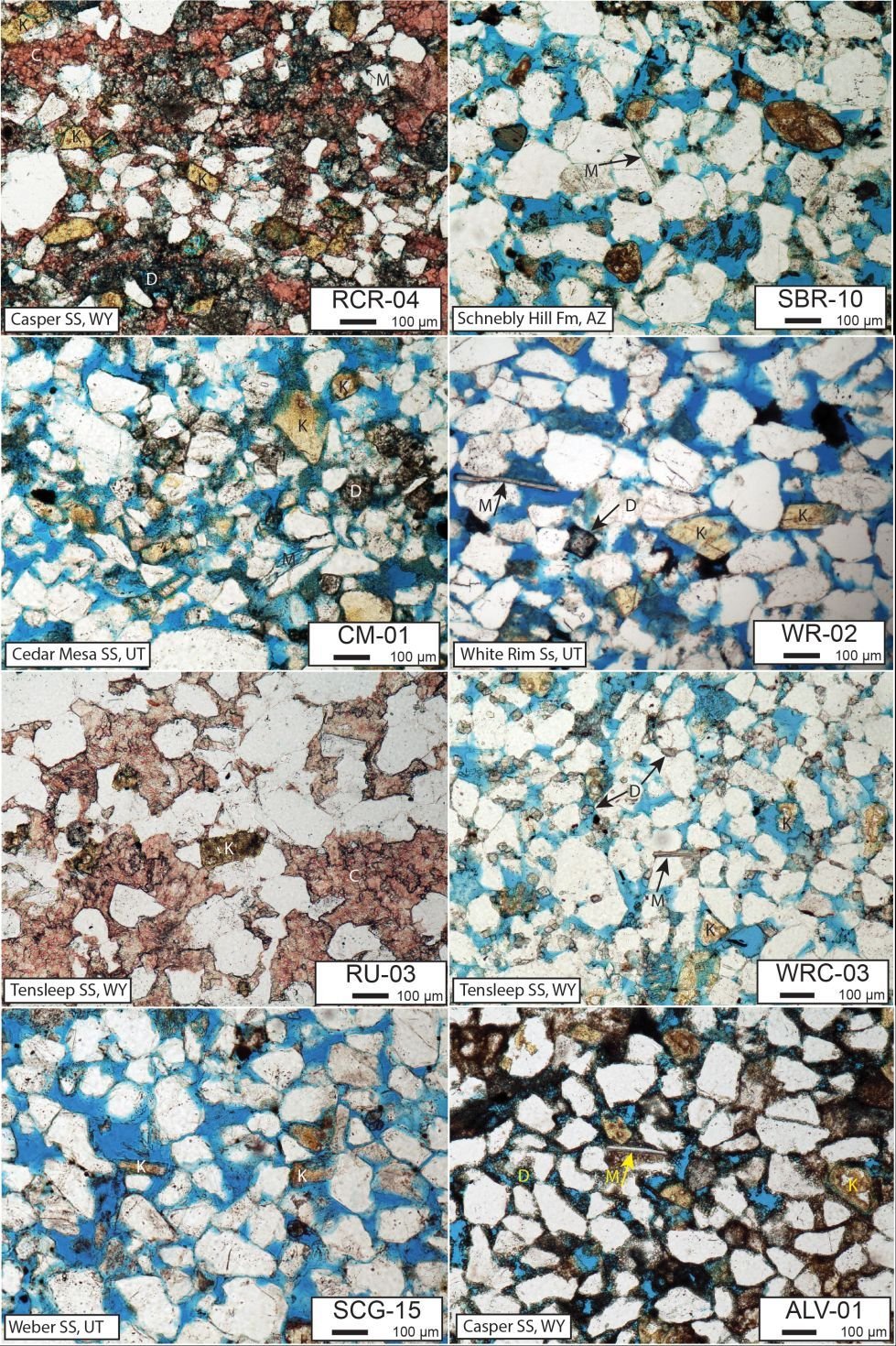
Figure 8. Mica and angular K-feldspar found in thin sections of Pennsylvanian and Permian sandstones of the western United States. C calcite, D dolomite, K K-feldspar, M muscovite. RCR Rogers Canyon Road, SBR Sedona Brins Ridge, CM Cedar Mesa, WR White Rim, RU Rawlins Uplift, WRC Wind River Canyon, SCG Sheep Creek Geological Loop, ALV Alcova.
Dolomite has been found to form in small quantities in specialized desert settings (Arvidson and Mackenzie 1997; Bontognali et al. 2010; Wright 1999), but it is not an analog for much more extensive dolomite ooids, clasts, widespread cement and even dolomite beds that can be found within some of the formations in this report (fig. 9). The formation of dolomite is one of the biggest geological mysteries, because its formation requires fluids (Lippman 1973), high temperatures (>100°C) and/ or high pressures (Arvidson and Mackenzie 1997). Water circulation must be constant and there must be a steady supply of Mg2+ and CO32- ions (Morrow 1988). These conditions must all be met in order for the mineral to form; conditions that are much more likely in a marine setting rather than a sabkha or a desert. Although dolomite has been found in sabkhas (Bontognali et al. 2010), these environments also have specific sedimentary features which seem to be missing in the studied sandstones (Kendall 2010).
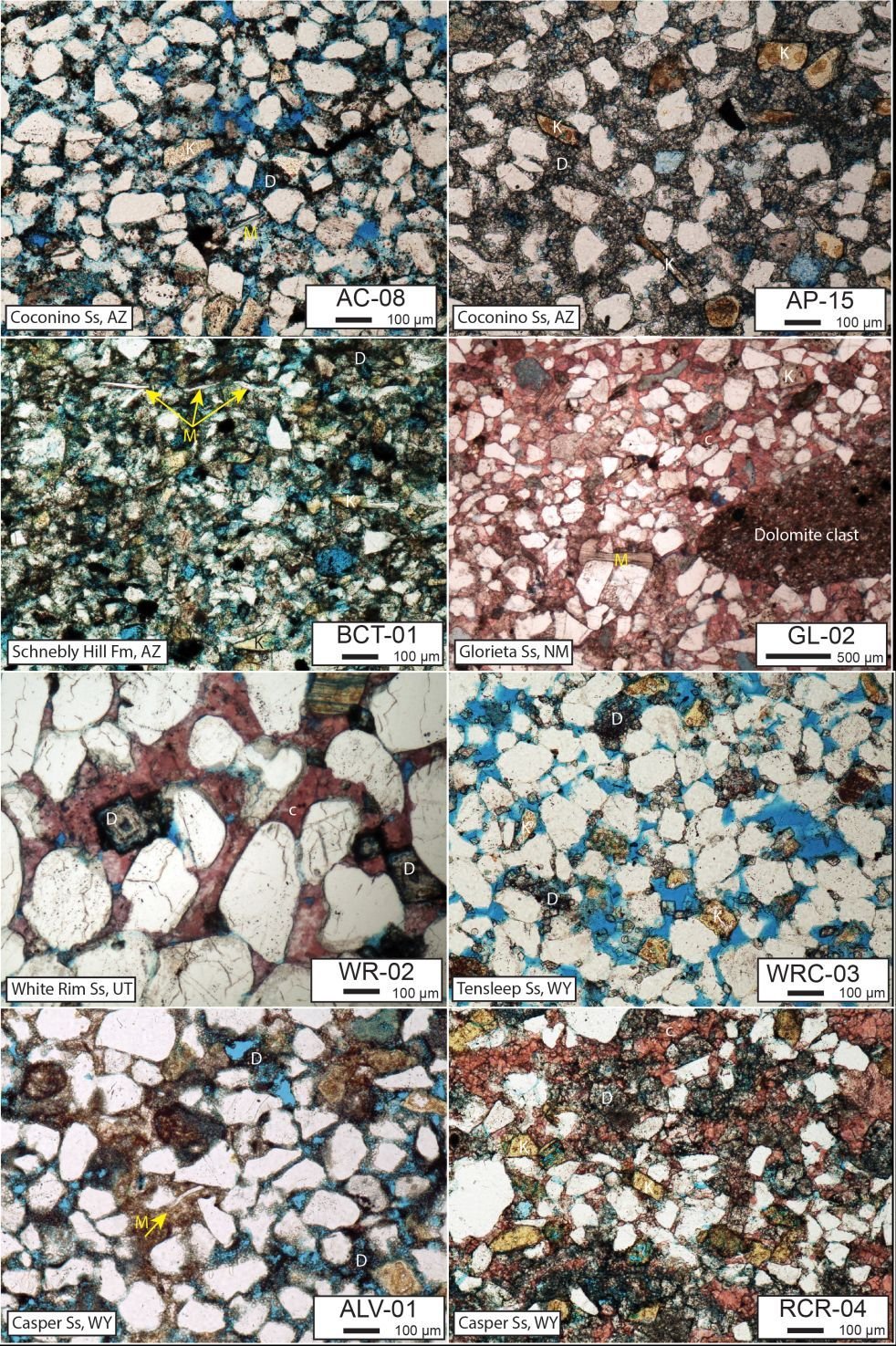
Figure 9. Occurrence of dolomite in thin section for some of the Pennsylvanian and Permian sandstones of the western United States. C calcite, D dolomite, K K-feldspar, M muscovite. AC Andrus Canyon, AP Andrus Point, BCT Bell Canyon Trail, GL Glorieta Sandstone, WR White Rim, WRC Wind River Canyon, ALV Alcova, RCR Rogers Canyon Road.
The following Pennsylvanian and Permian sandstones were found to have considerable amounts of dolomite within them: Broom Creek (Condra, Reed, and Scherer 1940; McCauley 1956), Casper (Agatston 1954), Cassa Group (Condra, Reed, and Scherer 1940; McCauley 1956), Coconino (Whitmore et al. 2014), Diamond Creek (Bissell 1962), Fairbank Formation (McCauley 1956), Fort Apache Member of the Schnebly Hill Formation (Blakey 1984, personal observations), Ingleside Formation (Maughan and Ahlbrandt 1985), Quadrant (James 1992; Saperstone and Ethridge 1984), Scherrer Formation (Luepke 1971), Tensleep (Agatston 1954; James 1992; Mankiewicz and Steidtmann 1979), Toroweap Formation (Rawson and Turner-Peterson 1980), Tubb Sandstone Member of the Clear Fork Group and Abo Formation (Hartig et al. 2011), Weber (Driese 1985), and the Yeso Formation (Baars 1979).
In addition the following Pennsylvanian and Permian sandstones (or the carbonate beds within the sandstones) in this report have fusulinids or other types of marine fossils: Bingham Mine Formation (Tooker and Roberts 1970), Broom Creek (Condra, Reed, and Scherer 1940), Brushy Canyon Formation (Harms 1974), Bursum Formation (Myers 1972), Casper Formation (Hoyt and Chronic 1962), Hudspath Cutoff Formation (Roberts et al. 1965), Scherrer Formation (Luepke 1976), Tensleep (Verville 1957; Verille, Sanderson, and Rea 1970), Toroweap (Rawson and Turner-Peterson 1980), and the Weber Formation (Bissell 1964b).
We have found what we interpret as parabolic recumbent folds in the Coconino and in the Toroweap (Whitmore, Forsythe, and Garner 2015). Rawson and Turner-Peterson (1980, 349) report recumbent folds in the cross-beds of the Toroweap and we think the ones they mention are some of the same ones we have also identified. McKee and Bigarella (1979, 202) picture a fold from Wupatki National Monument that we also believe is a parabolic recumbent fold when we field checked it (Whitmore, Forsythe, and Garner 2015). These specific types of folds have not been reported in the other formations is this paper, as far as we know. The significance of these folds is that they only form penecontemporaneously during the process of subaqueous cross-bed formation. Knight (1929) describes some folds that might be parabolic recumbent folds in the Casper Formation of Wyoming. The present author tried to locate these during the 2011 field season, but the location that Knight described is located on inaccessible private property. Knight described trough cross-bedding in the Casper; it is interesting that trough cross-bedding is also present in the Pennsylvanian Sharon Conglomerate (Formation) of northeast Ohio where these types of folds are dramatically displayed (Wells et al. 1993), and have been field checked by the author. One small fold that resembles a parabolic recumbent fold was found in the Tensleep Sandstone (fig. 10) in Wyoming.
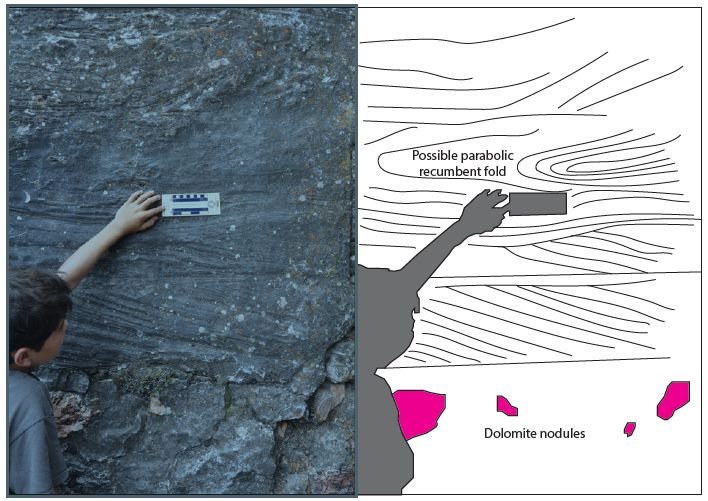
Fig. 10. A possible small parabolic recumbent fold in the Tensleep Sandstone, Tensleep Canyon, Wyoming. What first appeared to be chert nodules, reacted weakly with HCl showing that they were instead dolomite. Thin and laterally extensive dolomite beds were also present in the outcrop.
Brand has done exceptional work in interpreting the tetrapod footprints found in the Coconino (Brand 1979; Brand and Tang 1991). He experimented with salamanders—observing their tracks in dry sand, damp sand, and subaqueous sand. Based on his experimental observations, the subaqueous salamander tracks were the most similar to the Coconino tracks. Marchetti et al. (2019) disagreed with Brand (1979) after completing their own set of laboratory experiments. They interpret the Coconino trackways as indicative of tracks being made in dry sand. However, from personal observations of trackways in the Coconino, the trackways Marchetti et al. made in dry laboratory sand lack the digit details that are in the Coconino and in Brand’s underwater experiments. Francischini et al. (2019) have recently discussed the possibility of some of the Coconino tracks belonging to diadectomorphs, their first known (and unexpected) occurrence in “eolian” environments. Toepelman and Rodeck (1936) describe some tracks from the Lyons Sandstone of Colorado, which is sometimes known as the “twin of the Coconino” (McKee and Bigarella 1979). Mancuso et al. (2016, 374) report similar tracks “essentially belonging to the ichnogenus Chelichnus” from the Weber, Coconino, De Chelly, Lyons, Cedar Mesa, and Casper Formations. They also report occurrences in two Permian cross-bedded sandstones from Argentina and four sandstones from Europe. Studies and observations like Brand’s on the Coconino have not been completed on these other sandstones to see if the trackways have the same characteristics as those in the Coconino; perhaps because the trackways are not as common in these other sandstones. However, based on similar characteristics of these other sandstones with the Coconino, it is predicted some of the same subaqueous interpretations could be reached.
Average degrees of foreset dips in modern eolian settings can be in the mid-20s and even lower. For example, Ahlbrandt and Fryberger (1980) reported average dips from foresets of three dune types in the Nebraska Sandhills (fig. 11 A–C): 22° for barchans (n = 149), 24° for transverse ridge dunes (n = 72) and 16° for blowout dunes (n= 120). However, a significant percentage of the foresets had dips greater than 25° (about 37% for barchans, about 52% for transverse ridge and about 13% for blowout dunes, with many dips in the 30° range. There is a significant difference between the foreset dips of modern dunes, like those in the Nebraska Sand Hills, and those of supposed ancient eolian facies. In supposed ancient eolian facies, individual dips are only rarely greater than 25° (see fig. 6 A–O), while those preserved in Nebraska have frequent dips greater than 25°. This is graphically illustrated in fig. 12 where some ancient cross-bed dips are compared with the Nebraska Sand Hills. Some have claimed that cross-bed dips of supposed eolian sandstones have average angles in the low 20s because of compaction during diagenesis (see discussion on page 280 of Walker and Harms 1972, for example). However, when these sandstones are examined under the microscope, there is little evidence for the severe compaction that is probably needed to reduce the dips from the angle of repose to the low 20s. We have done some preliminary investigation on this problem (Emery, Maithel, and Whitmore 2011), but more work is needed. At this juncture, we believe that if these sandstones were eolian, they would contain a significant number of dips greater than 25°, more in line with the data from the Nebraska Sand Hills.
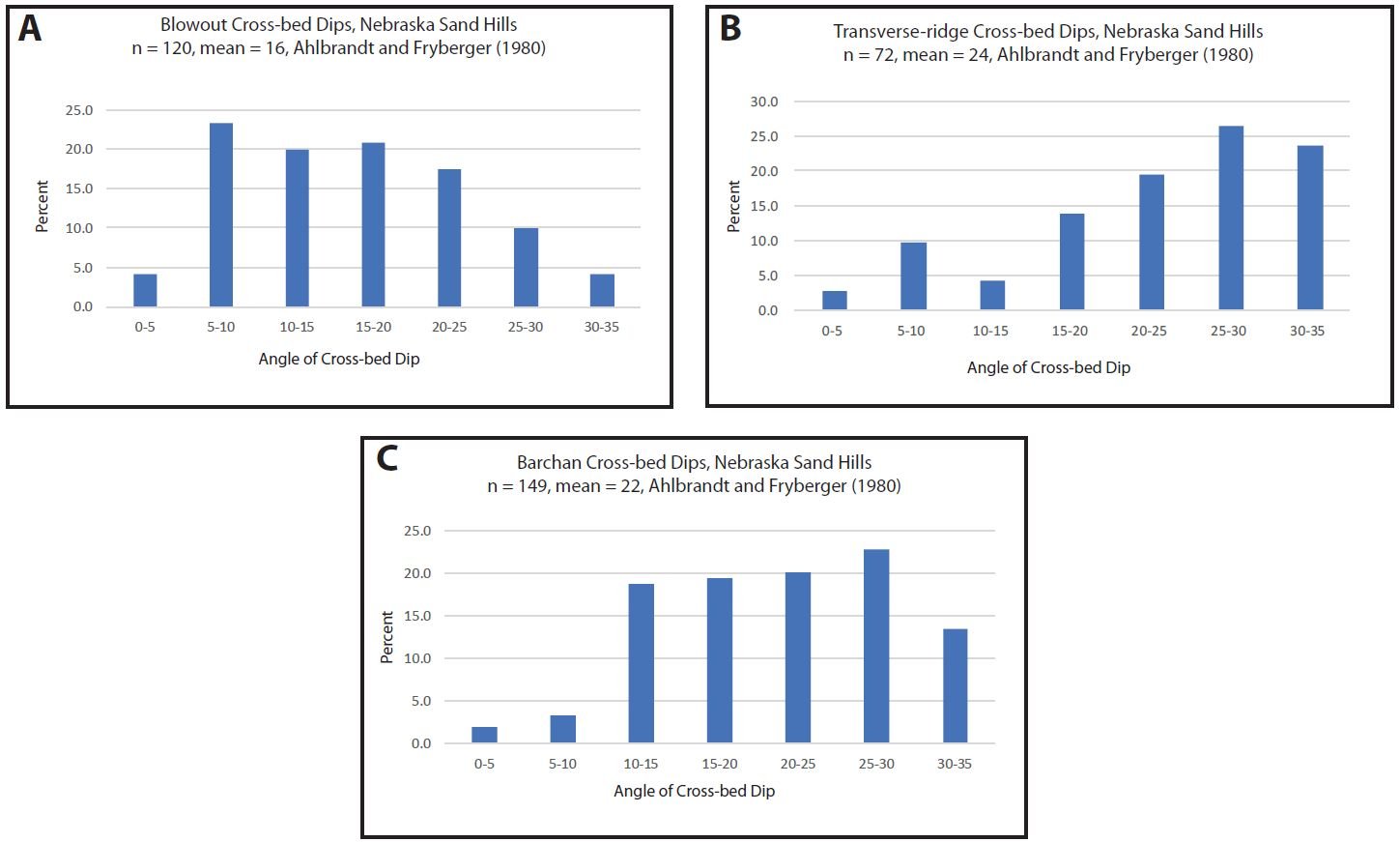
Fig. 11. Graphs of cross-bed dips for the Nebraska Sand Hills (Late Quaternary–Holocene). Data from Ahlbrandt, and Fryberger 1980.
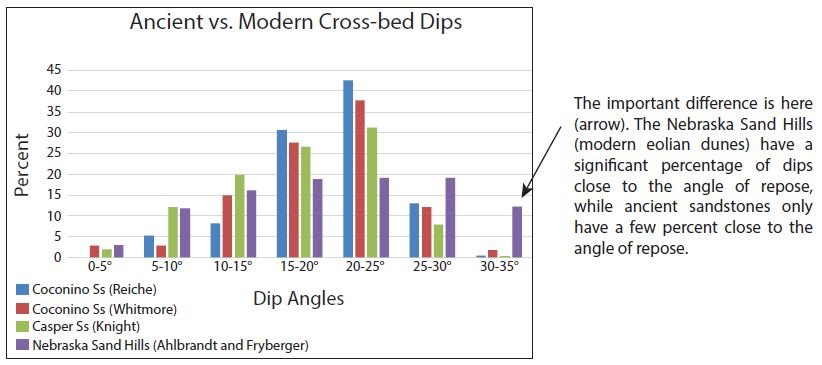
Fig. 12. A comparison of cross-bed dips between all of Reiche’s data from the Coconino Sandstone (1938, n = 193), Whitmore’s data from the Coconino Sandstone (this paper, n = 214), all of Knight’s data from the Casper Sandstone (1929, n = 806) and all of Ahlbrandt and Fryberger’s data from the stabilized relatively modern dunes in the Nebraska Sand Hills (1980, n = 341).
One of the most basic and overlooked observations that one can make when standing on the rim of the Grand Canyon is the significance of the planar contacts between rock layers that can be traced as far as the eye can see. Ariel Roth (2009) calls them “flat gaps.” Between some of the layers, like the Hermit Formation and Coconino Sandstone (fig. 1E), a sharp, flat contact can be found. Both of the formations are supposed to be formed in terrestrial settings: a floodplain for the Hermit and an erg for the Coconino. Yet, the contact between the formations is everywhere flat, even where the contact is transitional in the eastern end of Grand Canyon (Whitmore and Peters 1999). From a conventional view, there must be a significant amount of missing time (5–10 Ma?) because just 125 km south of Grand Canyon, one can find about 300 m of Schnebly Hill Formation sandwiched in between the Hermit and Coconino (Blakey and Knepp 1989). In Holbrook, the Schnebly Hill is even thicker! Time must have passed between the deposition of the Hermit and the Coconino to allow for the thick Schnebly Hill Formation to be deposited, assuming the top of the Hermit is isochronous. Especially in a terrestrial setting one might expect gullies, valleys, and canyons to be cut into the Hermit before the Coconino was deposited. While the example might seem extreme, there is more than 1.5 km of relief in the landscape of the Grand Canyon, perhaps made in six million years or less according to the most recent conventional estimates. In Death Valley, the relief is even greater when you consider the height of the mountain ranges to the west. In just about any terrestrial setting considered, there are often elevation changes of several meters over short distances. Exceptions might be a lake bed, but no one is suggesting that for the Hermit/Coconino contact. And yet, the contact between the two formations is flat. While flat surfaces are uncommon in terrestrial settings, they are quite common on the seafloor. So, it is not surprising that we see flat contacts between marine layers, which are most of the rocks in Grand Canyon, even from a conventional perspective. There is still missing time in between many of these layers from a conventional view, sometimes in excess of 100 Ma, but at least that is easier to explain in a marine setting. Thus, the flat contact between the Hermit and Coconino is best interpreted in a marine setting. This is not only true for the Coconino, but for all of the other supposed “erg” deposits that correlate with the Coconino. They have flat basal contacts as well.
In modern desert settings, like Death Valley or the Sahara, sand dunes are not the most common topographic feature. Actually, sand dunes comprise a very small percentage of the surface area of most deserts (Wilson 1973). Covering the floor of most deserts is bedrock, coarse alluvium (desert pavement), or fine-grained playa deposits with various salts and desiccation features. It is significant that these kinds of features are either missing or poorly defined beneath what are considered to be ancient eolian deposits. Some have suggested that the sand-filled cracks that can be found at the Hermit/Coconino contact are desiccation cracks that have been filled with sand from the Coconino, but the Hermit lacks the appropriate clays for them to be desiccation cracks and the sand-filled cracks have features of sand injectites, not desiccation cracks (Whitmore and Strom 2010).
In summary, the grain sorting, grain rounding, angular K-feldspar, muscovite, primary current lineation, lack of avalanche tongues, shallow cross-bed dips, parabolic recumbent folds, frequent dolomite, general lack of specific eolian sedimentary patterns, fossil footprint characteristics, flat contacts, the presence of marine fossils and facies, and the observation that these formations can be correlated with each other are difficult to explain if the rocks represent a fossil erg, but fit nicely with a marine depositional environment. Some have suggested the cross-bedded sandstones described in this study formed in a coastal setting and correlate directly with associated marine sands (see for example Rawson and Turner-Peterson 1980; Blakey and Ranney 2008), but the diagnostic sedimentary structures (like those typical of beach facies) that would support this hypothesis have yet to be reported.
2. Paleocurrents
A number of paleocurrent studies have been completed on the upper Paleozoic and lower Mesozoic sandstones of the western United States, most with the goal of determining wind directions of the giant ergs believed to have been present during the time they were deposited (Brand, Wang, and Chadwick 2015; Marzolf 1983; Opdyke and Runcorn 1960; Parrish and Peterson 1988; Peterson 1988; Poole 1962; Reiche 1938; Saperstone and Ethridge 1984). Some of these paleocurrent patterns are illustrated in fig. 7; many of these authors have noted a general trend of these current indicators from north to south. Statistics probably should be developed to determine the relative strength of the current patterns in a uniform way; some of the azimuths on fig. 7 indicate multiple dozens of measurements, while others indicate only a few measurements. However, it still appears that there is some kind of trend. Much has been written about the consistent southerly-directed cross-bed dip pattern in many of these formations. But, should cross-bed azimuths be expected to show the same directional pattern for eolian dunes across a wide geographical area like western North America during the Permian? In looking at wind data for the Sahara and all of its many ergs (fig. 13), wind directions and dune azimuths are extremely variable across the area, even when considering the same latitude. The varied directions are caused by various topographic obstacles that shift the wind (Mainguet 1978). Note the large circular gyre in the middle of the area; this means winds are blowing every single direction when the whole area is considered.
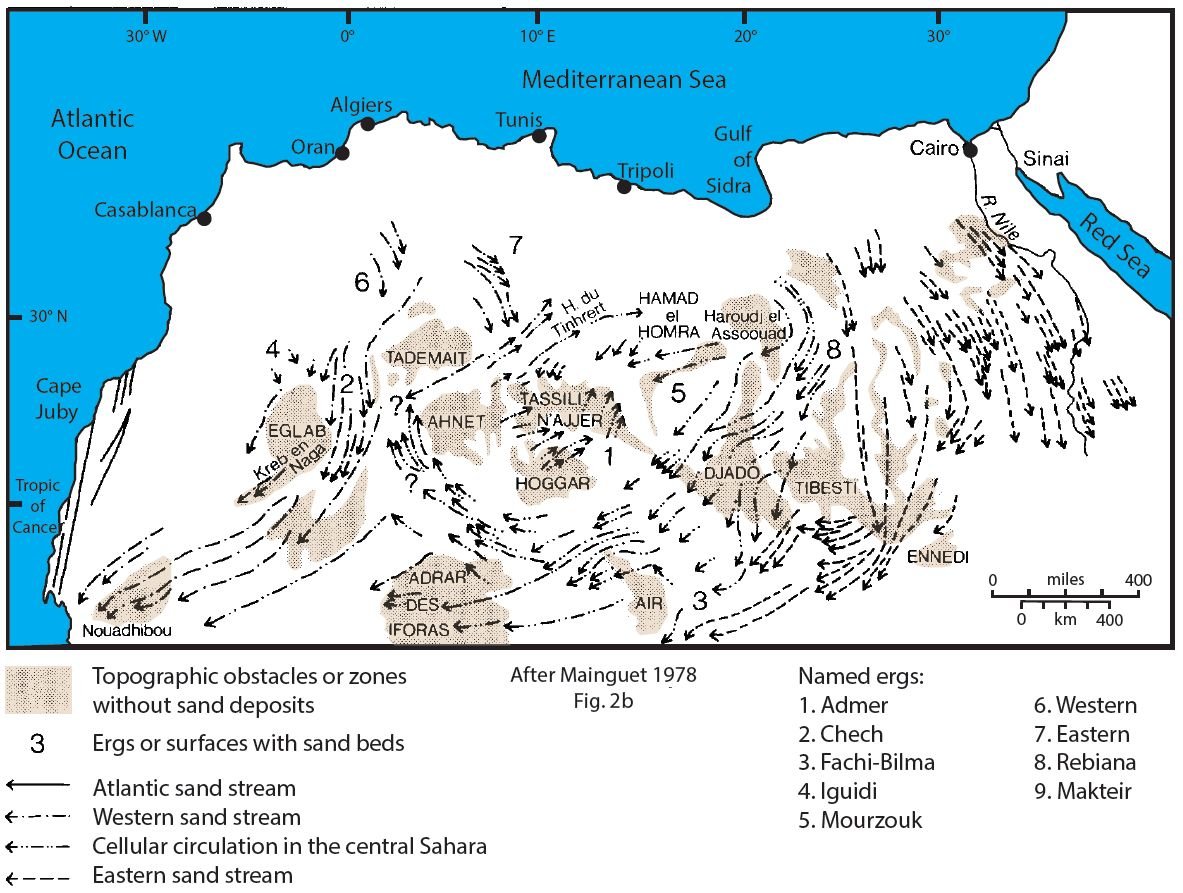
Fig. 13. Generalized map of the Sahara Desert showing the largest ergs (#1–9), sand-less areas, obstacles to wind and general wind directions. Note the large gyre centered in northern Africa producing wind directions of 360°. Map is after fig. 2b of Mainguet (1978).
If one examines the tectonic elements of the western United States during the late Paleozoic (fig. 14) it is difficult to image why all the cross-bed dips trend in the same direction, if the subaerial topography was so variable. Across the area there are multiple uplifts and basins which should have caused shifts in wind patterns. Blakey (2009) and Blakey and Ranney (2008, 2018) have drawn paleogeographic maps that illustrate their interpretation of what western Pangea may have looked like during the Late Paleozoic from a conventional viewpoint. Would not a paleogeography like this have caused multiple prevailing wind directions similar to what is observed in the Sahara today? Most significantly, in the middle of this ancient area is the Uncompahgre Uplift also known as the Ancestral Rocky Mountains, within which all of the units described in this paper are absent. Features like this should cause marked changes in cross-bed azimuths because of wind direction shifts around this feature. Additionally, it becomes problematic how the sand in a giant erg like this could survive during the forthcoming marine transgression. Recall, covering the Permian sands are formations like the Kaibab Limestone, the Phosphoria Formation, the Blaine Formation and other marine deposits, many containing gypsum. In order for a giant erg to be preserved, the whole area needs to undergo slow subsidence, without erosion. Rodriguez-López et al. (2014, 1493) state it like this: “Long-term preservation of aeolian accumulations in the ancient record requires that the body of strata is placed below some regional baseline, beneath which erosion does not occur (Kocurek and Havholm 1993). Thus, the rate of generation of accommodation space and the rate at which aeolian accumulations fill that space is a fundamental control on preserved architectural style (e.g. Howell and Mountney 1997).”
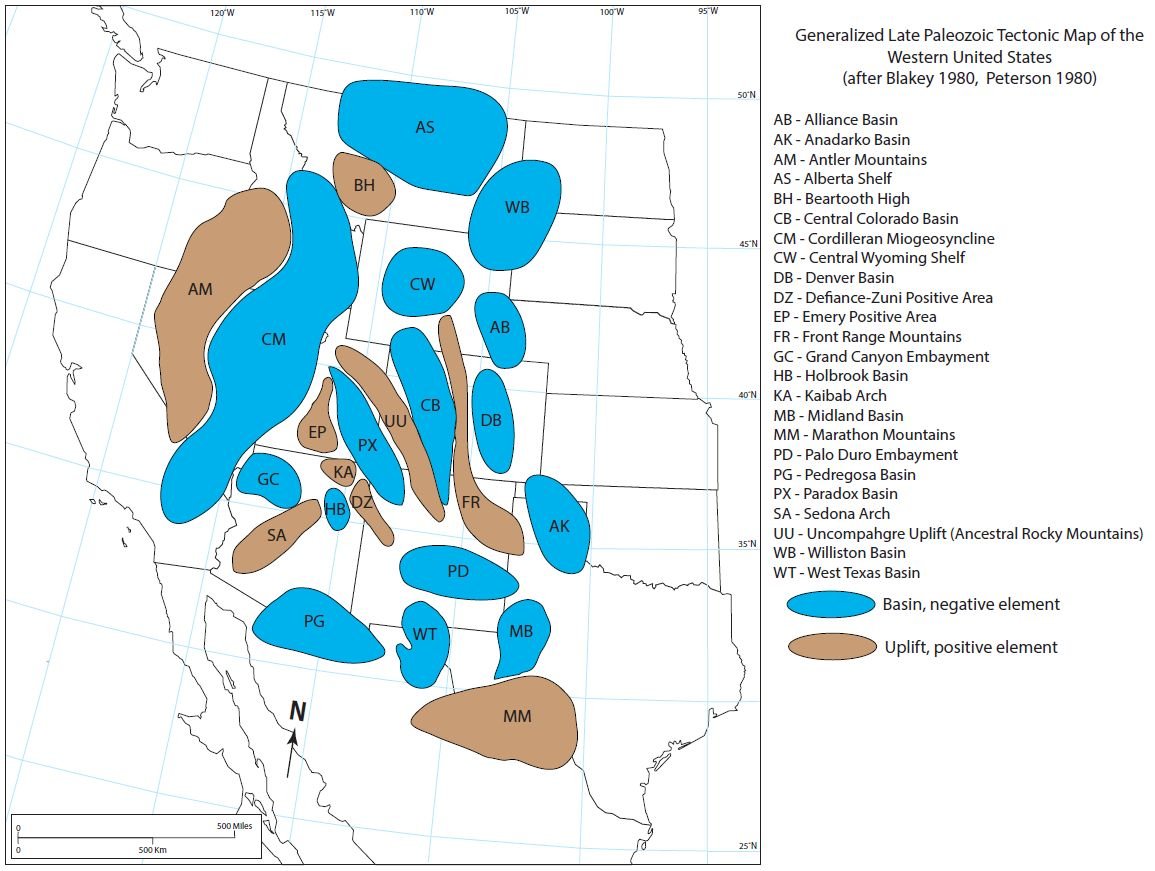
Fig. 14. Generalized Late Paleozoic tectonic map of the western United States. Data obtained from Blakey (1980) and Peterson (1980).
Cross-bed data from the sources listed above are not specific enough to decide between aqueous or an eolian origin. It is predicted that if this could be done, that not much of a difference would be seen between the two sets of data. (Note: Rawson and Turner-Peterson [1980] were trying to accomplish something similar to this, because they compared cross-bed dip angles and azimuths in the Toroweap and Coconino and used the similar measurements to argue that parts of the Toroweap were eolian.) However, from a conventional view, there should be a difference. Cross-bed azimuths in eolian settings should be fairly indicative of wind regime, especially for transverse-type dunes.
However, if this area were primarily underwater, as we might predict with a Flood model, we might expect many of the currents to trend in the same general direction, only occasionally being deflected by obstacles such as the Uncompahgre Uplift. In the analysis of paleocurrent trends for major periods of geological time, it can be discerned from the data of Brand, Wang, and Chadwick (2015) that whole-continent paleocurrent patterns existed in the Paleozoic and Mesozoic, but not so much in the Cenozoic, where the currents are highly variable in the American West (personal communication with A. Chadwick, 2017). During the Flood itself, we might expect paleocurrents to be driven primarily by tectonic forces or strong tidal activity and uplifts, which were probably of greater influence than the wind (Genesis 7:11, 8:1). As the mountainous regions of the continents rose while the Flood was ending, a more variable pattern in paleocurrents should arise—which we see in Brand et al.’s data for the Cenozoic. The currents would be variable because water (this author believes rivers) would flow in all directions away from a topographic high.
Strong ocean currents can produce large sand waves, which can have similar characteristics (size, shape, cross-beds, etc.) as eolian dunes (Barnard et al 2006; Cartwright and Stride 1958; Poppe et al. 2006, 2007, 2011). The Coconino and other associated cross-bedded sandstones may not have been deposited precisely by sand waves, but this would be a working model to consider. Although there are factors that can deflect ocean currents, just like wind currents can be deflected, it might be expected that cross-bedded features produced by ocean currents would be more consistent over a broader area. It is much easier to deflect an air current than a water current because of the amount of mass that needs to be redirected. It would be interesting to collect cross-bed data from the sandstones in this study and compare cross-bed azimuths for those beds that are clearly marine (that have fusulinids, for example) with those that are thought to be eolian. It is predicted that the azimuths would not be significantly different if the cross-beds were made by the same mechanism.
Furthermore, it might be predicted from a conventional paradigm that offshore current features would have different cross-bed azimuths than onshore eolian features. This is because ocean currents are deflected to the right of prevailing wind directions (in the northern hemisphere) due to the Coriolis Effect. Ekman (1909) was able to show net water deflection could be as much as 90° to the right of prevailing winds. Thus, if the conventional paradigm is correct, with rock units having both offshore dune features (to explain abundant fusulinids and dolomite in the sand, for example) and onshore eolian deposits, the offshore features should have consistent cross-bed azimuths to the right of the eolian features and should be separated by distinctive beach facies. To the author’s knowledge, this has yet to be demonstrated in the literature for any of the deposits discussed in this paper. Instead, consistent cross-bed dips for all of the formations suggest a singular, subaqueous origin.
3. Provenance studies
Johansen (1988) suggested a northwestern North American cratonic source for the upper Paleozoic sandstones in western United States. However, more recent provenance studies with detrital zircons have been completed on some of the sandstones in the present study. Link et al. (2014) studied the Wood River Formation (Idaho), the Tensleep Sandstone (Wyoming) and the Weber Sandstone (Utah, Colorado). They concluded that “a continental-scale system transported most of the sand grains in the Wood River and Tensleep Sandstone” (133). This sand arrived from the northeast and likely had its origin from the Grenville Province of eastern North America. There were also significant zircon contributions from the Yavapai-Mazatzal provinces of northwest Colorado, the Copper Basin Group of Idaho and from an area in the western Cordillera, especially for the Weber Sandstone.
Soreghan and Soreghan (2013) examined zircons from the Permian Delaware Basin, and of particular interest for this paper, the Brushy Canyon Formation. The authors state that there has been a long belief that the clastic sediments that fill the Delaware Basin originated from the Ancestral Rocky Mountains. However, their zircon studies show origin from Paleozoic, Neoproterozoic, and Mesoproterozoic (Grenville) sources. They conclude (798) the sediment may have come from the Ouachita system, recycled Appalachian material, and sources now in Mexico and Central America.
Dickinson and Gehrels (2003) and Gehrels et al. (2011) examined zircons from many of the formations in Grand Canyon including the Pennsylvanian and Permian Esplanade Sandstone, Hermit Formation, Coconino Sandstone, Toroweap Formation, and Kaibab Limestone. Zircon-age results showed similar patterns for all of the Permian units in Grand Canyon and the authors concluded that an Appalachian source was most likely for the zircons found in these units.
Lawton, Buller, and Parr (2015) studied zircons from Diamond Creek, Castle Valley, White Rim, and Cutler Group sandstones. They found that the Cutler Group strata were mostly sourced from the Uncompahgre Uplift, a source not more than 40 km away. However, the Diamond Creek, Castle Valley, and White Rim Sandstones all showed a significant proportion of zircons that were likely sourced from Appalachian and eastern Laurentian sources, not unlike the other cross-bedded sandstones in this study. The authors suggest that sand was carried by transcontinental rivers from Appalachia to the western edge of Laurentia where these sandstones accumulated.
The four studies cited above all agree that sand for these western United States Permian sandstones originated from various source rocks in the Appalachian area and then was transported westward by rivers. Some of the sand was sourced locally from the Ancestral Rocky Mountains (Uncompahgre Uplift), but it is surprising that more sand did not originate from there. From the data collected thus far from zircon studies, the Uncompahgre Uplift only contributed sediment to formations that were most near to it (Weber and Cutler). Other formations have primary contributions from the Appalachian area, even though that source is much more distant than the Uncompahgre Uplift. Authors have primarily suggested fluvial and eolian transport of sand from the Appalachians to the western margin of Pangea. Eolian transport seems unreasonable because of the micas and angular feldspars that are found in some of these formations. Micas and angular K-feldspars could survive fluvial, or even marine transport intact; but it seems likely they could not arrive this way from simple eolian transport because of the abrasion that easily happens to these minerals in eolian settings (Borsch et al. 2018; Whitmore and Strom 2017, 2018). Fully marine currents, as indicated by the paleocurrent data (Brand, Wang, and Chadwick 2015), seem to be a more attractive option for transportation of distant sand.
4. The reality of the geological column
Some creationists have denied the reality of the geological column (Oard 2010a, 2010b; Reed and Froede 2003; Woodmorappe 1981). But patterns demonstrated in this paper, as well as in larger projects like COSUNA and Clarey’s intercontinental correlations (Clarey and Werner 2018), clearly demonstrate that some rock layers are “blankets” that cover wide areas of the continents. (Note: The “blankets” being referred to here are high above modern sea levels, and not like the widespread deposits close to modern sea level as in the carbonates surrounding Africa as in fig. 9b of the Tejas sequence of Clarey and Werner 2018.) The Coconino and the formations that have been demonstrated to correlate with it, are one of these “blankets.” This has been recognized for many years. Donald Baars published a paper titled “Permian blanket sandstones of Colorado Plateau” in 1961. The Geologic Atlas of the Rocky Mountain Region (edited by Mallory 1972a) is a resource that shows how not only the Permian units can be traced over the Rocky Mountain region, but many of the other Paleozoic and Mesozoic formations as well (the “blankets” are not so widespread in the Cenozoic). Multiple rock units that can be traced over wide areas are the very essence of the definition of an ordered “geological column” and demonstrate that similar processes were happening over wide geographic areas at some particular points in time. When widespread rock layers can be correlated with one another, it seems as though an “event” is the most reasonable explanation for the origin of the rocks. Rock layers like the Coconino and its equivalents should be viewed as diachronous layers, in the sense that the northern units are mostly Pennsylvanian and the southern units are early Permian in age. In the case of the Coconino, it appears the northern part was deposited first (and is slightly older), followed by the southern part (which is slightly younger) as evidenced by regional cross-bed trends (which have a southerly direction).
5. Significance of these sandstones worldwide
Many of the Permian sandstones that can be found around the world (Appendix 3) have the same characteristics as the Permian sandstones of the western United States including footprints, association with deposits of a chemical nature (dolomite, halite, gypsum), large cross-beds with dips in the low 20s, laminated sands, textural characteristics, mica (Borsch et al. 2018) and angular K-feldspars (Whitmore and Strom 2018). Many times, these formations are directly associated with marine deposits that lead many of the authors to suggest the eolian sandstones were deposited in coastal environments. From the data gathered in Appendix 3, it appears there were four areas of primary sand deposition during the Permian: western United States, maritime Canada-western Europe, Brazil-Argentina and Saudi Arabia (fig. 15).
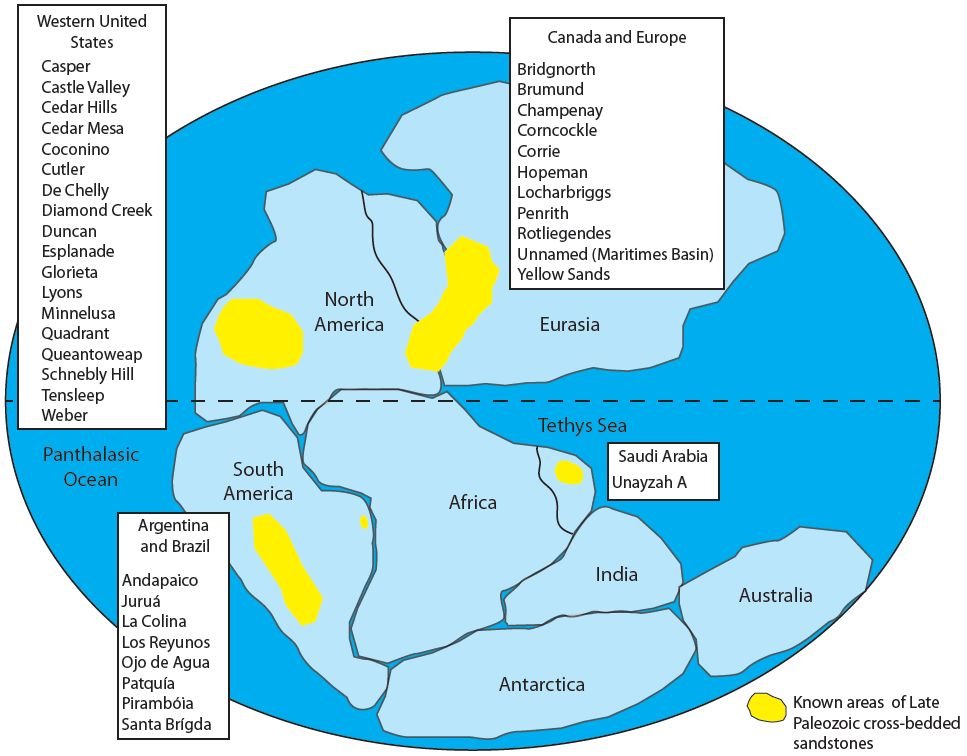
Fig. 15. Locations of cross-bedded sandstones attributed by most to eolian deposition during the Late Paleozoic when Pangea was assembled. References and data for this figure are contained within Appendices 2 and 3. Other areas, yet unknown, may be present in areas like Asia and Antarctica. In a Flood model, Pangea would have assembled about midway through the Flood, and it would have been mostly underwater.
It was observed that many of the sandstones listed in Appendix 3 have similar characteristics as those found in the Coconino (Whitmore and Garner 2018). While working on the Coconino project many of the sandstones described in Appendix 3 from western North America and Europe were visited and sampled. In addition, our study of the literature, outcrop observations and examination of thin sections shows that these formations have many similarities to the Coconino.
While some criteria have been established to recognize ancient eolian sandstones (Hunter 1977; McKee and Bigarella 1979), this study found that most papers ignore the fine details, and instead attribute an eolian origin on the basis of just a few characteristics. The most commonly cited evidence for an eolian origin are “steep” cross-bed dips and large-scale cross-beds. Rarely is any petrographic data presented, but often claims of exceptional sorting, exceptional rounding, and grain frosting are presumably made based on only hand sample observation with a low-power loupe. While precursory observations are necessary in any study, most do not realize they may have missed details such as mica and angular K-feldspar which are clear indicators of subaqueous transport and deposition (Borsch et al. 2018; Whitmore and Strom 2017, 2018). In the large amount of literature that was examined for this present paper, petrographic analysis and the presence of mica or angular K-feldspar was only rarely mentioned. In our limited study outside of the Coconino it appears marine indicators may be fairly common in all of these sandstones.
It has been argued by many that Pangea, during the Permian, was a dry and arid supercontinent (Blakey and Ranney 2018, Chapter 6). The evidence for this rests primarily with the eolian-like sandstones described in this paper and the presence of halite, gypsum, phosphate, and anhydrite that are often associated with these cross-bedded sandstones. These deposits are often interpreted as “evaporites” formed by the evaporation of sea water in large marine basins forming the minerals in an arid environment. The closest modern analogy might be the sabkhas around the edges of the Persian Gulf. It is beyond the scope of this paper to argue for a marine origin for these minerals, but it is interesting that many of these minerals, along with dolomite, are often associated with the Permian cross-bedded sandstones. Hovland et al. (2006) and Hovland, Rueslåtten, and Johnsen (2014) have argued that some of these kinds of deposits, especially halite, could be explained by the presence of supercritical water rising from volcanic vents on the seafloor.
Although clear stratigraphic connections cannot be made between the four areas highlighted on fig. 15, the upper Paleozoic sandstones clearly fit Ager’s definition of persistence of facies. They have many common features including bedding style, petrographic details, paleontology (or lack thereof), color and associated rock units of chemical origin. It is believed that there is no other interval in the geological column where all of these features come together. It is similar to the Cretaceous chalks, the Carboniferous coals, the Mississippian limestones, the basal Cambrian sandstones, etc. A feature that may tie the four widely separated areas together are paleocurrents. At least for the western sandstones in the United States the paleocurrents are fairly uniform. As was demonstrated from the Sahara (fig. 13) wind currents are highly variable, even across the same latitude because of various topographic barriers that the air needs to move around. However, with water currents, a more uniform direction might be expected and this could potentially be the one thing that unites these sandstones together and would be inexplicable with an eolian paradigm. Modern deposits that are similar to what the Coconino “blanket” may represent (but probably not exactly analogous) are sand waves. Sand waves contain large cross-beds and form on continental shelves in areas where there are strong currents. The data is sparse for the types of sediment and the cross-bed angles within sand waves (Garner and Whitmore 2011), but there is reason to suspect that modern sand waves have similar cross-bed dips to those found in the Permian sandstones.
Conclusions
The Coconino Sandstone of Arizona is one of the most well-known cross-bedded sandstones and historically has formed the basis for comparison for all other ancient “eolian” deposits. Edwin McKee was the first to publish an in-depth study of the Coconino (1934) and near the end of his career used it as an example to establish criteria for the identification of an eolian sandstone (McKee and Bigarella 1979). The present author has completed further study on the Coconino (summarized in Whitmore and Garner 2018) which included extensive microscopic study, techniques that were not refined when McKee studied the sandstone in 1934 and were not relied upon in his report with Bigarella of 1979. Based on thin section study, outcrop observations and other data, the author believes that the marine origin for the Coconino can now be firmly established and is the best explanation for this iconic sandstone.
During our study of the Coconino, similar sandstones were visited in the field and sampled, both in the United States and Europe as a basis for comparison to the Coconino. In this paper it has been shown that the Coconino can be correlated as a single lithostratigraphic package across the western United States. Thin sections, outcrop observations, the literature and now these correlations—all suggest that the Coconino is a “blanket” sandstone that was deposited in a marine setting that reached from California to North Dakota and from Texas to Idaho. Details that suggest a marine origin for the Coconino were also found in many of these other sandstones, although these other sandstones were not studied in such great detail. It was found that paleocurrent directions are fairly uniform throughout the western U.S. in the Permian sandstones, an observation that would be difficult to explain from the conventional eolian perspective, but would make much more sense if the deposits were marine. The establishment of this “blanket” sandstone along with other blanket layers of the western United States refute those who would suggest the geological column is imaginary.
A survey was completed not only of Permian sandstones in the United States, but for those around the world. Microscopic thin sections of European sandstones showed many of the same characteristics that we found in sandstones of the western United States: mica, angular K-feldspars, dolomite, angular sand grains, poor sorting, and other characteristics not usually found in modern eolian sands. Additionally, details like paleontology, associated deposits, cross-bed angles (much less than the angle of repose) and other features show these deposits are similar to the Coconino and probably had a similar origin. It is argued that Permian sandstones are exceptional examples of what Ager (1981) called the phenomenon of the persistence of facies.
Modern marine sand waves occur in multiple areas on continental shelves where there are strong currents. They are the same scale as modern desert dunes and also contain cross-beds and migrate with a current as do eolian dunes. Sand waves may not be an exact analogue of how we believe the “blanket” of the Coconino was deposited, but we envisage something similar depositing shallow marine sands all over the Pangean continent as it was underwater during the Flood.
Acknowledgments
Ray Strom, Paul Garner, and others have been significant contributors to the Coconino project that we started so many years ago. This paper is just a small part of the larger project. Countless hours have been spent in the field, the lab, and in the office— of which Ray and Paul have been an integral part. Cedarville University provided logistical support and funding to complete this particular paper. Institute for Creation Research and Calgary Rock and Materials Services Inc. contributed significantly, especially in funding the field work and thin section production that has been such an important part of the Coconino project as a whole. The helpful comments and rewording suggestions from the reviewers were much appreciated.
References
Adkison, W. L. ed. 1966. Stratigraphic Cross Section of Paleozoic Rocks Colorado to New York. Tulsa, Oklahoma: The American Association of Petroleum Geologists.
Adler, Frank J. 1986. Correlation of Stratigraphic Units in North America—Mid-Continent Region [MC] Correlation Chart. Tulsa, Oklahoma: American Association of Petroleum Geologists.
Agatston, R. S. 1952. “Tensleep Formation Of The Big Horn Basin.” Wyoming Geological Association Guide Book, 44– 48. 7th Annual Field Conference, Southern Big Horn Basin, Wyoming.
Agatston, Robert S. 1954. “Pennsylvanian And Lower Permian Of Northern And Eastern Wyoming.” Bulletin of the American Association of Petroleum Geologists 38, no. 4 (April): 508–583.
Ager, Derek V. 1981. The Nature of the Statigraphical Record. 2nd ed. London, United Kingdom: MacMillan Press.
Ahlbrandt, Thomas S., and Steven G. Fryberger. 1980. “Eolian Deposits In The Nebraska Sand Hills.” U.S. Geological Survey Professional Paper 1120A: 1–24.
Anderson, Calvin J., Alexander Struble, and John H. Whitmore. 2017. “Abrasion Resistance Of Muscovite In Aeolian And Subaqueous Transport Experiments.” Aeolian Research 24 (February): 33–37.
Andrews, Sarah A., and Lindi S. Higgins. 1984. “Influence Of Depositional Facies On Hydrocarbon Production In The Tensleep Sandstone, Big Horn Basin, Wyoming: A Working Hypothesis.” In The Permian and Pennsylvanian Geology of Wyoming. Edited by J. Goolsby and D. Morton, 183–197. Casper, Wyoming: Wyoming Geological Association, 35th Annual Field Conference Guidebook.
Arthurton, R. S., I. C. Burgess, and D. W. Holliday. 1978. “Permian and Triassic.” In The Geology of the Lake District. Edited by Frank Moseley, 189–206. Yorkshire Geological Society, Occasional Publication No. 3.
Arvidson, Rolf S., and Fred T. Mackenzie. 1997. “Tentative Kinetic Model For Dolomite Precipitation Rate And Its Application To Dolomite Distribution.” Aquatic Geochemistry 2, no. 3: 273–298.
Baars, D. L. 1961. “Permian Blanket Sandstones Of Colorado Plateau.” In Geometry of Sandstone Bodies. Edited by James A. Peterson and John C. Osmond, 179–207. Tulsa, Oklahoma: American Association of Petroleum Geologists.
Baars, D. L. 1962. “Permian System Of Colorado Plateau.” Bulletin of the American Association of Petroleum Geologists 46 (February): 149–218.
Baars, Donald L. 1974. “Permian Rocks Of North-Central New Mexico.” In Ghost Ranch Central Northern New Mexico. Edited by C. T. Siemers, 167–169. New Mexico Geological Society Guidebook, 25th Field Conference.
Baars, D. L. 1979. “The Permian System.” In Permianland. Edited by D. L. Baars, 1–6. Guidebook of the Four Corners Geological Society, 9th Field Conference.
Baars, D. L. 2010. “Geology Of Canyonlands National Park, Utah.” In Geology of Utah’s Parks and Monuments. 3rd edition. Edited by Douglas A. Sprinkel, Thomas C. Chidsey Jr., and Paul B. Anderson, 61–83. Salt Lake City, Utah: Utah Geological Association and Bryce Canyon Natural History Association, Utah Geological Association Publication 28.
Baars, D. L., and W. R. Seager. 1970. “Stratigraphic Control Of Petroleum In White Rim Sandstone (Permian) In And Near Canyonlands National Park, Utah.” The American Association of Petroleum Geologists Bulletin 54, no. 5 (May): 709–718.
Baetcke, Gustav Berndt. 1969. “Stratigraphy Of The Star Range And Reconnaissance Study Of Three Selected Mines.” Ph.D. dissertation, University of Utah.
Baker, Arthur A. 1946. “Geology Of The Green River Desert-Cataract Canyon Region, Emery, Wayne, And Garfield Counties, Utah.” Bulletin of the U.S. Geological Survey 951: 1–122.
Baker, A. A., J. W. Huddle, and D. M. Kinney. 1949. “Paleozoic Geology Of North And West Sides Of Uinta Basin, Utah.” Bulletin of the American Association of Petroleum Geologists 33, no. 7 (July): 1161–1197.
Ballard, William W., John P. Bluemle, and Lee C. Gerhard. 1983. Correlation of Stratigraphic Units in North America (COSUNA) Project—Northern Rockies/Williston Basin Region Correlation Chart. Tulsa, Oklahoma: American Association of Petroleum Geologists.
Baltz, Elmer H. 1965. “Stratigraphy And History Of Raton Basin And Notes On San Luis Basin, Colorado-New Mexico.” Bulletin of the American Association of Petroleum Geologists 49, no. 11 (November): 2041–2075.
Baltz, Rachel May. 1982. “Geology Of The Arica Mountains.” Master’s Thesis, San Diego State University.
Barnard, Patrick L., Daniel M. Hanes, David M. Rubin, and Rikk G. Kvitek. 2006. “Giant Sand Waves At The Mouth Of San Francisco Bay.” EOS 87, no. 29 (18 July): 285–289.
Beard, L. S., R. E. Anderson, D. L. Block, R. G. Bohannon, R. J. Brady, S. B. Castor, E. M. Duebendorfer et al. 2007. “Preliminary Geologic Map Of The Lake Mead 30’ × 60’ Quadrangle, Clark County, Nevada And Mohave County, Arizona.” U.S. Geological Survey. Open File Report 2007-1010.
Bergstrom, D. J., and G. B. Morey. 1984. Correlation Of Stratigraphic Units In North America. Northern Mid-Continent Region Correlation Chart. Tulsa, Oklahoma: American Association of Petroleum Geologists.
Billingsley, George H., and Jeremiah B. Workman. 2000. “Geologic Map Of The Littlefield 30’ × 60’ Quadrangle, Mohave County, Northwestern Arizona.” U.S. Geological Survey Geological Investigation Series, Map I-2628.
Bissell, Harold J. ed. 1959. Geology of the Southern Oquirrh Mountains and Fivemile Pass—Northern Boulter Mountains Area, Tooele and Utah Counties, Utah. Salt Lake City, Utah: Utah Geological Society, Guidebook to the Geology of Utah Number 14.
Bissell, Harold J. 1962. “Permian Rocks Of Parts Of Nevada, Utah And Idaho.” Geological Society of America Bulletin 73, no. 9 (September 1): 1083–1110.
Bissell, H. J. 1964a. “Ely, Arcturus, And Park City Groups (Pennsylvanian-Permian) In Eastern Nevada And Western Utah.” Bulletin of the American Association of Petroleum Geologists 48, no. 5 (May): 565–636.
Bissell, H. J. 1964b. “Lithology And Petrography Of The Weber Formation, In Utah And Colorado.” In Guidebook to the Geology and Mineral Resources of the Uinta Basin, Utah’s Hydrocarbon Storehouse. Edited by Edward F. Sabatka, 67–91. Salt Lake City, Utah: Intermountain Association of Petroleum Geologists, 13th Annual Field Conference.
Bissell, Harold J., and Orlo E. Childs. 1958. “The Weber Formation Of Utah And Colorado.” In Symposium on Pennsylvanian Rocks of Colorado and Adjacent Areas. Edited by B. F. Curtis and H. L. Warner, 26–30. Denver, Colorado: Rocky Mountain Association of Geologists.
Blakey, Ronald C. 1980. “Pennsylvanian And Early Permian Paleogeography, Southern Colorado Plateau And Vicinity.” In Paleozoic Paleogeography of the West-Central United States. Edited by T. D. Fouch and E. R. Magathan, 239–257. Denver, Colorado: The Rocky Mountain Section of the Society of Economic Paleontologists and Mineralogists, Rocky Mountain Paleogeography Symposium 1.
Blakey, Ronald C. 1984. “Marine Sand-Wave Complex In The Permian Of Central Arizona.” Journal of Sedimentary Petrology 54, no. 1 (March 1): 29–51.
Blakey, Ronald C. 1988. “Basin Tectonics And Erg Response.” Sedimentary Geology 56, nos. 1–4 (April): 127–151.
Blakey, Ronald C. 1990. “Stratigraphy And Geologic History Of Pennsylvanian And Permian Rocks, Mogollon Rim Region, Central Arizona And Vicinity.” Geological Society of America Bulletin 102, no. 9 (September 1): 1189–1217.
Blakey, Ronald C. 2003. “Supai Group And Hermit Formation.” In Grand Canyon Geology. 2nd ed. Edited by Stanley S. Beus, and Michael Morales, 136–162. New York: Oxford University Press.
Blakey, Ronald C. 2009. “Paleogeography And Geologic History Of The Western Ancestral Rocky Mountains, Pennsylvanian-Permian, Southern Rocky Mountains And Colorado Plateau.” In The Paradox Basin Revisited: New Developments in Petroleum Systems and Basin Analysis. Edited by W. S. Houston, P. Moreland, and L. Wray, 222– 264. Denver, Colorado: Rocky Mountain Association of Geologists. 2009 Special Publication.
Blakey, Ronald C., and Larry T. Middleton. 1983. “Permian Shoreline Eolian Complex In Central Arizona: Dune Changes In Response To Cyclic Sea-Level Changes.” In Eolian Sediments and Processes. Edited by M. E. Brookfield and T. S. Ahlbrandt, 551–581. Amsterdam, Netherlands: Elsevier. Developments in Sedimentology 38.
Blakey, Ronald C., and Rex Knepp. 1989. “Pennsylvanian And Permian Geology Of Arizona.” In Geologic Evolution of Arizona. Edited by J. P. Jenney, and S. J. Reynolds, 313– 347. Tucson, Arizona: Arizona. Arizona Geological Society. Arizona Geological Society Digest 17.
Blakey, Ron, and Wayne Ranney. 2008. Ancient Landscapes of the Colorado Plateau. Grand Canyon, Arizona: Grand Canyon Association.
Blakey, Ronald C., and Wayne D. Ranney. 2018. Ancient Landscapes of Western North America: A Geologic History with Paleogeographic Maps. Springer Nature.
Blakey, Ronald C., Fred Peterson, and Gary Kocurek. 1988. “Synthesis Of Late Paleozoic And Mesozoic Eolian Deposits Of The Western Interior Of The United States.” Sedimentary Geology 56, nos. 1–4 (April): 3–125.
Bolyard, Dudley W. 1959. “Pennsylvanian And Permian Stratigraphy In Sangre De Cristo Mountains Between La Veta Pass And Westcliffe, Colorado.” Bulletin of the American Association of Petroleum Geologists 43, no. 8 (August): 1896–1939.
Bontognali, Tomaso R. R., Crisógono Vasconcelos, Rolf J. Warthmann, Stefano M. Bernasconi, Christophe Dupraz, Christian J. Strohmenger, and Judith A. McKenzie. 2010. “Dolomite Formation Within Microbial Mats In The Coastal Sabkha Of Abu Dhabi (United Arab Emirates).” Sedimentology 57, no. 3 (April): 824–844.
Borsch, K., John H. Whitmore, Raymond Strom, and George Hartree. 2018. “The Significance Of Micas In Ancient Cross-Bedded Sandstones.” In Proceedings of the Eighth International Conference on Creationism. Edited by J. H. Whitmore, 306–326. Pittsburgh, Pennsylvania: Creation Science Fellowship.
Brand, Leonard, Mingmin Wang, and Arthur Chadwick. 2015. “Global Database Of Paleocurrent Trends Through The Phanerozoic And Precambrian.” Scientific Data 2: 150025. DOI https://doi.org/10.1038/sdata.2015.25.
Brand, Leonard. 1979. “Field And Laboratory Studies On The Coconino Sandstone (Permian) Vertebrate Footprints And Their Paleoecological Implications.” Palaeogeography, Palaeoclimatology, Palaeoecology 28: 25–38.
Brand, Leonard, and Thu Tang. 1991. “Fossil Vertebrate Footprints In The Coconino Sandstone (Permian) Of Northern Arizona: Evidence For Underwater Origin.” Geology 19, no. 12 (December): 1201–1204.
Brill, Kenneth G. Jr. 1952. “Stratigraphy In The Permo-Pennsylvanian Zeugogeosyncline Of Colorado And Northern New Mexico.” Bulletin of the Geological Society of America 63, no. 8 (August 1): 809–880.
Brookfield, M. E. 1977. “The Origin Of Bounding Surfaces In Ancient Aeolian Sandstones.” Sedimentology 24, no. 3 (June): 303–332.
Brookfield, M. E. 1978. “Revision Of The Stratigraphy Of Permian And Supposed Permian Rocks Of Southern Scotland.” Geologische Rundschau 67, no. 1 (February): 110–149.
Butler, W. C. 1971. “Permian Sedimentary Environments In Southeastern Arizona.” Arizona Geological Society Digest 9: 71–94.
Calder, J. H., D. Baird, and E. B. Urdang. 2004. “On The Discovery Of Tetrapod Trackways From Permo-Carboniferous Redbeds Of Prince Edward Island And Their Biostratigraphic Significance.” Atlantic Geology 40, nos. 2–3 (October 10): 217–226.
Cartwright, D., and A. H. Stride. 1958. “Large Sand Waves Near The Edge Of The Continental Shelf.” Nature 181, no. 4601 (4 January): 41.
Caselli, Alberto Tomás, and Carlos Oscar Limarino. 2002. “Sedimentología Y Evolución Paleoambiental De La Formación Patquía (Pérmico) En El Extremo Sur De La Sierra De Maz Y Cerro Bola, Provincia De La Rioja, Argentina.” [“Sedimentology And Paleoenvironmental Evolution Of The Permian Patquia Formation At The Southern End Of Sierra de Maz and Cerro Bola, La Rioja Province, Argentina”]. Revista de la Asociacion Geologica Argentina 57, no. 4 (December): 415–436.
Castor, S. B., J. E. Faulds, S. M. Rowland, and C. M. dePolo. 2000. Geologic map of the Frenchman Mountain Quadrangle, Clark County, Nevada. Nevada Bureau of Mines and Geology, Map 127.
Chan, Marjorie A. 1989. “Erg Margin Of The Permian White Rim Sandstone, SE Utah.” Sedimentology 36, no. 2 (April): 235–251.
Childs, O. E., R. A. Knepp, S. J. Reynolds, G. Haxel, S. Thompson, III, and J. Wright. 1988. “Correlation Of Stratigraphic Units Of North America (COSUNA) Documentation Records For Southern Arizona And Vicinity.” Arizona Geological Survey Open-File Report 88-3.
Chure, Daniel J., George F. Engelmann, Thomas Roger Good, Geoffrey Haymes, and Robin Hansen. 2014. “The First Record Of Vertebrate Tracks From The Eolian Weber Sandstone (Pennsylvanian-Permian), Northeastern Utah: A Preliminary Report.” In Fossil Footprints of Western North America. New Mexico Museum of Natural History Bulletin 62: 95–102. Edited by Martin G. Lockley, and Spencer G. Lucas.
Clarey, Timothy L., and Davis J. Werner. 2018. “Global Stratigraphy And The Fossil Record Validate A Flood Origin For The Geologic Column.” In Proceedings of the Eighth International Conference on Creationism, 327–350. Edited by J. H. Whitmore. Pittsburgh, Pennsylvania: Creation Science Fellowship.
Clemmensen, Lars B., and Jan Hegner. 1991. “Eolian Sequence And Erg Dynamics: The Permian Corrie Sandstone, Scotland.” Journal of Sedimentary Petrology 61, no. 5 (September 1): 768–774.
Clemmensen, Lars B., and Kjell Abrahamsen. 1983. “Aeolian Stratification And Facies Association In Desert Sediments, Arran Basin, (Permian), Scotland.” Sedimentology 30, no. 3 (June): 311–339.
Condon, Steven M. 1997. “Geology Of The Pennsylvanian And Permian Cutler Group And Permian Kaibab Limestone In The Paradox Basin, Southeastern Utah And Southwestern Colorado.” U.S. Geological Survey Bulletin 2000: P1–P46.
Condra, G. E., E. C. Reed, and O. J. Scherer. 1940. Correlation of the Formations of the Laramie Range, Hartville Uplift, Black Hills, and western Nebraska. Lincoln, Nebraska: University of Nebraska Conservation and Survey Division.
Correa, Gustavo A., Maria L. Carrevedo, and Pedro R. Gutiérrez. 2012. “Paleoambiente Y Paleontología De La Formación Andapaico (Paleozoico Superior, Precordillera Central, Argentina).” [“Paleoenvironment And Paleontology Of The Andapaico Formation (Upper Paleozoic, Central Precordillera, Argentina)”]. Andean Geology 39, no. 1 (January): 22–52.
Cramer, Howard Ross. 1971. “Permian Rocks From The Sublett Range, Southern Idaho.” The American Association of Petroleum Geologists Bulletin 55, no. 10 (October): 1787–1801.
Curry, William H. III 1984. “Paleotopography At The Top Of The Tensleep Formation, Bighorn Basin, Wyoming.” In The Permian and Pennsylvanian Geology of Wyoming. Wyoming Geological Association 35th Annual Field Conference Guidebook. Edited by J. Goolsby and D. Morton, 199–211. Casper, Wyoming: Wyoming Geological Association.
Darton, N. H., 1904. “Comparison Of The Stratigraphy Of The Black Hills, Bighorn Mountains And Rocky Mountain Front Range.” Geological Society of America Bulletin 15, no. 1 (January 1): 394–401.
Delorenzo, Kayo, Nardi Dias, and Claiton M. S. Scherer. 2008. “Cross-Bedding Set Thickness And Stratigraphic Architecture Of Aeolian Systems: An Example From The Upper Permian Pirambóia Formation (Paraná Basin), Southern Brazil.” Journal of South American Earth Sciences 25, no. 3 (May): 405–415.
Dickinson, William R., and George E. Gehrels. 2003. “U-Pb Ages Of Detrital Zircons From Permian And Jurassic Eolian Sandstones Of The Colorado Plateau, USA: Paleogeographic Implications.” Sedimentary Geology 163, nos. 1–2 (15 December): 29–66.
Dinterman, P. A. 2001. “Regional Analysis Of The Depositional Environments Of The Yeso And Glorieta Formations (Leonardian), New Mexico.” Master’s Thesis, New Mexico State University.
Doe, T. W., and R. H. Dott Jr. 1980. “Genetic Significance Of Deformed Cross Bedding—With Examples From The Navajo And Weber Sandstones Of Utah.” Journal of Sedimentary Petrology 50, no. 3 (September 1): 793–812.
Doelling, Hellmut H., Robert E. Blackett, Alden H. Hamblin, J. Douglas Powell, and Gayle L. Pollock. 2003. “Geology Of Grand Staircase-Escalante National Monument, Utah.” In Geology of Utah’s Parks and Monuments. Utah Geological Association Publication 28. 2nd ed. Edited by D. A. Sprinkel, T. C. Chidsey Jr., and P. B. Anderson, 189–231. Salt Lake City, Utah: Utah Geological Association and Bryce Canyon Natural History Association.
Donnell, John R. 1958. “The Weber Sandstone In The White River Uplift.” In Symposium on Pennsylvanian Rocks of Colorado and Adjacent Areas. Edited by B. F. Curtis and H. L. Warner, 95–98. Denver, Colorado: Rocky Mountain Association of Geologists.
Driese, Steven G. 1985. “Interdune Pond Carbonates, Weber Sandstone (Pennsylvanian-Permian), Northern Utah And Colorado.” Journal of Sedimentary Petrology 55, no. 2 (March 1): 187–195.
Dunbar, Carl O. Arthur A. Baker, G. Arthur Cooper, Philip B. King, Edwin D. McKee, Arthur K. Miller, Raymond C. Moore et al. 1960. “Correlation Of The Permian Formations Of North America.” Bulletin of the American Geological Society of America 71, no. 12 (December): 1763–1806.
Duncan, Henry. 1830. “An Account Of The Tracks And Footmarks Of Animals Found Impressed On Sandstone In The Quarry Of Corncockle Muir, In Dumfriesshire.” Transactions of the Royal Society, Edinburgh 11: 194–209.
Ekman, Vagn Walfrid. 1909. “On The Influence Of The Earth’s Rotation On Ocean Currents.” Arkiv För Matematik, Astronomi Och Fysik 2, no. 11: 1–51.
Elias, Andreia R. D., L. F. De Ros, Ana M. P. Mizusaki, and Sylvia M. C. Anjos. 2004. “Diagenetic Patterns In Eolian/ Coastal Sabkha Reservoirs Of The Solimoñes Basin, Northern Brazil.” Sedimentary Geology 169, nos. 3–4 (15 July): 191–217.
Emery, Matthew K., Sarah A. Maithel, and John H. Whitmore. 2011. “Can Compaction Account For Lower-Than-Expected Cross-Bed Dips In The Coconino Sandstone (Permian), Arizona?” Geological Society of America Abstracts with Programs 43, no. 5: 430.
Francischini, Heitor, Paula Dentzien-Dias, Spencer G. Lucas, and Cesar L. Schultz. 2018. “Tetrapod Tracks In Permo–Triassic Eolian Beds Of Southern Brazil (Paraná Basin).” PeerJ 6: e4764. https://doi.org/10.7717/peerj.4764.
Francischini, Heitor, Spencer G. Lucas, Sebastian Voigt, Lorenzo Marchetti, Vincent L. Santucci, Cassandra L. Knight, John R. Wood, Paula Dentzien-Dias, and Cesar L. Schultz. 2019. “On The Presence Of Ichniotherium In The Coconino Sandstone (Cisuralian) Of The Grand Canyon And Remarks On The Occupation Of Deserts By Non-Amniote Tetrapods.” Paläontologische Zeitschrift (13 May). https://doi.org/10.1007/s12542-019-00450-5.
Fryberger, Steven G. 1979. “Eolian-Fluviatile (Continental) Origin Of Ancient Stratigraphic Trap For Petroleum In The Weber Sandstone, Rangely Oil Field, Colorado.” The Mountain Geologist 16: 1–36.
Fryberger, Steven G. 1984. “The Permian Upper Minnelusa Formation, Wyoming: Ancient Example Of An Offshore-Prograding Eolian Sand Sea With Geomorphic Facies, And System-Boundary Traps For Petroleum.” In The Permian and Pennsylvanian Geology of Wyoming. 35th Annual Field Conference Guidebook. Edited by J. Goolsby and D. Morton, 241–271. Casper, Wyoming: Wyoming Geological Association.
Garner, Paul A., and John H. Whitmore. 2011. “What Do We Know About Marine Sand Waves? A Review Of Their Occurrence, Morphology And Structure.” Geological Society of America Abstracts with Programs 43, no. 5: 596.
Gehrels, George E., Ron Blakey, Karl E. Karlstrom, J. Michael Timmons, Bill Dickinson, and Mark Pecha. 2011. “Detrital Zircon U-Pb Geochronology Of Paleozoic Strata In The Grand Canyon, Arizona.” Lithosphere 3, no. 3 (June 1): 183–200.
Gibling, Martin R., N. Culshaw, M. C. Rygel, and V. Pascucci. 2008. “The Maritimes Basin Of Atlantic Canada: Basin Creation And Destruction In The Collisional Zone Of Pangea.” In The Sedimentary Basins of the U.S. and Canada. Sedimentary Basins of the World Volume 5. Edited by Andrew D. Miall, 211–244. Amsterdam, Netherlands: Elsevier.
Gilluly, James, J. R. Cooper, and J. S. Williams. 1954. “Late Paleozoic Stratigraphy Of Central Cochise County Arizona.” U.S. Geological Survey Professional Paper 266: 1–49.
Glennie, K. W. 1972. “Permian Rotliegendes Of Northwest Europe Interpreted In Light Of Modern Desert Sedimentation Studies.” Bulletin of the American Association of Petroleum Geologists 56, no. 6 (June): 1048– 1071.
Gregory, J. W. 1915. “The Permian And Triassic Rocks Of Arran.” Transactions of the Geological Society of Glasgow 15: 174–187.
Harms, J. C. 1974. “Brushy Canyon Formation, Texas: A Deep Water Density Current Deposit.” Geological Society of America Bulletin 85, no. 11 (November): 1763–1784.
Hartig, Katherine A., Gerilyn S. Soreghan, Robert H. Goldstein, and Michael H. Engel. 2011. “Dolomite In Permian Paleosols Of The Bravo Dome CO2 Field, U.S.A.: Permian Reflux Followed By Late Recrystallization At Elevated Temperature.” Journal of Sedimentary Research 81, no. 4 (April): 248–265.
Hills, J. M., and F. E. Kottlowski. 1983. Correlation of Stratigraphic Units in North America—Southwest/ Southwest Mid-Continent Region [SSMC] Correlation Chart. American Association of Petroleum Geologists.
Hintze, L.F. 1985. Correlation of Stratigraphic Units in North America (COSUNA)—Great Basin Region [GB] Correlation Chart. American Association of Petroleum Geologists.
Hintze, L. F. 1986. “Stratigraphy And Structure Of The Beaver Dam Mountains, Southwestern Utah.” In Thrusting and Extensional Structures and Mineralization in the Beaver Dam Mountains, Southwestern Utah. 1986 Annual Field Conference. Edited by Dana T. Griffen, and William Revell Phillips, 1–26. Salt Lake City, Utah: Utah Geological Association.
Hintze, Lehi F. 1988. Geologic History Of Utah. Provo, Utah: Brigham Young University. Geology Studies Special Publication 7.
Hoare, R. D., and J. D. Burgess. 1960. “Fauna From The Tensleep Sandstone In Wyoming.” Journal of Paleontology 34, no. 4 (July): 711–716.
Hovland, M., H. Rueslåtten, H. K. Johnsen, B. Kvamme, and T. Kuznetsova. 2006. “Salt Formation Associated With Sub-Surface Boiling And Supercritical Water.” Marine and Petroleum Geology 23, no. 8 (September): 855–869.
Hovland, Martin, Håkon Rueslåtten, and Hans Konrad Johnsen. 2014. “Buried Hydrothermal Systems: The Potential Role Of Supercritical Water, ‘ScriW,’ In Various Geological Processes And Occurrences In The Sub-Surface.” American Journal of Analytical Chemistry 5, no. 2 (January): 128–139.
Howell, John A., and Nigel P. Mountney. 1997. “Climatic Cyclicity And Accommodation Space In Arid To Semi-Arid Depositional Systems: An Example From The Rotliegend Group Of The UK Southern North Sea.” In Petroleum Geology Of The Southern North Sea: Future Potential. Geological Society Special Publication 123. Edited by Karen P. Ziegler, Peter Turner, and Stephen R. Daines, 63–86. Bath, United Kingdom: Geological Society of London.
Hoyt, John Harger and John Chronic. 1961. “Wolfcampian Fusulinid From Ingleside Formation, Owl Canyon, Colorado.” Journal of Paleontology 35, no. 5 (1 September): 1098.
Hoyt, John H. and John Chronic. 1962. “Atokan Fusulinids From The Casper Formation, East Flank Of the Laramie Mountains, Wyoming.” Journal of Paleontology 36, no. 1 (January): 161–164.
Hubert, John F. 1960. “Petrology Of The Fountain And Lyons Formations, Front Range, Colorado.” Quarterly of the Colorado School of Mines 55, no. 1: 1–242.
Hunter, Ralph E. 1977. “Basic Types Of Stratification In Small Eolian Dunes.” Sedimentology 24, no. 3 (June): 361–387.
Hunter, Ralph E. 1981. “Stratification Styles In Eolian Sandstones: Some Pennsylvanian To Jurassic Examples From The Western Interior U.S.A.” In Recent and Ancient Nonmarine Depositional Environments: Models for Exploration. Edited by F. G. Ethridge, and R. M. Flores, 315–329. Tulsa, Oklahoma: Society of Economic Paleontologists and Mineralogists, Special Publication 31.
Hurst, Andrew, and Kenneth W. Glennie. 2008. “Mass-Wasting Of Ancient Aeolian Dunes And Sand Fluidization During A Period Of Global Warming And Inferred Brief High Precipitation: The Hopeman Sandstone (Late Permian), Scotland.” Terra Nova 20, no. 4 (August): 274–279.
Irwin, C. Dennis. 1971. “Stratigraphic Analysis Of Upper Permian And Lower Triassic Strata In Southern Utah.” The American Association of Petroleum Geologists Bulletin 55, no. 11 (November): 1976–2007.
Irwin, C. Dennis. 1976. “Permian And Lower Triassic Reservoir Rocks Of Central Utah.” In Geology of the Cordilleran Hingeline. Edited by J. Gilmore Hill, 193–202. Denver, Colorado: Rocky Mountain Association of Geologists.
Irwin, James Haskell, and Robert B. Morton. 1969. “Hydrogeologic Information On The Glorieta Sandstone And The Ogallala Formation In The Oklahoma Panhandle And Adjoining Areas As Related To Underground Waste Disposal.” U.S. Geological Survey Circular 630.
Isaacson, Peter E., Steven L. Bachtel, and Mark D. McFaddan. 1983. Stratigraphic Correlation of the Paleozoic and Mesozoic Rocks of Idaho. Moscow, Idaho: Idaho Department of Lands. Idaho Bureau of Mines and Geology, Information Circular 37.
James, W. C. 1992. “Sandstone Diagenesis In Mixed Siliciclastic-Carbonate Sequences: Quadrant And Tensleep Formations (Pennsylvanian), Northern Rocky Mountains.” Journal of Sedimentary Petrology 62, no. 5 (September 1): 810–824.
Johansen, Steven J. 1988. “Origins Of Upper Paleozoic Quartzose Sandstones, American Southwest.” Sedimentary Geology 56, nos. 1–4 (April): 153–166.
Jones, Fábio Herbert, Claiton Marlon dos Santos Scherer, and Juliano Kuchle. 2016. “Facies Architecture And Stratigraphic Evolution Of Aeolian Dune And Interdune Deposits, Permian Caldeirão Member (Santa Brígida Formation), Brazil.” Sedimentary Geology 337 (15 May): 133–150.
Kamola, Diane L., and Marjorie A. Chan. 1988. “Coastal Dune Facies, Permian Cutler Formation (White Rim Sandstone), Capitol Reef National Park Area, Southern Utah.” Sedimentary Geology 56, nos. 1–4 (April): 341–356.
Karpeta, W. P. 1990. “The Morphology Of Permian Palaeodunes—A Reinterpretation Of The Bridgnorth Sandstone Around Bridgnorth, England, In The Light Of Modern Dune Studies.” Sedimentary Geology 69, nos. 1–2 (November): 59–75.
Kendall, A. C. 2010. “Marine Evaporites.” In Facies Models 4. GEOtext 6. Edited by Noel P. James, and Robert W. Dalrymple, 505–539. Newfoundland, Canada: Geological Association of Canada.
Kent, Harry C., Elton L. Couch and Rex A. Knepp. 1988. Correlation of Stratigraphic Units in North America—Central and Southern Rockies Region Correlation [CSR] Chart. Tulsa, Oklahoma: American Association of Petroleum Geologists.
Kerr, Dennis R., and Robert H. Dott Jr. 1988. “Eolian Dune Types Preserved In The Tensleep Sandstone (Pennsylvanian-Permian), North-Central Wyoming.” Sedimentary Geology 56, nos. 1–4 (April): 383–402.
Kerr, Dennis R., David M. Wheeler, David J. Rittersbacher, and John C. Horne. 1986. “Stratigraphy And Sedimentology Of The Tensleep Sandstone (Pennsylvanian And Permian), Bighorn Mountains, Wyoming.” Earth Science Bulletin 19, nos. 1–2: 61–77.
Kiersnowski, H., and Arkadiusz Buniak. 2016. “Sand Sheets Interaction With Aeolian Dune, Alluvial And Marginal Playa Beds In Late Permian Upper Rotliegend Setting (Western Part Of The Poznań Basin, Poland).” Geological Quarterly 60, no. 4: 771–800.
King, Philip B. 1948. “Geology Of The Southern Guadalupe Mountains, Texas.” U.S. Geological Survey Professional Paper 215: 1–183.
Knight, Susan H. 1929. “The Fountain And The Casper Formations Of The Laramie Basin.” University of Wyoming Publications in Science (Geology) 1: 1–82.
Kocurek, Gary. and Karen G. Havholm. 1993. “Eolian Sequence Stratigraphy—A Conceptual Framework.” In Siliciclastic Sequence Stratigraphy: Recent Developments and Applications, 393–409. AAPG Memoir 58. Edited by Paul Weimer and Henry Posamentier. Tulsa, Oklahoma.
Kocurek, Gary, and Brenda L. Kirkland. 1998. “Getting To The Source: Aeolian Influx To The Permian Delaware Basin Region.” Sedimentary Geology 117, nos. 3–4 (May): 143–149.
Koelmel, M. H. 1986. “Post-Mississippian Paleotectonic, Stratigraphic, And Diagenetic History Of The Weber Sandstone In The Rangely Field Area, Colorado. In Paleotectonics and Sedimentation in the Rocky Mountain Region, United States. Edited by James A. Peterson. AAPG Memoir 41: 371–396. Tulsa, Oklahoma: The American Association of Petroleum Geologists.
Krainer, K., and S. G. Lucas. 2015. “Type Section Of The Lower Permian Glorieta Sandstone, San Miguel County, New Mexico.” In Guidebook 66—Geology of the Las Vegas Area. 66th Annual Fall Field Conference Guidebook. Edited by Jennifer Lindline, Michael Petronis, and Joseph Zebrowski, 205–210. Socorro, New Mexico: New Mexico Geological Society, .
Krapovickas, V., A. C. Mancuso, A. B. Arcucci, and A. T. Caselli. 2010. “Fluvial And Eolian Ichnofaunas From The Lower Permian Of South America (Patquía Formation, Paganzo Basin).” Geologica Acta 8, no. 4: 449–462.
Lane, Donald W. 1973. The Phosphoria and Goose Egg Formations in Wyoming. Preliminary Report No. 12. Laramie, Wyoming: The Geological Survey of Wyoming.
Langenheim, Ralph L. Jr., and E. R. Larson. 1973. Correlation of Great Basin Stratigraphic Units, Bureau of Mines and Geology Bulletin 72. Reno, Nevada: MacKay School of Mines, University of Nevada.
Larsen, Bjørn T., Snorre Olaussen, Bjørn Sundvoll, and Michel Heeremans. 2008. “The Permo-Carboniferous Oslo Rift Through Six Stages And 65 Million Years.” Episodes 31, no. 1 (March): 52–58.
Lavoie, D., N. Pinet, J. Dietrich, P. Hannigan, S. Castonguay, A. P. Hamblin, and P. Giles. 2009. “Petroleum Resource Assessment, Paleozoic Successions Of The St. Lawrence Platform And Appalachians Of Eastern Canada.” Geological Survey of Canada Open File 6174.
Lawton, Timothy F., Cody D. Buller, and Todd R. Parr. 2015. “Provenance Of A Permian Erg On The Western Margin Of Pangea: Depositional System Of The Kungurian (Late Leonardian) Castle Valley And White Rim Sandstones And Subjacent Cutler Group, Paradox Basin, Utah, USA.” Geosphere 11, no. 5 (October 1): 1475–1506.
Lee, W. T. 1927. “Correlation Of Geologic Formations Between East-Central Colorado, Central Wyoming And Southern Montana.” U.S. Geological Survey Professional Paper 149: 1–80.
Lee, W. T., and G. H. Girty. 1909. The Manzo Group of the Rio Grande Valley, New Mexico. U.S. Geological Survey Bulletin 389: 1–141.
Lessentine, Ross H. 1965. “Kaiparowits And Black Mesa Basins: Stratigraphic Synthesis.” Bulletin of the American Association of Petroleum Geologists 49, no. 11 (November): 1997–2019.
Limarino, C. O., and L. A. Spalletti. 1986. “Eolian Permian Deposits In West And Northwest Argentina.” Sedimentary Geology 49, nos. 1–2 (August): 109–127.
Link, Paul K., Robert C. Mahon, Luke P. Beranek, Erin A. Campbell-Stone, and Ranie Lynds. 2014. “Detrital Zircon Provenance Of Pennsylvanian To Permian Sandstones From The Wyoming Craton And Wood River Basin, Idaho, U.S.A.” Rocky Mountain Geology 49, no. 2 (January 1): 115–136.
Lippman, F. 1973. Sedimentary Carbonate Minerals. New York: Springer-Verlag.
Loope, David B. 1984. “Eolian Origin Of Upper Paleozoic Sandstones, Southeastern Utah.” Journal of Sedimentary Petrology 54, no. 2 (June 1): 563–580.
Lovell, M. A., P. D. Jackson, P. K. Harvey, and R. C. Flint. 2006. “High Resolution Petrophysical Characterization Of Samples From An Aeolian Sandstone: The Permian Penrith Sandstone of NW England.” In Fluid Flow and Solute Movement in Sandstones: The Onshore UK Permo-Triassic Red Bed Sequence. Geological Society of London Special Publication 263. Edited by R. D. Barker, and J. H. Tellam, 49–63. Bath, United Kingdom: The Geological Society Publishing House.
Luepke, Gretchen. 1971. “A Re-Examination Of The Type Section Of The Scherrer Formation (Permian) In Cochise County, Arizona.” Arizona Geological Society Digest 9: 245–257.
Luepke, Gretchen. 1976. “Archaeocidaris Spines From The Permian Scherrer Formation, Southeastern Arizona.” Journal of the Arizona Academy of Science 11, no. 3 (October): 87–88.
Macfarlane, P. Allen, J. Combes, S. Turbek, D. S. Kirschen, and S. Yoder. 1993. [2010 update by J. J. Woods]. “Thickness Of The Cedar Hills Sandstone Aquifer” [Map]. Kansas Geological Survey Open File Report 93-1A, Plate 16.
Mack, G. H. 1977. “Depositional Environments Of The Cutler-Cedar Mesa Facies Transition (Permian) Near Moab, Utah.” The Mountain Geologist 14, no. 2 (April): 53–68.
MacLachlan, James, and Alan Bieber. 1963. “Permian And Pennsylvanian Geology Of the Hartville Uplift—Alliance Basin—Chadron Arch Area.” In Guidebook to the Geology of the Northern Denver Basin and Adjacent Uplifts. Guidebook to the Fourteenth Field Conference. Edited by Dudley W. Bolyard, and Philip J. Katich, 84–94. Denver, Colorado: Rocky Mountain Association of Geologists.
Mader, Detlef, and Michael John Yardley. 1985. “Migration, Modification And Merging In Aeolian Systems And The Significance Of The Depositional Mechanisms In Permian And Triassic Dune Sands Of Europe And North America.” Sedimentary Geology 43, nos. 1–4 (April): 85–218.
Maher, John C. ed. 1960. Stratigraphic Cross Section of Paleozoic Rocks West Texas to Northern Montana. Tulsa, Oklahoma: The American Association of Petroleum Geologists.
Maher, John C. 1954. “Lithofacies And Suggested Depositional Environment Of Lyons Sandstone And Lykins Formation In Southeastern Colorado.” Bulletin of the American Association of Petroleum Geologists 38 (October): 2233– 2239.
Maher, John Charles, and Jack B. Collins. 1953. “Permian And Pennsylvanian Rocks Of Southeastern Colorado And Adjacent Areas.” U.S. Geological Survey, Oil and Gas Investigations Map OM 135.
Mainguet, Monique. 1978. “The Influence Of Trade Winds, Local Air-Masses And Topographic Obstacles On The Aeolian Movement Of Sand Particles And The Origin And Distribution Of Dunes And Ergs In The Sahara And Australia.” Geoforum 9, no. 1: 17–28.
Maithel, S. A. 2019. “Characterization Of Cross-Bed Depositional Processes In The Coconino Sandstone.” PhD Dissertation. Loma Linda, California: Loma Linda University School of Medicine in conjunction with the Faculty of Graduate Studies.
Maithel, Sarah A., Paul A. Garner, and John H. Whitmore. 2015. “Preliminary Assessment Of The Petrology Of The Hopeman Sandstone (Permo-Triassic), Moray Firth Basin, Scotland.” Scottish Journal of Geology 51, no. 2 (November): 177–184.
Mallory, William W. ed. 1972a. Geologic Atlas of the Rocky Mountain Region. Denver, Colorado: Rocky Mountain Association of Geologists.
Mallory, W. W. 1972b. “Regional Synthesis Of The Pennsylvanian System.” In Geologic Atlas of the Rocky Mountain Region. Edited by W. W. Mallory, 111–127. Denver, Colorado: Rocky Mountain Association of Geologists.
Mancuso, Adriana Cecilia, Veronica Krapovickas, Claudia Marsicano, Cecilia Benavente, Dario Benedito, Marcelo De La Fuente, and Eduardo G. Ottone. 2016. “Tetrapod Tracks Taphonomy In Eolian Facies From The Permian Of Argentina.” Palaios 31, no. 8 (August): 374–388.
Mankiewicz, David, and James R. Steidtmann. 1979. “Depositional Environments And Diagenesis Of The Tensleep Sandstone, Eastern Big Horn Basin, Wyoming.” In Aspects of Diagenesis. Special Publication No. 26. Edited by Peter A. Scholle, and Paul R. Schluger, 319–336. Tulsa, Oklahoma: Society of Economic Paleontologists and Mineralogists.
Mankin, Charles J. 1986. Correlation of Stratigraphic Units in North America. Texas-Oklahoma Tectonic Region [TOT] Correlation Chart. American Association of Petroleum Geologists.
Marchetti, Lorenzo, Sebastian Voigt, Spencer G. Lucas, Heitor Francischini, Paula Dentzien-Dias, Roberto Sacchi, Marco Mangiacotti, Stefano Scali, Andrea Gazzola, Ausonio Ronchi, and Amanda Millhouse. 2019. “Tetrapod Ichnotaxonomy In Eolian Paleoenvironments (Coconino And De Chelly Formations, Arizona) And Late Cisuralian (Permian) Sauropsid Radiation.” Earth-Science Reviews 190 (March): 148–170.
Marzolf, John E. 1988. “Controls On Late Paleozoic And Early Mesozoic Eolian Deposition Of The Western United States.” Sedimentary Geology 56, nos. 1–4 (April): 167–191.
Maughan, Edwin K., and Albert E. Roberts. 1967. “Big Snowy And Amsden Groups And The Mississippian-Pennsylvanian Boundary In Montana.” USGS Professional Paper 554-B: 1–27.
Maughan, E. K., and R. F. Wilson. 1960. “Pennsylvanian And Permian Strata In Southern Wyoming And Northern Colorado.” In Guide to the Geology of Colorado. Edited by R. J. Weimer, and J. D. Haun, 34–42. Denver, Colorado: Geological Society of America, Rocky Mountain Association of Geologists, and Colorado Scientific Society.
Maughan, Edwin K., and Thomas S. Ahlbrandt. 1985. “Pennsylvanian And Permian Eolian Sandstone Facies, Northern Colorado And Southeastern Wyoming.” In Rocky Mountain Section Field Trip Guide. Edited by D. L. Macke, and E. K. Maughan, 99–113. Denver, Colorado: American Association of Petroleum Geologists, Rocky Mountain Section; Society of Economic Paleontologists and Mineralogists, Rocky Mountain Section; Rocky Mountain Association of Geologists, National Energy Minerals Division.
Mazzullo, Jim, Ariel Malicse, and Joel Siegel. 1991. “Facies And Depositional Environments Of The Shattuck Sandstone On The Northwest Shelf Of The Permian Basin.” Journal of Sedimentary Petrology 61, no. 6 (November): 940–958.
McCauley, Victor T. 1956. “Pennsylvanian And Lower Permian Of The Williston Basin.” In Williston Basin Symposium. Proceedings of the 1st International Williston Basin Symposium, 150–164. Bismarck, North Dakota: North Dakota Geological Society and Saskatchewan Geological Society.
McKee, Edwin D. 1934. “The Coconino Sandstone—Its History And Origin.” In Papers Concerning the Palaeontology of California, Arizona and Idaho, 77–115. Carnegie Institute of Washington, Publication 440.
McKee, Edwin D. 1982. “The Supai Group Of Grand Canyon.” U.S. Geological Survey Professional Paper 1173:1-504.
McKee, Edwin D., and Joao J. Bigarella. 1979. “Ancient Sandstones Considered To Be Eolian.” In A Study of Global Sand Seas. Edited by Edwin D. McKee, 187–238. U.S. Geological Survey Professional Paper 1052.
McKee, E. D., and S. S. Oriel eds. 1967. “Paleotectonic Investigations Of The Permian System In The United States.” U.S. Geological Survey Professional Paper 515.
McKeever, Patrick J. 1991. “Trackway Preservation In Eolian Sandstones From The Permian Of Scotland.” Geology 19, no. 7 (July 1): 726–729.
McKelvey, V. E. et al. 1959. The Phosphoria, Park City And Shedhorn Formations In The Western Phosphate Field. U.S. Geological Survey Professional Paper 313-A:1-47.
McKnight, Edwin T. 1940. “Geology Of Area Between Green And Colorado Rivers Grand And San Juan Counties Utah.” Bulletin of the U.S. Geological Survey 908: 1–147.
McNair, Andrew H. 1951. “Paleozoic Stratigraphy Of Part Of Northwestern Arizona.” Bulletin of the American Association of Petroleum Geologists 35, no. 1 (March): 503–541.
Meibos, Lynn C. 1981. “Structure And Stratigraphy Of The Nephi NW 7½-Minute Quadrangle, Juab County, Utah.” Brigham Young University, Geology Studies 30, no. 1: 37–58.
Melton, Frank A. 1925. “Correlation Of Permo-Carboniferous Red Beds In Southwestern Colorado And Northern New Mexico.” Journal of Geology 33, no. 8 (November– December): 807–815.
Melvin, John, Ronald A. Sprague, and Christian J. Heine. 2010. “From Bergs To Ergs: The Late Paleozoic Gondwanan Glaciation And Its Aftermath In Saudi Arabia.” In Late Paleozoic Glacial Events and Postglacial Transgressions in Gondwana. Edited by Oscar R. López-Gamundí, and Luis A. Buatois, 37–80. Denver, Colorado: Geological Society of America Special Paper 468.
Middleton, Larry T., David K. Elliott, and Michael Morales. 2003. “Coconino Sandstone.” In Grand Canyon Geology. 2nd ed. Edited by Stanley S. Beus, and Michael Morales, 163–179. New York: Oxford University Press.
Miller, F. K., and McKee, E. H. 1971. “Thrust And Strike-Slip Faulting In The Plomosa Mountains, Southwestern Arizona.” Geological Society of America Bulletin 82, no. 3 (March): 717–722.
Mitchell, Gary C. 1985. “The Permian-Triassic Stratigraphy Of The Northwest Paradox Basin Area, Emery, Garfield, And Wayne Counties, Utah.” The Mountain Geologist 22, no. 4 (October): 149–166.
Moore, Raymond C., John C. Frye, J. M. Jewett, Wallace Lee, and Howard G. O’Connor. 1951. The Kansas Rock Column. University of Kansas Publications, State Geological Survey of Kansas, Bulletin 89.
Morrow, D.W. 1982. “Diagenesis 1. Dolomite–Part 1: The Chemistry Of Dolomitization And Dolomite Precipitation.” Geoscience Canada 9, no. 1 (March): 5–13.
Mountney, Nigel P., and Alison Jagger. 2004. “Stratigraphic Evolution Of An Aeolian Erg Margin System: The Permian Cedar Mesa Sandstone, SE Utah, USA.” Sedimentology 51, no. 4 (August): 713–743.
Myers, Donald A. 1972. “The Upper Paleozoic Madera Group In The Manzano Mountains, New Mexico.” U.S. Geological Survey Bulletin 1372-F: 1–13.
Needham, C. E., and Robert L. Bates. 1943. “Permian Type Sections In Central New Mexico.” Bulletin of the Geological Society of America 54, no. 11 (November 1): 1653–1668.
Newell, A. J. 2001. “Bounding Surfaces In A Mixed Aeolian-Fluvial System (Rotliegend, Wessex Basin, SW UK).” Marine and Petroleum Geology 18, no. 3 (March): 339–347.
Nielson, R. LaRell. 1994. “Stratigraphic Analysis Of The Diamond Creek Sandstone (Permian) In Central Utah.” Geological Society of America Abstracts with Programs, Rocky Mountain Section 26, no. 6: 57.
Nielson, R. Larell. 1999. “Stratigraphy And Origin Of Breccia Deposits In The Kirkman Formation, Central Utah.” In Geology of Northern Utah and Vicinity. Utah Geological Association Publication 27. Edited by Lawrence E. Spangler, and Constance J. Allen, 111–121. Salt Lake City, Utah: Utah Geological Association.
Oard, Michael J. 2010a. “Is The Geological Column A Global Sequence?” Journal of Creation 24, no. 1 (April): 56–64.
Oard, Michael J. 2010b. “The Geological Column Is A General Flood Order With Many Exceptions.” Journal of Creation 24, no. 2 (August): 78–82.
Ogilvie, S., K. Glennie, and C. Hopkins. 2000. “Excursion Guide 13: The Permo-Triassic Sandstones Of Morayshire, Scotland.” Geology Today 16, no. 5 (September–October): 185–190.
Ohlen, H. R., and L. B. McIntyre. 1965. “Stratigraphy And Tectonic Features Of Paradox Basin, Four Corners Area.” Bulletin of the American Association of Petroleum Geologists 49, no. 11 (November): 2020–2040.
Opkyke, N. D., and S. K Runcorn. 1960. “Wind Direction In The Western United States In The Late Paleozoic.” Bulletin of the Geological Society of America 71, no. 7 (July): 959–972.
Parrish, Judith Totman, and Fred Peterson. 1988. “Wind Directions Predicted From Global Circulation Models And Wind Directions Determined From Eolian Sandstones Of The Western United States.” Sedimentary Geology 56, nos. 1–4 (April): 261–282.
Peacock, J. D. 1966. “VII.—Contorted Beds In The Permo-Triassic Aeolian Sandstones Of Morayshire.” Bulletin of the Geological Society of Great Britain 24: 157–162.
Peacock, J. D., N. G. Berridge, A. L. Harris, and F. May. 1968. The Geology of the Elgin District. South Kensington, London: Institute of Geological Sciences.
Peirce, H. W., N. Jones, and R. Rogers. 1977. A Survey of Uranium Favorability of Paleozoic Rocks in the Mogollon Rim and Slope Region—East Central Arizona. State of Arizona Bureau of Geology and Mineral Technology, Circular 19. Tucson, Arizona: State of Arizona Bureau of Geology and Mineral Technology.
Peterson, Fred. 1988. “Pennsylvanian To Jurassic Eolian Transportation Systems In The Western United States.” Sedimentary Geology 56, nos. 1–4: 207–260.
Peterson, James A. 1980. “Permian Paleogeography And Sedimentary Provinces, West Central United States.” In Paleozoic Paleogeography of the West-Central United States. Edited by T. D. Fouch, and E. R. Magathan, 271– 292. Denver, Colorado: The Rocky Mountain Section of the Society of Economic Paleontologists and Mineralogists, Rocky Mountain Paleogeography Symposium 1.
Pike, James D., and Dustin E. Sweet. 2018. “Environmental Drivers Of Cyclicity Recorded In Lower Permian Eolian Strata, Manitou Springs, Colorado, Western United States.” Palaeogeography, Palaeoclimatology, Palaeoecology 499 (15 June): 1–12.
Piper, D. J. W. 1970. “Eolian Sediments In The Basal New Red Sandstone Of Arran.” Scottish Journal of Geology 6 (1 November): 295–308.
Poland, Zachary A., and Alexander R. Simms. 2012. “Sedimentology Of An Erg To An Erg-Margin Depositional System, The Rush Springs Sandstone Of Western Oklahoma, U.S.A.: Implications For Paleowinds Across Northwestern Pangea During The Guadalupian (Middle Permian).” Journal of Sedimentary Research 82, no. 5 (May): 345–363.
Poole, F. G. 1962. “Wind Directions In Late Paleozoic To Middle Mesozoic Time On The Colorado Plateau.” In Short Papers in Geology Hydrogoloy, and Topography Articles 120–179, 147–151. U.S. Geological Survey Professional Paper 450-D.
Poppe, L. J., J. F. Denny, S. J. Williams, M. S. Moser, N. A. Forfinski, H. F. Stewart, and E. F. Doran. 2007. “The Geology Of Six Mile Reef, Eastern Long Island Sound.” U.S. Geological Survey Open-File Report 2007-1191.
Poppe, L. J., K. Y. McMullen, S. D. Ackerman, D. S. Blackwood, J. D. Schaer, M. R. Forrest, A. J. Ostapenko, and E. F. Doran. 2011. “Sea-Floor Geology And Topography Offshore In Eastern Long Island Sound.” U.S. Geological Survey Open-File Report 2010-1150.
Poppe, L. J., M. L. DiGiacomo-Cohen, S. M. Smith, H. F. Stewart, and N. A. Forfinski. 2006. “Seafloor Character And Sedimentary Processes In Eastern Long Island Sound And Western Block Island Sound.” Geo-Marine Letters 26 (June): 59–68.
Pryor, Wayne A. 1971. “Petrology Of The Permian Yellow Sands Of Northeastern England And Their North Sea Basin Equivalents.” Sedimentary Geology 6, no. 4 (December): 221–254.
Rascoe, Bailey Jr., and Donald L. Baars. 1972. “Permian System.” In Geologic Atlas of the Rocky Mountain Region. Edited by W. W. Mallory, 143–165. Denver, Colorado: Rocky Mountain Association of Geologists.
Rawson, Richard R., and Christine E. Turner-Peterson. 1980. “Paleogeography Of Northern Arizona During The Deposition Of The Permian Toroweap Formation.” In Paleozoic Paleogeography of the West-Central United States. Edited by T. D. Fouch, and E. R. Magathan, 341– 352. Denver, Colorado: The Rocky Mountain Section of the Society of Economic Paleontologists and Mineralogists, Rocky Mountain Paleogeography Symposium 1.
Read, Charles B., and Gordon H. Wood. 1947. “Distribution And Correlation Of Pennsylvanian Rocks In Late Paleozoic Sedimentary Basins Of Northern New Mexico.” Journal of Geology 55, no. 3, part 2 (May): 220–236.
Reed, John K., and Carl R. Froede Jr. 2003. “The Uniformitarian Stratigraphic Column: Shortcut Or Pitfall For Creation Geology?” Creation Research Society Quarterly 40, no. 2 (September): 90–98.
Reeves, Frank. 1931. “Geology Of The Big Snowy Mountains, Montana.” U.S. Geological Survey Professional Paper 165: 135–149.
Reiche, Parry. 1938. “An Analysis Of Cross-Lamination The Coconino Sandstone.” Journal of Geology 46. no. 7 (October– November): 905–932.
Richards, R. W., and G. R. Mansfield. 1912. “The Bannock Overthrust. A Major Fault In Southeastern Idaho And Northeastern Utah.” Journal of Geology 20, no. 8 (November–December): 681–709.
Roberts, Ralph J., M. D. Crittenden Jr., E. W. Tooker, H. T. Morris, R. K. Hose, and T. M. Cheney. 1965. “Pennsylvanian And Permian Basins In Northwestern Utah, Northeastern Nevada And South-Central Idaho.” Bulletin of the American Association of Petroleum Geologists 49, no. 11 (November): 1926–1956.
Rodríguez-López, Juan Pedro, Lars B. Clemmensen, Nick Lancaster, Nigel P. Mountney, and Gonzalo D. Veiga. 2014. “Archean To Recent Aeolian Sand Systems And Their Sedimentary Record: Current Understanding And Future Prospects.” Sedimentology 61, no. 6 (October): 1487–1534.
Ross, Marcus R., William A. Hoesch, Steven A. Austin, John H. Whitmore, and Timothy L. Clarey. 2010. “Garden Of The Gods At Colorado Springs: Paleozoic And Mesozoic Sedimentation And Tectonics.” In Through the Generations: Geologic and Anthropogenic Field Excursions in the Rocky Mountains from Modern to Ancient. Edited by Lisa A. Morgan, and Steven L. Quane, 77–93. Denver, Colorado: Geological Society of America. Field Guide 18.
Roth, Arial A. 2009. “‘Flat Gaps’ In Sedimentary Rock Layers Challenge Long Geologic Ages.” Journal of Creation 23, no. 2 (August): 76–81.
Sando, William J., and Charles A. Sandberg. 1987. “New Interpretations Of Paleozoic Stratigraphy And History In The Northern Laramie Range And Vicinity, Southeast Wyoming.” U.S. Geological Survey Professional Paper 1450: 1–39.
Sando, William J., Mackenzie Gordon Jr., J. Thomas Dutro Jr. 1975. “Stratigraphy And Geologic History Of The Amsden Formation (Mississippian And Pennsylvanian) Of Wyoming.” U.S. Geological Survey Professional Paper 848: A1–A83.
Saperstone, Herb I., and Frank G. Ethridge. 1984. “Origin And Paleotectonic Setting Of The Pennsylvanian Quadrant Sandstone, Southwestern Montana.” In The Permian and Pennsylvanian Geology of Wyoming. 35th Annual Field Conference Guidebook. Edited by J. Goolsby, and D. Morton, 309–331. Casper, Wyoming: Wyoming Geological Association.
Scott, George L., and William Eugene Ham. 1957. Geology and Gypsum Resources of the Carter Area, Oklahoma. Norman, Oklahoma: Oklahoma Geological Survey. Circular 42.
Shotton, F. W. 1937. “The Lower Bunter Sandstones Of North Worcestershire And East Shropshire.” Geological Magazine 74, no. 12 (December): 534–553.
Shotton, F. W. 1956. “Some Aspects Of The New Red Desert In Britain.” Geological Journal 1, no. 5: 450–465.
Siemers, W. Aaron, Thomas M. Stanley, and Neil H. Suneson. 2000. “Geology Of Arcadia Lake Parks—An Introduction And Field-Trip Guide.” Oklahoma Geology Notes 60, no. 1 (Spring): 4–17.
Skipp, Betty, and Theodore R. Brandt. 2012. Geologic Map of the Fish Creek Reservoir 7.5´ Quadrangle, Blaine County, Idaho. U.S. Geological Survey, Scientific Investigations Map 3191.
Skipp, B., R.D. Hoggan, D.L. Schleicher, and R.C. Douglass. 1979. “Upper Paleozoic Carbonate Bank In East-Central Idaho─Snaky Canyon, Bluebird Mountain, And Arco Hills Formations, And Their Paleotectonic Significance.” U.S. Geological Survey Bulletin 1486: 1–78.
Smith, George Varty. 1884. “On Further Discoveries Of The Footprints Of Vertebrate Animals In The Lower New Red Sandstone Of Penrith.” Quarterly Journal of the Geological Society of London 40, nos. 1–4 (January): 479–481.
Soreghan, Gerilyn S., and Michael J. Soreghan. 2013. “Tracing Clastic Delivery To The Permian Delaware Basin, U.S.A.: Implications For Paleogeography And Circulation In Westernmost Equatorial Pangea.” Journal of Sedimentary Research 83, no. 9 (September 1): 786–802.
Spalletti, Luis A., Carlos Oscar Limarino, and Ferran Colombo. 2010. “Internal Anatomy Of An Erg Sequence From The Aeolian-Fluvial System Of The De La Cuesta Formation (Paganzo Basin, Northwestern Argentina).” Geologica Acta 8, no. 4: 431–447.
Stanesco, J. D. 1991. “Sedimentology And Cyclicity In The Lower Permian De Chelly Sandstone On The Defiance Plateau: Eastern Arizona.” The Mountain Geologist 28, no. 4 (October): 1–11.
Steele, Brenda A. 1987. “Depositional Environments Of The White Rim Sandstone Member Of The Permian Cutler Formation, Canyonlands National Park, Utah.” U.S. Geological Survey Bulletin 1592: 1–20.
Steele, Grant. 1960. “Pennsylvanian-Permian Stratigraphy Of East-Central Nevada And Adjacent Utah.” In Guidebook to the Geology of East-Central Nevada. Eleventh Annual Field Conference of the Intermountain Association of Petroleum Geologists. Edited by Jerome W. Boettcher, and William W. Sloan Jr, 91–113. Salt Lake City, Utah and Ely, Nevada: Intermountain Association of Petroleum Geologists and Eastern Nevada Geological Society.
Steele, Richard P. 1983. “Longitudinal Draa In The Permian Yellow Sands Of North-East England.” In Eolian Sediments and Processes. Edited by M. E. Brookfield, and T. S. Ahlbrandt, 543–550. Oxford, United Kingdom: Elsevier. Developments in Sedimentology 38.
Steele-Mallory, B. A. 1982. “The Depositional Environment And Petrology Of The White Rim Sandstone Member Of The Permian Cutler Formation, Canyonlands National Park, Utah.” U.S. Geological Survey Open File Report 82-204.
Steidtmann, James R. 1974. “Evidence For Eolian Origin Of Cross-Stratification In Sandstone Of The Casper Formation, Southernmost Laramie Basin, Wyoming.” Geological Society of America Bulletin 85, no. 12 (December 1): 1835–1842.
Stokes, William Lee 1986. Geology of Utah. Salt Lake City, Utah: Utah Museum of Natural History and Utah Geological and Mineral Survey.
Swezey, Christopher, Max Deynoux, and Daniel Jeannette. 1996. “Sandstone Depositional Environments Of The Upper Permian Champenay Formation, Champenay Basin, Northeastern France.” Sedimentary Geology 105, nos. 1–2 (August): 91–103.
Thompson, M. L., and Harold W. Scott. 1941. “Fusulinids From The Type Section Of The Lower Pennsylvanian Quadrant Formation.” Journal of Paleontology 15, no. 4 (July): 349–353.
Thompson, Warren O. 1949. “Lyons Sandstone Of Colorado Front Range.” Bulletin of the American Association of Petroleum Geologists 33, no. 1 (January): 52–72.
Thompson, W. O., and J. M. Kirby. 1940. “Cross Sections From Colorado Springs To Black Hills Showing Correlation Of Paleozoic Stratigraphy.” In Guide Book, Fourteenth Annual Field Conference, The Kansas Geological Society, 142–148. Wichita, Kansas: The Kansas Geological Society.
Toepelman, W. C., and H. G. Rodeck. 1936. “Footprints In Late Paleozoic Red Beds Near Boulder, Colorado.” Journal of Paleontology 10, no. 7 (October): 660–662.
Tooker, E.W., and Ralph J. Roberts. 1970. “Upper Paleozoic Rocks In The Oquirrh Mountains And Bingham Mining District, Utah.” U.S. Geological Survey Professional Paper 629: A1–A76.
Tubbs, Robert E. Jr. 1989. “Depositional History Of The White Rim Sandstone, Wayne And Garfield Counties, Utah.” The Mountain Geologist 26, no. 4 (October): 101–112.
Verille, G. J., G. A. Sanderson, and B. D. Rea. 1970. “Missourian Fusulinids From The Tensleep Sandstone, Bighorn Mountains, Wyoming.” Journal of Paleontology 44, no. 3 (1 May): 478–479.
Versey, H. C. 1925. “The Beds Underlying The Magnesian Limestone In Yorkshire.” Proceedings of the Yorkshire Geological Society 20, no. 2 (1 January): 200–214.
Verville G. J. 1957. “Wolfcampian Fusulinids From The Tensleep Sandstone In The Big Horn Mountains, Wyoming.” Journal of Paleontology 31, no. 2: 349–352.
Verville, George J., and Eric E. Thompson. 1963. “Desmoinesian Fusulinids From The Minnelusa Formation In The Southern Black Hills, South Dakota.” In Northern Powder River Basin, Wyoming and Montana Guidebook. 1st Joint Field Conference. Edited by Gerald C. Cooper, Donald F. Cardinal, Howard W. Lorenz, and John R. Lynn, 61–66. Casper, Wyoming: Wyoming Geological Association and Billings Geological Society.
Walker, T. R., and J. C. Harms. 1972. “Eolian Origin Of Flagstone Beds, Lyons Sandstone (Permian), Type Area, Boulder County, Colorado.” The Mountain Geologist 9, nos. 2–3 (April–July): 279–288.
Waugh, B. 1970a. “Formation Of Quartz Overgrowths In The Penrith Sandstone (Lower Permian) Of Northwest England As Revealed By Scanning Electron Microscopy.” Sedimentology 14, nos. 3–4: 309–320.
Waugh, Brian. 1970b. “Petrology, Provenance And Silica Diagenesis Of The Penrith Sandstone (Lower Permian) Of Northwest England.” Journal of Sedimentary Petrology 40, no. 4 (December 1): 1226–1240.
Wells, Neil A., Susan S. Richards, Shengfeng Peng, Sharon E. Keattch, Jeffrey A. Hudson, and Catherine J. Copsey. 1993. “Fluvial Processes And Recumbently Folded Crossbeds In The Pennsylvanian Sharon Conglomerate In Summit County, Ohio, U.S.A.” Sedimentary Geology 85, nos. 1–4 (May): 63–83.
Whelan, J. A. 1982. Geology, Ore Deposits And Mineralogy Of The Rocky Range, Near Milford Beaver County, Utah. Utah Geological and Mineral Survey, Special Studies 57.
Whitmore, John H., and Paul A. Garner. 2018. “The Coconino Sandstone (Permian, Arizona, USA): Implications For The Origin Of Ancient Cross-Bedded Sandstones.” In Proceedings of the Eighth International Conference on Creationism. Edited by J. H. Whitmore, 581–627. Pittsburgh, Pennsylvania: Creation Science Fellowship.
Whitmore, John H., and Ray Strom. 2010. “Sand Injectites At The Base Of The Coconino Sandstone, Grand Canyon, Arizona.” Sedimentary Geology 230, nos. 1–2 (October): 46–59.
Whitmore, John H., and Ray Strom. 2017. “Rounding Of Quartz And K-Feldspar Sand From Beach To Dune Settings Along The California And Oregon Coastlines: Implications For Ancient Sandstones.” Answers Research Journal 10 (November 15): 259–270. https://answersingenesis.org/geology/sedimentation/rounding-quartz-and-k-feldspar-sand-beach-dune-settings-along-california-oregon-coastlines/.
Whitmore, John H., and Raymond Strom. 2018. “The Significance Of Angular K-feldspar Grains In Ancient Sandstones. In Proceedings of the Eighth International Conference on Creationism. Edited by J. H. Whitmore, 628–651. Pittsburgh, Pennsylvania: Creation Science Fellowship.
Whitmore, J. H., and R. A. Peters. 1999. “Reconnaissance Study Of The Contact Between The Hermit Formation And The Coconino Sandstone, Grand Canyon, Arizona.” Geological Society of America Abstracts with Programs 31, no. 7: A-235.
Whitmore, John H., Guy Forsythe, and Paul Garner. 2015. “Intraformational Parabolic Recumbent Folds In The Coconino Sandstone (Permian) And Two Other Formations In Sedona, Arizona (USA).” Answers Research Journal 8 (January 21): 21–40. https://answersingenesis.org/geology/rock-layers/intraformational-parabolic-recumbent-folds/.
Whitmore, John H., Raymond Strom, Stephen Cheung, and Paul Garner. 2014. “The Petrology Of The Coconino Sandstone (Permian), Arizona.” Answers Research Journal 7 (December 10): 499–532. https://answersingenesis.org/geology/rock-layers/petrology-of-the-coconino-sandstone/.
Wilson, I. G. 1973. “Ergs.” Sedimentary Geology 10, no. 2 (October): 77–106.
Woodmorappe, J. 1981. “The Essential Nonexistence Of The Evolutionary-Uniformitarian Geologic Column: A Quantitative Assessment.” Creation Research Society Quarterly 18, no. 1 (June): 46–71.
Wright, David T. 1999. “The Role Of Sulphate-Reducing Bacteria And Cyanobacteria In Dolomite Formation In Distal Ephemeral Lakes Of The Coorong Region, South Australia.” Sedimentary Geology 126, nos. 1–4: 147–157.
Yancey, Thomas E., Gary D. Ishibashi, and Paul T. Bingman. 1980. “Carboniferous And Permian Stratigraphy Of The Sublett Range, South-Central Idaho.” In Paleozoic Paleogeography of the West-Central United States. Edited by Thomas D. Fouch, and Esther R. Magathan, 259–269. Denver, Colorado: The Rocky Mountain Section of the Society of Economic Paleontologists and Mineralogists, Rocky Mountain Paleogeography Symposium 1.
Zeller, Robert A. 1965. Stratigraphy of the Big Hatchet Mountains Area, New Mexico. Socorro, New Mexico: State Bureau of Mines and Mineral Resources, New Mexico Institute of Mining and Technology, Memoir 16.
Appendix 1
A comparison of currently recognized chronostratigraphic (time-rock) designations for the Pennsylvanian and Permian by the International Commission on Stratigraphy (column 1), Global Chronostratigraphic Units at the time the COSUNA project was published (column 2) and North American Stratigraphic Units at the time the COSUNA project was published (column 3). Conventional ages (Ma) are shown on the right and apply to all three columns. Note that this table is not meant to show stage equivalencies of the columns because the accepted boundary dates fluctuate. For example, “Wolfcampian” in column 3 is not equivalent to the Artinskian and Kunguian in column 1. Wolfcampian rocks would include the Asselian and Sakarian of column 1.
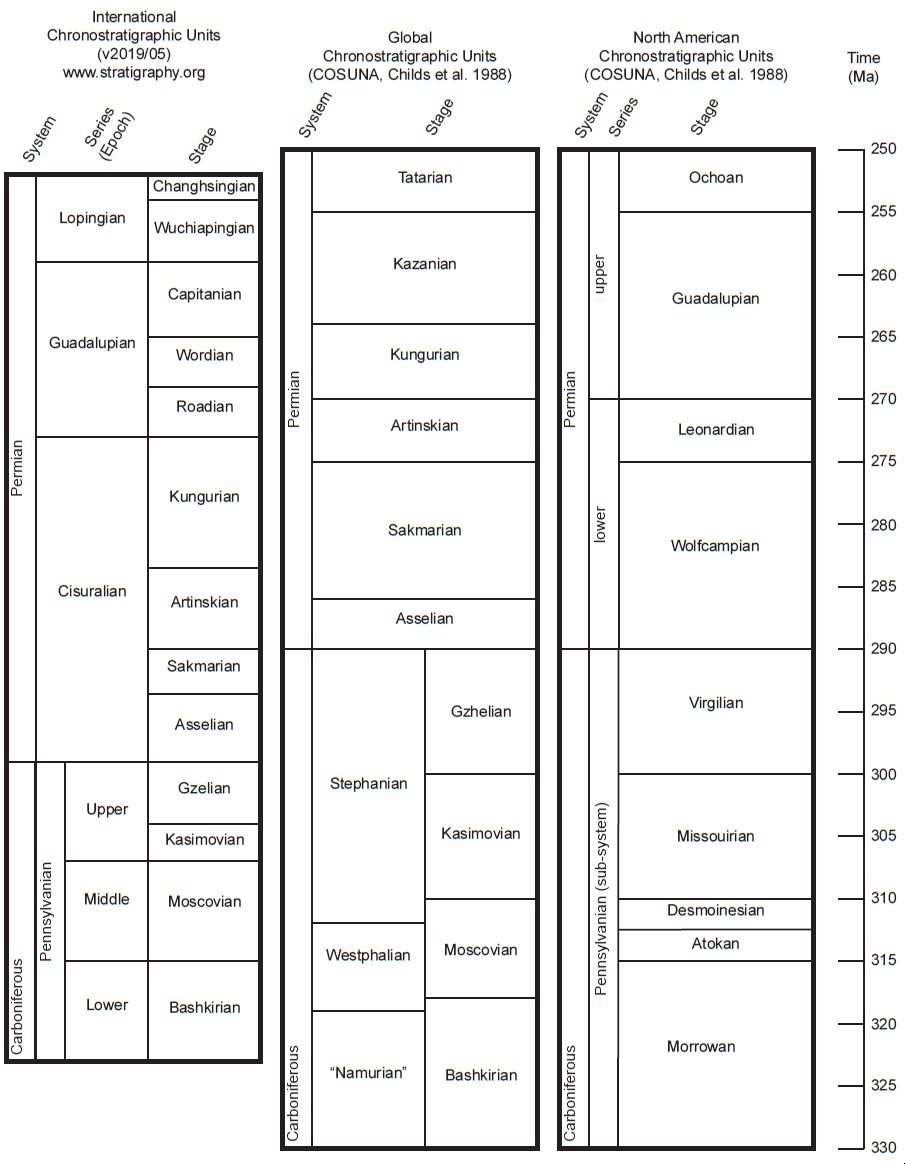
Appendix 2
Catalog of selected Upper Pennsylvanian and Lower Permian sandstones of the western United States. Note: some of the formations found above and below the units will vary depending on where they are located within a particular area. Paul A. Garner contributed some of the research and text contained in this table, but any errors are my responsibility.
Sandstone and column numbers in fig. 5, if applicable | Age (stage in NA chron. units) of sandstone | Stratigraphy above and below sandstone and COSUNA chart abbreviation | Location and max. thickness (m) | Primary reference(s) | Description and notes | ||||
---|---|---|---|---|---|---|---|---|---|
Abo Formation 5– 8 | Leonardian Wolfcampian |
SSMC |
NM 305 | Lee and Girty 1909; Melton 1925; Needham and Bates 1943 | The Abo Formation’s name comes from Abo Canyon at the southern end of the Manzano Range in central New Mexico. Parts of the formation consist of coarse-grained sandstones which are dark red to purple in color. Other parts of the formation include some conglomerate and shale beds. The overlying Yeso Formation contains gypsum. It can be correlated with the Cutler Group of Utah and the Sangre de Cristo Formation of New Mexico and Colorado. At the type section in Valencia and Torrance Counties (New Mexico) it consists of about 60% red shale and about 40% sandstone, arkose and conglomerate, which is more or less typical of the formation. The upper contact with the Yeso Formation is gradational, especially in the northern part of New Mexico. To the south, it grades into the Hueco Limestone of Texas. In the Grand Canyon region, it is probably equivalent to the Supai Group. The Abo is unfossiliferous. |
||||
Bingham Mine Formation 41, 43 | Wolfcampian(?) Virgilian Missourian |
|
UT 2,228 | Tooker and Roberts 1970 | The “Bingham Sequence” of north-central Utah consists of a number of thick Upper Paleozoic formations and groups part of which is the Missourian and Virgilian Bingham Mine Formation which is divided into the Clipper Ridge and Markham Peak Members; the type sections of which occur in the Bingham Canyon Quadrangle. The Clipper Ridge Member is composed of interbedded orthoquartzite and calcareous quartzite containing fusulinids, corals and bryozoans. The sand is laminated, medium- to thick-bedded, fine- to medium-grained and locally cross-bedded. The Markham Peak Member is composed of interbedded orthoquartzite, calcareous quartzite, calcareous sandstone and calcareous siltstone. Some thin limestone beds contain fusulinids. The orthoquartzite is buff to light brown in color, medium- to massively-bedded and locally crossbedded. |
||||
Broom Creek Group 55, 57, 58, 60 | Wolfcampian |
|
MT, ND, NE, SD, WY 98 | Condra, Reed, and Scherer 1940; McCauley 1956 | The Broom Creek Group occurs throughout several states and is generally thought to be Wolfcampian in age. It grades into the Cassa Formation (above) in the Black Hills and west central North Dakota. Here, it consists of beds of dolomite, anhydrite, red shale and white to pink fine- to medium-grained sandstone in a bed 1–9 m thick. In North Dakota the Broom Creek has lesser amounts of dolomite and becomes a very fine-grained sandstone in beds 6–24 m thick. Condra et al. (1940) show a number of formations that are equivalent to the Minnelusa as one goes south from the core of the Black Hills uplift. These include (from oldest to youngest) the Fairbank Formation, the Roundtop Group, the Wendover Group, the Broom Creek Group and the Cassa Group. These formations and groups are constrained by the Pahasapa Formation (below, Redwall Limestone equivalent) and the Phosphoria Formation above (Toroweap/Kaibab Limestone equivalent). |
||||
Brushy Canyon Formation 9, 10 | Guadalupian |
|
TX 300 | Harms 1974; Soreghan and Soreghan 2013 | Harms (1974) originally interpreted this formation as a deep-water density current deposit that is found below the Capitan Reef complex in the Guadalupe Mountains of Texas. The formation is a wedge-shaped body that thins from 300 to 0 m in a distance of just 3.5 km. A number of channels are present which is consistent with the deep-water density flow interpretation. It consists of finely laminated siltstone, coarsely laminated to massive sandstone and massive to boulder or cobble conglomerates. The rocks are primarily composed of quartz, feldspar and various carbonate grains. Sandstones comprise about 35% of the deposit. Fossils include fusulinids, brachiopods, bryozoans and crinoids. Zircon studies indicate a source primarily from the Ouachita system. |
||||
Bursum Formation 7, 8 | Wolfcampian |
|
NM 71 | Myers 1972 | The Bursum Formation consists of red and green shale, arkose, arkosic conglomerate, gray limestone and red sandstones. It occurs in the Oscura and Manzano Mountains of New Mexico. It contains fusulinids that give it a Wolfcampian age. |
||||
Casper Formation 23, 24 | Wolfcampian Virgilian Missourian Desmoinesian Atokan Morrowan |
|
WY 212 | Agatston 1954; Hoyt and Chronic 1962; Knight 1929; McKee and Bigarella 1979; Sando and Sandberg 1987; Steidtmann 1974 | The Casper Formation includes all of the strata from the top of the Madison Limestone to the base of the Opeche red shales in east central Wyoming. In southeastern Wyoming, the Casper rests on the Fountain Formation (as does the Lyons Sandstone in Colorado). It consists of festoon cross-bedded sandstones with some interbedded limestones. The sandstones gradually grade upward into the limestone beds. Occasionally some interbedded shales are present. The sandstones are fine- to medium-grained and range from brick-red, orange, pink, brown, yellow and white in color. Knight, who believed the Casper was water-deposited, reported nearly 800 cross-bed dip measurements with an average dip of about 20°. Most beds dipped towards the south. He also reported some folding in the Casper, which may be related to parabolic recumbent folds, but not enough details are given in the paper to tell for sure. The Darwin Sandstone is the lowest member of the Casper Formation and contains fine-grained calcareous cross-bedded sand of almost pure quartz. It varies from subangular to rounded and has “fair” sorting. Fossils include crinoids, gastropods and conodonts indicative of a Pennsylvanian age. Other authors such as Steidtmann (1974) have attributed the trough crossstratification in the Casper as eolian in origin. McKee and Bigarella (1979) argued the well-sorted nature of the sand, contorted bedding, ripple marks and limestones (interdunal) argued for an eolian origin. Nautiloids and fusulinids collected from parts of the Casper suggest a Wolfcampian age for the upper part of the formation. The upper part of the Casper grades into red shales, dolomite and anhydrite beds. It is thought the Casper is correlative with the Cassa and Broom Creek groups to the northeast and the Minnelusa Formation to the north. Parts of it may be slightly older than the Tensleep Formation to the west. The relationship with the Lyons Sandstone to the south is uncertain. Both formations overlie the Fountain Formation and both thin as they approach the Colorado-Wyoming border. The Lyons may be correlative with the upper part of the Casper. The Casper contains occasional dolomite beds and other carbonates (Agatston 1954). |
||||
Cassa Group 57, 58 | Wolfcampian |
|
CO, NE, SD, WY 122 | Agatston 1954; Condra, Reed, and Scherer 1940; McCauley 1956 | The Cassa Group is composed of two main bodies of sandstone (the Converse Sands) in Wyoming separated by dolomite and anhydrite. The sand is pink to white, medium- to fine-grained, well-sorted, and subrounded to subangular. The Cassa is thought to correlate with the upper part of the Hartville Formation in Wyoming and Nebraska. In North Dakota it contains salt beds and silty shales. Condra, Reed, and Scherer (1940) placed the Lyons Sandstone and the Owl Canyon Formation within the Cassa Group in Colorado and correlated it across the Colorado-Wyoming border to the north. These beds occur above the Casper and consist of shales, limestones, and sandstones. Thin, Lyons-like beds occasionally occur in the measured sections of Condra, Reed, and Scherer (1940) in Wyoming. At their Broom Creek locality, about 15 m of cross-bedded sandstones occur separated by thin limestone beds. About 8 m of cross-beds occur just below in the Broom Creek Formation. Condra, Reed, and Scherer (1940) show that a number of formations are equivalent to the Minnelusa as one goes south from the core of the Black Hills uplift. These include (from oldest to youngest) the Fairbank Formation, the Roundtop Group, the Wendover Group, the Broom Creek Group and the Cassa Group. These formations and groups are constrained by the Pahasapa Formation (below, Redwall Limestone equivalent) and the Phosphoria Formation (above, Toroweap/Kaibab Limestone equivalent). |
||||
Castle Valley Sandstone | Leonardian |
|
UT 183 | Lawton, Buller, and Parr 2015 | The “Castle Valley Sandstone” is a newly proposed unit by Lawton, Buller, and Parr (2015) that occurs in the Castle Valley area of Utah, about 15 km northeast of Moab, Utah. In the past, it has been considered to be a continuation of the White Rim Sandstone that occurs in Canyonlands National Park and vicinity. Lawton, Buller, and Parr (2015) consider this to be an eolian unit that sits above the fluvia l sandstones and conglomerates of the Cutler Formation. Thin sections of the sandstone show bimodality and a wide variety of minerals including mica and K-feldspar. Some of the sand grains of the formation were derived from the nearby Uncompahgre Uplift (including mica), but these authors believe a significant portion of the zircons had eastern Laurentia and Baltica sources. They propose a significant portion of the sand was transported to western Laurentia to make this sandstone by transcontinental river systems. |
||||
Cedar Hills Sandstone 15–18 | Guadalupian Leonardian |
SSMC |
CO, NE, KS 91 | Macfarlane et al. 1993; Moore et al. 1951 | The Cedar Hills Sandstone is a red feldspathic sandstone, siltstone, and silty shale containing beds of white sandstone in the upper and lower parts. The upper sandstone contains gypsum. It occurs over most of the southwestern quarter of Kansas and is a main aquifer. Surface outcrops can be found in Barber and Harper Counties. |
||||
Cedar Mesa Sandstone 26, 27 | Leonardian Wolfcampian |
|
UT 427 | Baars 1979; Condon 1997; Loope 1984; Mack 1977; Mountney and Jagger 2004; Ohlen and McIntyre 1965; Roberts et al. 1965 | The Cedar Mesa Sandstone of southeastern Utah consists of a variety of facies including cross-bedded sandstones, redbeds and mudstones. Baars (1979) and also Mack (1977) believed much of the sandstone was marine based on type and orientation of cross-strata, marine fossils and ripples. There are places in Cataract Canyon and in Mexican Hat where white cross-bedded Cedar Mesa lithologies quickly grade into gypsiferous pink mudstones. In Grand Canyon, it probably correlates with the Esplanade Sandstone. Mountney and Jagger (2004) believed that the Cedar Mesa was primarily eolian based on cross-bed spatial variation and architecture. They believed it was deposited in a wet eolian system with a fluctuating water table and occasional fluvial flooding. They give considerable data on cross-bed dips, many averaging about 20°. Ohlen and McIntye (1965) believe the Cedar Mesa grades into the Cutler Group towards Colorado. Roberts et al. (1965) indicate it grades into the Oquirrh beds to the north. |
||||
Cherry Canyon Formation 9, 10 | Guadalupian |
|
TX 391 | King 1948; Soreghan and Soreghan 2013 | The Cherry Canyon Formation of Texas consists of marine brown shales with some limestones and some sandstones. Most of the sandstone beds are quite thin and some are laminated and can be traced for long distances. Based on detrital zircon studies, Soreghan and Soreghan (2013) believe the source for the Cherry Canyon was from the Ouachita system and several terranes that are now in Mexico and Central America. |
||||
Coconino Sandstone 1–3, 25, 26, 35 | Leonardian |
GB SSMC |
AZ, CA, NV, UT 300 | Baars 1961, 1962; Baltz 1982; Beard et al. 2007; Billingsley and Workman 2000; Blakey 1988, 1990; Blakey, Peterson, and Kocurek 1988; Blakey and Knepp 1989; Brand and Tang 1991; Castor et al. 2000; Doelling et al. 2003; Hintze 1986, 1988; Luepke 1971; McKee 1934; Middleton. Elliott, and Morales 2003; Miller and McKee 1971; Whitmore and Garner 2018; Whitmore et al. 2014; Whitmore, Forsythe, and Garner 2015 | The Coconino is best known as a tan cross-bedded sandstone in central and northern Arizona with excellent outcrops in the Sedona and Grand Canyon areas, first seriously studied by Edwin McKee in 1934. Whitmore et al. (2014) report that it is a subrounded to subangular, fine-grained quartz sandstone that is poorly- to moderately-sorted. It contains occasional dolomite beds, but more widespread dolomite clasts, ooids, cement and rhombs. It contains virtually no fossils except for vertebrate tracks (Brand and Tang 1991) and occasional invertebrate trails. It is usually overlain by the gypsum-bearing Toroweap Formation. Its greatest thickness is in the Pine area where it approaches 300 m. It thins and disappears at the Utah border to the north. It is about 100 m thick in the Grand Canyon area. Baltz (1982) reports 27–177 m-thick beds in the Arica mountains of California. At Frenchman Mountain in Las Vegas, Castor et al. (2000) report a thickness of 5–70 m. Billingsley and Workman (2000) suggest that the Coconino laterally interfingers with the Toroweap Formation in Virgin Mountains and in the Virgin River Gorge area. Hintze (1986) reports up to 615 m of a sandstone with small-scale cross-bedding below Kaibab and Toroweap Formations in the Beaver Dam Mountains of Utah, the upper part of which is perhaps the Coconino; the rest is the Queantoweap Sandstone. Miller and McKee (1971) report about 150 m of Coconino in the Plomosa Mountains of southwestern Arizona (it is metamorphosed). The Scherrer Formation of southeastern Arizona and southwestern New Mexico is a series of cross-bedded marine sandstones and dolomite beds with similar lithologies to the Coconino, about 200 m thick in the type section area, according to Luepke (1971). It extends into Mexico (range unknown, but probably not great). It is thought to be a Coconino equivalent (Blakey 1990). The Coconino extends into extreme southern Utah as mostly planar-bedded sandstones and dolomite beds in the Kaibab/Buckskin Gulch area. Doelling et al. (2003) recognized about 20 m of Coconino here. Further north into Utah, the Coconino may be partially correlative with the White Rim Sandstone, both of which intertongue extensively with the Toroweap Formation according to Blakey (1988). The De Chelly Sandstone caps many of the famous landmarks in Monument Valley where it reaches a maximum thickness of 240 m. It may be slightly older than the Coconino and probably correlates with the Schnebly Hill Formation of the Sedona and Holbrook areas, which underlies the main Coconino body. Blakey and Knepp (1989) show a transitional contact at the base of the Coconino with the Schnebly Hill Formation. Both formations (De Chelly and Schnebly Hill) have similarities to the Coconino in outcrop and thin section. The Glorieta Sandstone of New Mexico is a direct correlative of the Coconino (Baars 1961, 1962) where its maximum thickness is about 100 m. It is mostly a planar-bedded sandstone, but has similar lithology to the Coconino. The Coconino has classically been interpreted as an eolian deposit (McKee 1934) but Brand and Tang (1991) and also Whitmore, Forsythe, and Garner (2015) and Whitmore and Garner (2018) have presented compelling evidence of subaqueous origin. |
||||
Cutler Formation 26 | Leonardian Wolfcampian |
|
AZ, CO, NM, UT 1,800 | Baars 1962, 1979; Condon 1997; Kamola and Chan 1988; Lawton, Buller, and Parr 2015; Melton 1925; Steele 1987 | The Cutler Formation was named for red shales, siltstones, sandstones and arkoses that overlie the marine Pennsylvanian beds near Ouray, Colorado. Some of the arkosic beds reach thicknesses exceeding 1,800 m. In southeast Utah, the Cutler is divided into five formations (oldest to youngest): Halgaito Shale, Cedar Mesa Sandstone, Organ Rock Shale, De Chelly Sandstone, and the Hoskinnini Member. Both the Cedar Mesa and De Chelly Sandstones have large sweeping cross-beds. In the San Rafael Swell area of Utah, the Cutler beds correlate with the Halgaito Formation, Elephant Canyon Formation, Cedar Mesa Sandstone, Organ Rock Shale and the White Rim Sandstone according to Baars (1962). The White Rim Sandstone also has large cross-beds and is quite similar to the Coconino in other ways. The Cutler Formation in Capitol Reef National Park (White Rim Sandstone) is considered to be eolian by Kamola and Chan (1988). They report that it intertongues with the Kaibab Formation above. According to Baars (1979) most of the Cutler Formation grades into and interfingers with marine sediments toward Nevada and New Mexico; the Permian system of the Perm basin in Russia does something similar, also according to Baars. The Cutler beds are likely equivalent to the Yeso and the Abo beds of New Mexico. More recently (Lawton, Buller, and Parr 2015) report the arkosic parts of the Cutler have been identified as fluvial and originating from the Uncompahgre Uplift as interpreted from zircon ages in the formation. |
||||
De Chelly Sandstone 26, 27 | Leonardian |
|
AZ, CO, UT, NM 230 | Baars 1979; Blakey 1990; Condon 1997; Lessentine 1965; Ohlen and McIntyre 1965; Stanesco 1991 | The type section for the De Chelly Sandstone is Canyon De Chelly east of Chinle, Arizona. It has a maximum thickness of about 230 m. The De Chelly caps the “monuments” in Monument Valley along the Arizona-Utah border. Like the Coconino, it contains large cross-beds, very fine to medium sand that ranges from being rounded to subangular. It overlies the Organ Rock Shale, which is correlative with the Hermit Formation in the Grand Canyon region. Like the Schnebly Hill Formation, which it likely correlates with in the Sedona, Arizona area, its sand grains are coated with iron oxide giving the formation a reddish-orange color. Deep wells in Cameron, Arizona and correlation efforts by Blakey (1990) show the De Chelly likely underlies but is closely associated with the Coconino and Glorieta Sandstones. However, Ohlen and McIntyre (1965) thought that both the White Rim and Coconino were equivalents of the De Chelly. Most recently, Stanesco (1991) reported a tongue of the Supai Formation that separated the lower and upper parts of the De Chelly. |
||||
Diamond Creek Sandstone 41–43 | Leonardian |
|
UT 701 | Baker, Huddle, and McKinney 1949; Bissell 1962; Hintze 1988; Meibos 1981; Nielson 1994, 1999; Roberts et al. 1965 | Roberts et al. (1965) show the Diamond Creek Sandstone sitting above the Oquirrh Group, which they claim, is equivalent to the Cedar Mesa Sandstone and Elephant Canyon Formation in southeastern Utah. Nielson (1994) identifies the top of the Diamond Creek Sandstone as resting conformably below the Park City Group, which is a Toroweap Formation equivalent. Nielson (1994, 1999) has the most complete description of the Diamond Creek and he made the report from central Utah where the Diamond Creek consists of three stratigraphic units and rests conformably on the laminated limestones and intraformational breccias of the Kirkman Formation. According to Nielson, all three of the units are marine shelf deposits. The lowest unit in the Diamond Creek is a thin-bedded sandstone to siltstone that contains poorly developed cross-beds. The middle unit contains cyclical units consisting of several sandstone bedding styles including cross-beds, homogeneous sandstones, bimodal sandstones, thinly laminated sandstones and flaserbedded sandstones. The uppermost unit contains fine-bedded sandstones and siltstones. Nielson (1999) identifies the Diamond Creek Sandstone as equivalent to the Weber Sandstone. Baker, Huddle, and McKinney (1949) tentatively correlate the Diamond Creek with the Coconino Sandstone. Hintze (1988) reports a thickness of the Diamond Creek Sandstone of 701 m in the southern Oquirrh Mountains, which is also known as the Hudspeth Cutoff and Oquirrh Formations in this area. Bissel l (1962) reports dolomite in the formation. |
||||
Duncan Sandstone 14 | Guadalupian |
|
OK 60 | Scott and Ham 1957 | The Duncan Sandstone is a fine-grained crossbedded dolomitic sandstone to siltstone that contains no fossils. The cross-beds are concave upwards, have dips from 8–20° and are 0.3 to 1.5 m thick. Individual laminae pinch out downdip. Northwest is the most common dip direction. The Duncan is interbedded with the overlying Flowerpot Shale and is unconformable with the underlying Hennessey Shale. |
||||
Esplanade Sandstone 25 | Wolfcampian Virgilian Missourian |
|
AZ 100 | Baars 1962, 1979; Blakey 1990, 2003; McKee 1982 | In the Grand Canyon area, the Esplanade Sandstone is the uppermost formation of the Supai Group. It correlates with the Queantoweap Sandstone to the northwest in Utah and Nevada. To the northeast, it is likely equivalent to the Cedar Mesa Sandstone in southern and southeastern Utah. To the west, the Abo Formation is the likely equivalent. McKee (1982) has done the most extensive studies on the Esplanade and he reports it as consisting mostly well-sorted, very fine cross-stratified sandstone. The cross-bedding is planar-tabular and planarwedge type. Most is medium scale with 0.3 to 6 m foresets, although foresets up to 21 m have been found. McKee reported equal numbers of cross-beds with dips of greater than 20° and less than 20°. Dip direction is predominantly to the southeast. Of the hundreds of dips reported, only three (all from the same location) had dips of greater than 30°. McKee suggested an aqueous origin of the Esplanade suggesting it was deposited during a “high stage of the lower flow regime” (p. 236). The Esplanade interfingers with marine dolomites in western Grand Canyon (Baars 1979). |
||||
Fairbank Formation 57–59 | Morrowan |
|
SD 30 | Condra, Reed, and Scherer 1940; MacLachlan and Bieber 1963; McCauley 1956 | The Fairbank Formation is a red calcareous sandstone (with some shale) which in some cases may be a lower tongue of the Fountain Formation or perhaps equivalent to the lower part of the Minnelusa Formation. The fine to medium sand is subangular to subrounded and contains some dolomite. Condra, Reed, and Scherer (1940) show a number of formations that are equivalent to the Minnelusa as one goes south from the core of the Black Hills uplift. These include (from oldest to youngest) the Fairbank Formation, the Roundtop Group, the Wendover Group, the Broom Creek Group and the Cassa Group. These formations and groups are constrained by the Pahasapa Formation (below, Redwall Limestone equivalent) and the Phosphoria Formation (above, Toroweap/ Kaibab Limestone equivalent). The Fairbanks is equivalent to the “Bell Sand” in the southern part of the Powder River Basin according to MacLachlan and Bieber (1963). |
||||
Garber Sandstone | Leonardian |
|
OK 180 | Siemers, Stanley, and Suneson 2000 | Siemers, Stanley, and Suneson (2000) report that the Garber consists of sandstones interbedded with siltstones, minor conglomerates and shales. The type section is near Garber, Oklahoma. The upper member of the Garber, referred to by some as the Hayward Sandstone, is cross-bedded. These authors report that the cross-beds are part of a large river system that flowed from east to west. The formation contains dolomite pebbles, trough cross-bedding, planar bedding, ripple marks and structures interpreted to be desiccation cracks and paleosols. |
||||
Glorieta Sandstone 5-9, 11–13, 19, 20 | Guadalupian Leonardian |
SSMC |
NM, OK, TX 90 | Baars 1974; Blakey 1990; Brill 1952; Dinterman 2001; Irwin and Morton 1969; Krainer and Lucas 2015; Needham and Bates 1943 | The Glorieta is recognized in New Mexico, Texas and Oklahoma. Baars (1974) describes the Glorieta as a fine- to medium-grained quartz sandstone with thin to medium cross-beds with dips of 10 to 20°. Baars thought that most of the Glorieta was aqueously deposited. Dinterman (2001) describes the Glorieta (in NM) as being primarily a well-sorted, fine-grained quartz arenite. According to Blakey (1990) it is probably correlative with the main body of the Coconino in Arizona and Brill (1952) believes it is correlative to the Lyons in Colorado. Irwin and Morton (1969) show correlatives in the Texas Panhandle (Glorieta), the Oklahoma Panhandle (Glorieta) and Kansas (Cedar Hills Sandstone). The Glorieta is interbedded with gypsum and interfingers with the San Andres Formation that also contains gypsum. Dinterman (2001) reports two primary facies within the Glorieta in New Mexico: interbedded and small-scale ripple-laminated sandstone (CRLS) and interbedded symmetrical-rippled crossbedded and horizontally-laminated sandstone (SCHL). The CRLS facies, interpreted as eolian, is common at the base of the formation and consists of 0.5 to 3 m-thick planar and trough cross-beds. Current direction is to the south and southeast. The ripples are interpreted as climbing wind ripples. The SCHL facies has some cm-thick ripples that are interbedded with small trough and planar cross-beds and horizontal laminae. Most cross-beds dip to the south and southwest. Dinterman suggests longshore currents produced these facies. Krainer and Lucas (2015) still believe some of the facies in this sandstone are eolian, especially when they occur as cross-beds. |
||||
Hudspeth Cutoff Formation 47 | Leonardian Wolfcampian |
|
ID, NV, UT 700 | Cramer 1971; Isaacson, Bachtel, and McFaddan 1983; Roberts et al. 1965; Yancey, Ishibashi, and Bingman 1980 | The Hudspeth Cutoff Sandstone was erected by Cramer
(1971) as having a thickness of 1,067 m. Yancey, Ishibashi,
and Bingman (1980) redefined it as the highest sandstone
of the Oquirrh Group and having a thickness of 700 m and
consisting of mostly non-calcareous sandstone with minor
beds of sandy limestone and chert. The type section is
in Oneida County, Idaho. Because of the nature of how
the sandstone weathers, sedimentary structures are
largely unknown. Roberts et al. (1965) clearly believe the
formation is marine because it contains fusulinids and
brachiopods in thinly bedded limestone units that occur
within the formation. |
||||
Ingleside Formation 21, 22 | Wolfcampian |
|
CO 100 | Hoyt 1961; Lee 1927; Maughan and Wilson 1960; Maughan and Ahlbrandt 1985; McKee and Oriel 1967; Pike and Sweet 2018 | Lee (1927) describes the Ingleside Formation as consisting of a lower sandstone, a middle limestone/ sandstone and an upper sandstone unit; together they are about 100 m thick. He suggests the beds are equivalent, at least in part, to the Lyons Sandstone of northern Colorado and the Casper Sandstone of southeastern Wyoming. McKee, and Oriel (1967, section 22) show the Ingleside as stratigraphically below the Lyons and Satanka Shale in eastern Colorado and the Ingleside beds may be equivalent to the upper part of the Fountain Formation of north-central Colorado. Pike and Sweet (2018) identify the Ingleside as consisting of eolian deposits in the Manitou Springs area of Colorado which rest conformably on the Fountain Formation. Previous workers have identified these same beds as the Lyons Formation. Maughan and Wilson (1960) describe the Ingleside as consisting of fine-grained sandstone of various colors and carbonate rock. The sandstones have larger grains of rounded quartz, and smaller subangular grains. Bedding is variable and consists of horizontal laminae, medium- to large-scale cross-beds with both high and low angles of stratification. Both limestone and dolomite are present in the carbonate beds. It unconformably overlies the Fountain Formation. |
||||
Juniper Gulch Member and Gallagher Peak Sandstone of Snaky Canyon Formation 50 | Leonardian Wolfcampian Virgilian Missourian |
|
ID 59/597 | Isaacson, Bachtel, and McFaddan 1983; Skipp et al. 1979 | Skipp et al. (1979) report that the Gallagher Peak Sandstone Member of the Snaky Canyon Formation is a medium-gray to light-brownish-gray, very fine-grained, thin-bedded quartzose sandstone with calcareous cement. The sandstone may not be laterally extensive and may consist of a series of lenses. It is fossiliferous containing brachiopods, pelecypods, fusilinids, corals and bryozoans. The Juniper Gulch Member of the Snaky Canyon Formation consists mostly of sandy carbonate rocks with chert nodules and a thickness of 597 m. It is correlative, at least in part, with the Wood River Formation, Quadrant Formation, Wells Formation, Oquirrh Formation and Tussing and Hudspeth Cutoff Formations (Isaacson, Bachtel, and McFaddan 1983). |
||||
Lyons Sandstone 21 | Leonardian |
|
CO 107 | Brill 1952; Hubert 1960; Lee 1927; Maher 1954; Maher and Collins 1953; McKee and Bigarella 1979; Pike and Sweet 2018; Ross et al. 2010; Thompson 1949; Walker and Harms 1972 | The Lyons Sandstone is best known from the Colorado Front Range where it extends into the subsurface of southeastern Colorado, western Kansas, and parts of Wyoming and Nebraska (Maher 1954). The Lyons can be traced into New Mexico and is correlative with the Glorieta Sandstone (Brill 1952) which has been long recognized to correlate with the Coconino Sandstone in Arizona. At most locations, the Lyons has been divided into three units: lower, middle, and upper. At its type locality, in Lyons, Colorado, the formation is about 107 m thick. The Lyons is very similar to the Coconino in many respects (McKee and Bigarella 1979) but authors have disagreed over the years whether the deposit is a shallow marine or coastal dune deposit. Pike and Sweet (2018) identify previously mapped Lyons strata in the Manitou Springs area of Colorado as eolian beds in the Ingleside Formation. |
||||
Minnelusa Formation 32, 53, 54, 56, 59 | Wolfcampian Virgilian Missourian Desmoinesian Atokan Morrowan |
|
MT, SD, WY 250–366 | Condra, Reed, and Scherer 1940; Fryberger 1984; McCauley 1956; Thompson and Kirby 1940; Verville and Thompson 1963 | The Minnelusa is a red calcareous sandstone, which in some cases may be a lower tongue of the Fountain Formation. Condra, Reed, and Scherer (1940) show a number of formations that are equivalent to the Minnelusa as one goes eastward from the core of the Rocky Mountain uplift. These include (from oldest to youngest) the Fairbank Formation, the Roundtop Group, the Wendover Group, the Broom Creek Group and the Cassa Group. These formations and groups are constrained by the Pahasapa Formation (below, Redwall Limestone equivalent) and the Phosphoria Formation (above, Toroweap/Kaibab Limestone equivalent). Parts of the Minnelusa contain fusulinids (Verville and Thompson 1963). |
||||
Oquirrh Formation 42 | Wolfcampian Virgilian Missourian Desmoinesian Atokan |
|
UT, ID 8,000 | Bissell 1959; Isaacson, Bachtel, and McFaddan 1983; Roberts et al. 1965; Yancey, Ishibashi, and Bingman 1980 | Roberts et al. (1965) described a thick sequence of rocks in northwestern Utah, northeastern Nevada and south-central Idaho, containing carbonates and siliciclastics. The rocks range in age from Upper Pennsylvanian through Permian and include the coarse clastic facies of the Oquirrh Formation. The Oquirrh Formation grades south and southwest into the Riepe Spring (Sandstone or Formation) and Arcturus Formations. To the east, it probably grades into the Weber, but the transition cannot be observed because it is covered with thrust sheets. To the southeast it grades into the Cedar Mesa and Cutler Sandstones. According to Roberts et al. (1965) the Oquirrh is exceptionally thick (up to 8,000 m) and consists of 80% terrigenous sandstone, 5–10% fine-grained limestone and shaly limestone, 5% shale, and 2% nodular and bedded chert. In Idaho, the formation is correlative, at least in part to the Snaky Canyon Formation, the Wells Formation, the Wood River Formation and the Quadrant Formation (Isaacson, Bachtel, and McFaddan 1983). |
||||
Quadrant Sandstone 51, 52 | Desmoinesian |
NRW |
ID, MT 533 | James 1992; Reeves 1931; Saperstone and Ethridge 1984; Thompson and Scott 1941 | Saperstone and Ethridge (1984) describe the Quadrant Sandstone as consisting of a lower marine unit and an upper eolian unit. The sand reaches thicknesses exceeding 530 m in the southwest corner of Montana according to their isopach map. It is equivalent to the Quadrant Formation and Devil’s Pocket Formation in central Montana and the Tensleep Sandstone to the south, in Wyoming. The lower marine unit consists of bioturbated sandstones and dolostones, some of which are cross-bedded. According to the authors, the upper unit consists of several sand facies including small- and large-scale cross-beds, massive sands and horizontally laminated sandstone. The authors note that cross-bed dips are predominantly to the south, the same as other correlative sandstones. James (1992) studied 138 thin sections from the Quadrant. Nine different cements were found, the most significant of which were anhydrite, quartz, dolomite, and calcite. The sand was reported as well-sorted and compositionally mature. Carbonate beds within the sandstone were present as abundant dolostone beds, common calcareous dolostone, and rare limestone beds. Carbonate beds were centimeters to meters in thickness. Fossils recognized in the carbonate beds include echinoderms, fusulinids, bivalves, brachiopods, and corals. The type section of the Quadrant contains fusulinids (Thompson and Scott 1941). |
||||
Queantoweap Formation 35–39 | Wolfcampian |
|
UT, NV 610 | Bissell 1962; Hintze 1986; McNair 1951 | The Queantoweap outcrops in the Virgin River Gorge (extreme northwestern Arizona) and then extends northward into western Utah and southeastern Nevada. In western Utah, the Queantoweap is equivalent to the Cedar Mesa and Talisman Quartzite. Hintze (1986) reports thicknesses of 457–610 m in the Beaverdam Mountains of southwestern Utah. It was named by McNair (1951) for exposures of cross-bedded sandstone that occur below the Hermit Formation in Queantoweap Canyon (Whitmore Canyon) in northern Arizona. Here, the Queantoweap is equivalent to exposures of the Esplanade Sandstone further to the east at the top of the Supai Group. Hintze reports that no body fossils have been found in the Queantoweap, but that it is locally intensely bioturbated. McNair (1951) reports that the Queantoweap has a transitional contact with the Hermit Formation ranging in a zone from 2–16 m. |
||||
Rib Hill Sandstone | Wolfcampian |
|
NV 305 | Bissell 1964a; Langenheim and Larson 1973; Steele 1960 | The name “Rib Hill Sandstone” shows up on some stratigraphic charts for central Nevada, but according to Bissell (1964b) and Steele (1960) it should be abandoned in favor of the Riepetown Sandstone (described below). Bissell (1964a) suggested the name Riepetown Formation because sandstone is not the dominant lithology at the Rib Hill type section. |
||||
Riepetown Formation 40, 62–64 | Wolfcampian |
|
NV, UT 366 | Bissell 1962, 1964a; Steele 1960 | Steele (1960) suggested the “Rib Hill Sandstone” should be formally called the “Riepetown Sandstone” and Bissell (1964a) suggested it should be called the “Riepetown Formation” because sandstone is not the dominant lithology at the Rib Hill type section. Here, according to Steele (1960, 103), it is a yellowish-gray, very fine- to medium-grained, rounded to subrounded, and a thin- to thick-bedded sandstone. It contains some limestones. Steele claims this is the most widespread Permian sandstone of the area, covering approximately 65,000 km2. Bissell (1964a) describes the sandstone as consisting of a variety of lithologies and cements. It includes non-calcareous, calcareous and dolomitic cements, fine-grained sand and medium to thick bedded sandstones. |
||||
Rush Springs Sandstone | Guadalupian |
|
OK 25–125 | Kocurek and Kirkland 1998; Poland and Simms 2012 | Poland and Simms (2012) interpret the Rush Springs as an ancient erg to erg margin sandstone with wind directions toward the west and southwest, generally consistent with paleocurrent directions of other sandstones on the Colorado Plateau. Kocurek and Kirkland (1998) report that the formation contains thick (up to 3 m) sets of steeply dipping trough cross-beds and massive beds, with good sorting, frosted grains, absence of marine fossils, wind-ripple strata and grainflow strata. |
||||
Sangre de Cristo Formation 19, 20 | Wolfcampian Virgilian MissourianDesmoinesian |
|
NM 2,900 | Baltz 1965; Bolyard 1959; Melton 1925; Read and Wood 1947 | The Sangre de Cristo Formation outcrops in northern New Mexico and is correlative to the Yeso Formation (Read and Wood 1947). It consists of red and green shales that are interbedded with arkoses, conglomerates and marine limestones (Baltz 1965). It likely continues into Colorado where it is correlative of similar rocks representing the Fountain Formation. Both the Sangre de Cristo and the Fountain Formation consist of sandstones and arkoses that were shed from various orogenic events in the area of the Rocky Mountains. In places, both formations rest on Precambrian basement rock. |
||||
Scherrer Formation 4 | Leonardian |
|
AZ, NM 210 | Blakey and Knepp 1989; Butler 1971; Gilluly, Cooper, and Williams 1954; Luepke 1971; Zeller 1965 | The Scherrer Formation received its name for the type section on Scherrer Ridge, Cochise County, Arizona (Gilluly, Cooper, and Williams 1954). Luepke (1971) described four informal members of the Scherrer: basal red beds, a lower sandstone member, a middle carbonate member and an upper sandstone member. Most of these strata are considered water-deposited, although an eolian origin has been suggested for the lower sandstone member. This sandstone is pale-colored and consists of subangular to rounded, moderately- to well-sorted, very fine- to fine-grained quartz sand. Zeller (1965) describes it as a medium- to fine-grained subrounded quartz sand in a limestone matrix. However, no obvious pitting or frosting of grains is observed (Luepke 1971, 250) and the presence of two dolomite horizons may indicate a littoral or shallow marine environment. Luepke (1971, 250) proposed that these facies might represent beach deposits or coastal dunes that had undergone marine reworking or shallow marine sands that had been reworked on a beach. Blakey and Knepp (1989) identify the formation as Leonardian and correlative with the Coconino, found further to the north. They are more uncertain about the depositional environment of the Scherrer. |
||||
Schnebly Hill Formation 3 | Leonardian |
|
AZ 600 | Blakey (1984); Blakey and Knepp (1989); Blakey and Middleton (1983) | The Schnebly Hill’s type section is in the Sedona area and it is correlative with the De Chelly Sandstone and grades into the Yeso Formation of New Mexico (Blakey and Knepp 1989). It intertongues with the Coconino Sandstone in the Sedona area and it reaches thicknesses of up to 600 m in the Holbrook Basin (Blakey and Knepp 1989). Based on sedimentary structures, Blakey and Middleton (1983) identified the Schnebly Hill as having various marine, coastal dune and inland dune facies. It has cross-bed foresets up to 15 m thick. |
||||
Shattuck Sandstone | Guadalupian |
|
TX 30 | Mazzullo, Malicse, and Siegel 1991 | Mazzullo, Malicse, and Siegel (1991) report that the Shattuck Sandstone represents a shelf facies in the Permian Basin of Texas and it is equivalent to the Goat Seep Formation (interpreted as a reef on the basin margin) and the Cherry Canyon Formation (interpreted as a basin sandstone in the Permian Basin). The Shattuck is a member of the Queen Formation of the Artesia Group. Like many other units in the Permian, the Shattuck is bounded by a variety of carbonates and evaporite minerals. These authors believe it was deposited in a semi-arid coastal plain environment. They report non-fossiliferous, well-sorted and cross-bedded sandstones in what they believe are eolian dunes. |
||||
Shedhorn Formation (Sandstone) 51, 52 | Guadalupian |
|
MT, WY 36 | McKelvey et al. (1959) | McKelvey et al. (1959) identify the Shedhorn Sandstone as “Phosphoria” in age and occurring in the vicinity of Yellowstone National Park as upper and lower members. Sandwiched between the two members are beds of chert, mudstone, phosphorite, and carbonate rock of the Phosphoria Formation. The sandstones are fine- to coarse-grained and contain silicified fossils, some thin limestone beds, glauconite, chert nodules, and chert beds. |
||||
Talisman Quartzite 37 | Wolfcampian |
|
UT 369 | Baetcke 1969; Whelan 1982 | The Talisman outcrops in Beaver County near the Talisman Mine in the Star Range, Utah, and has been separated into a lower and upper unit. The lower unit consists mostly of limestone with fine- to coarse-grained sandstone beds. The upper unit consists mostly of cross-bedded sandstone. The lower part of the Talisman is considered to be correlative with the Queantoweap (Esplanade) Sandstone of northern Arizona and the Cedar Mesa Sandstone of southern Utah (Whelan 1982). Baetcke (1969) agrees with the previous interpretation that the lower unit is equivalent to the Queantoweap. He hesitates assigning the cross-bedded upper unit to the Coconino because previous writers have only assigned “eolian” units to the Coconino (p. 44), although he agrees these beds “may” be equivalent to the Coconino. His hesitation has to do with his recognition that the cross-beds of the Talisman are marine sands with “herringbone structures.” (p. 45) |
||||
Tensleep Sandstone 29, 30, 33, 34 | Wolfcampian Virgilian Missourian Desmoinesian Atokan |
NRW |
MT, WY 25–305 | Agatston 1952, 1954; Andrews and Higgins 1984; Curry 1984; Darton 1904; Hoare and Burgess 1960; Kerr and Dott 1988; Kerr et al. 1986; Lane 1973; Link et al. 2014; Mankiewicz and Steidtmann 1979; Sando, Gordon, and Dutro 1975; Verille, Sanderson, and Rea 1970 | The Tensleep received its name from exposures in
the lower canyon of Tensleep Creek in Washakie
County, Wyoming (Darton 1904). It has a gradational
lower contact with mudstones and carbonates of the
Pennsylvanian Amsden Formation (Kerr et al. 1986)
and is overlain unconformably by the Permian Goose
Egg and Park City Formations (Kerr et al. 1986).
The members of the Goose Egg Formation onlap
onto a dendritic palaeotopography incised into the
top of the Tensleep (Lane 1973; Curry 1984). The
Tensleep Sandstone exceeds 100 m of thickness in
the southern Bighorn Mountains and reaches 170 m in
the extreme southeast. Around Shell Canyon it thins
to about 20 m but then thickens northwards again to
about 80 m (Kerr et al. 1986). Variations in thickness
are thought to be the result of the erosional relief at
the top of the unit (Kerr et al. 1986). |
||||
Toroweap Formation 3, 25, 35–37, 39, 40, 61 | Leonardian |
GB SSMC |
AZ, UT, NV 100–300 | Blakey 1990; Rawson and Turner-Peterson 1980 | At Show Low in east central Arizona the Coconino is water-deposited (Peirce, Jones, and Rogers 1977). It is overlain by and intertongues with the marine Toroweap Formation. In fact, the upper Coconino may be regarded as an eastern facies of the Toroweap (Rawson and Turner-Peterson 1980). In the Sycamore Canyon area of Arizona, the Toroweap and Coconino laterally interfinger with one another (Blakey 1990). Rawson and Turner-Peterson (1980) identify four depositional environments of the Toroweap: 1) open marine, 2) restricted marine, 3) sabkha, and 4) eolian dune. According to these authors, the Toroweap was deposited as the sea transgressed and drowned the Coconino eolian dune field. Gypsum deposits are typical in the Toroweap. The Toroweap is extensive, covering parts of Arizona, Utah and Nevada. |
||||
Tubb Sandstone Member of Clear Fork Group and Abo Formation 11–13 | Leonardian |
|
NM, TX 65 | Hartig et al. 2011 | The Tubb Sandstone is a member of the Clear Fork Group of west-central Texas and is equivalent to the Drinkard Sandstone. In New Mexico, it is a member of the Yeso Formation. In both areas, it is stratigraphically below and distinct from the Glorieta Sandstone. Hartig et al. (2011) report numerous fluvial and eolian deposits in the Abo-Tubb interval containing dolomite and paleosols. |
||||
Tussing Formation 47 | Missourian Desmoinesian |
|
ID 1,300 | Cramer 1971; Isaacson, Bachtel, and McFaddan 1983; Yancey, Ishibashi, and Bingman1980 | The Tussing Formation is a mixture of sandy limestones, calcareous sandstones, siltstones and bioclastic limestones (Yancey, Ishibashi, and Bingman 1980). Brachiopods and bryozoans have been used to date the formation. It is correlative, at least in part, with the Snaky Canyon Formation, Quadrant Formation, Wells Formation, Oquirrh Formation and Wood River Formation (Isaacson, Bachtel, and McFaddan 1983). |
||||
Tyler Formation 34, 53–56, 60 | Morrowan |
|
MT 30–60 | Maughan and Roberts 1967 | In the Big Snowy Mountains in central Montana, the Tyler Formation is part of the Amsden Group. East of that location, the Tyler is predominantly sandstone. Across the border, into western North Dakota the Tyler becomes predominantly shale. In central Montana, Maughan and Roberts (1967) divide the Tyler into two members: the lower Stonehouse Canyon Member (25 m) and the upper Cameron Creek Member (88 m). The Cameron Creek member is a series of shales and limestones with a prominent 2 m-thick calcareous sandstone contained within. The Stonehouse Canyon member also has varied lithologies, with a predominance of sandstone. The Tyler is equivalent to parts of the Amsden Formation further south in Wyoming. Maughan and Roberts (1967, B3) show the Quadrant Sandstone stratigraphically above the Tyler. |
||||
Weber Sandstone 28, 29, 44, 45 | Leonardian Wolfcampian Virgilian Missourian Desmoinesian Atokan |
GB |
UT 100–700 | Bissell 1964a, 1964b; Bissell and Childs 1958; Chure et al. 2014; Doe and Dott 1980; Donnell 1958; Driese 1985; Fryberger 1979; Koelmel 1986; Link et al. 2014; Melton 1925; Roberts et al. 1965 | According to Fryberger (1979) the Weber has multiple evidences for the eolian origin of its beds including large-scale cross-beds, raindrop imprints, contorted stratification and well-sorted quartz sandstones (with interbedded fluvial deposits). However, he does recognize that parts of the Weber further to the west are marine. He reported that the Weber is correlative with the Tensleep Sandstone of Wyoming and the Wells Formation of northeastern Utah. The Weber Sandstone is a subangular to subrounded, fine-grained, crossbedded quartz sandstone of Pennsylvanian to Permian age (Koelmel 1986). Chure et al. (2014) report vertebrate tracks and invertebrate traces from the formation. Overlying the Weber Sandstone are marine carbonates, sandstones and phosphatic shales of the Permian Park City Formation. Below the Weber is the Lower Pennsylvanian Morgan Formation, also of marine origin. There are apparently transitional contacts between the Weber Sandstone and the formations above and below it in the vicinity of Weber Canyon (Bissell and Childs 1958, 28). Thin fusulinid-bearing carbonates are interbedded with the sandstone in the lowermost and uppermost Weber (Bissell 1964a). In eastern Utah, the transitional contact with the overlying Park City Formation persists but the junction with the underlying Morgan Formation is abrupt (Bissell and Childs 1958, 28). East of Rangely both contacts are again transitional (Bissell and Childs 1958, 28). Further to the east the Weber displays a transitional upper contact with the Phosphoria Formation in Utah and the State Bridge Formation in Colorado (Bissell and Childs 1958, 28). Laterally the Weber interfingers with the shales, siltstones and arkoses of the Maroon Formation in Colorado (Bissell and Childs 1958, 28; Donnell 1958, 97). Roberts et al. (1965, 1932) says that Oquirrh probably grades eastward into Weber. Thin, laterally discontinuous, unfossiliferous dolomites occur within the eastern part of the Weber outcrop in northern Utah and Colorado (Driese 1985). They are found exclusively along first-order bounding surfaces separating 1–30 m-thick sets of cross-bedded sandstone. The dolomite beds consist predominantly of euhedral to subhedral dolomite rhombs with about 10–50% sand and silt particles. In thin section they closely resemble the dolomitized carbonates of the underlying Morgan Formation (Driese 1985,187). Several features of the dolomite beds are suggestive of shallow to emergent conditions. These include wrinkled laminae of possible algal origin, calcite-filled vugs interpreted as nodular anhydrite pseudomorphs and sand-filled cracks interpreted as desiccation features. Driese (1985) proposed that the dolomites represent interdunal pond deposits similar to the Pleistocene-Holocene non-marine carbonates of the North African Sahara, although he did express some bewilderment as to why so few organisms appear to have been present in the Weber interdunes compared with modern interdune environments. Link et al. (2014) have found detrital zircons in the Weber that suggest one of its primary sources of sand was from the Appalachians. |
||||
Wells Formation 46 | Wolfcampian Virgilian Missourian Desmoinesian Atokan |
CSR |
ID, MT, UT, WY 732 | Richards and Mansfield 1912; Yancey, Ishibashi, and Bingman 1980 | Richards and Mansfield (1912) report the Wells Formation as consisting of sandy limestones, calcareous limestones and other sands of “variable character.” The type section is in Wells Canyon, Idaho, which is a 732 m section containing marine fossils. The formation is recognized in the vicinity of where the states Utah, Idaho, Montana and Wyoming come together. Yancey, Ishibashi, and Bingman (1980, 267) state that the upper member of the Wells Formation is quite similar to part of the Hudspeth Cutoff Formation. |
||||
White Rim Sandstone 27, 38 | Leonardian |
GB |
UT 80 | Baars 1979; Baars and Seager 1970; Baars 2010; Blakey, Peterson, and Kocurek 1988; Chan 1989; Ohlen and McIntyre 1965; Steele 1987; Steele-Mallory 1982; Tubbs 1989 | The best exposures of the White Rim Sandstone occur
in the vicinity of Canyonlands National Park, Utah where
it forms a “white rim” around much of the Colorado
and Green River canyons. The sandstone probably
correlates with the upper portion of the Coconino (Blakey
Peterson, and Kocurek 1988). Its greatest thickness
is about 80 m (Chan 1989). Baars and Seager (1970)
thought that the sandstone represented a nearshore
shallow marine bar, a view which Baars still held in 2010.
But, Tubbs (1989) and most others now identify the
White Rim as a coastal dune deposit based on windripple
strata, sand-flow toes, raindrop imprints, planar
bounding surfaces, eolian textural trends, high percentage
quartzose composition, lack of clay and silt in the deposit
and deformational features. Steele-Mallory (1982) reports
glauconite (a marine mineral) and a crinoid fragment from
the formation. |
||||
Wood River Formation 48, 49 | Guadalupian Wolfcampian Virgilian Missourian Desmoinesian |
|
ID 2983 | Isaacson, Bachtel, and McFaddan 1983; Link et al. 2014; Skipp and Brandt 2012; Skipp et al. 1979 | The marine Wood River Formation is likely named from exposures near the Wood River in Blaine County, Idaho. It also occurs in the Fish Creek and Bay Horse areas. It is correlative, at least in part, with the Snaky Canyon Formation, Quadrant Formation, Wells Formation, Oquirrh Formation and Tussing and Hudspeth Cutoff Formations (Isaacson, Bachtel, and McFaddan 1983). In the Fish Creek Reservoir area, it consists of siliceous sandstone, calcareous sandstone, sandy limestone and minor conglomerate and siltstone (Skipp and Brandt 2012). Link et al. (2014) conclude based on zircon studies that most of the sand grains in the Wood River and Tensleep Formations were derived from a “continental-scale system” ultimately derived from the Grenville Province of eastern North America. |
||||
Yeso Formation 5–8, 19, 20 | Leonardian |
SSMC |
NM 300–600 | Baars 1979; Dinterman 2001; Needham and Bates 1943 | Needham and Bates (1943) redescribed the type section of the Yeso, which occurs near Socorro, New Mexico. It occurs directly below the Glorieta Sandstone and has a gradational contact with it. The Yeso consists of 300–600 m of sandstone, shale, dolomites, limestone and gypsum. The sandstone varies in color (gray, pink, yellow, red, purple) and is coarse- to fine-grained in texture. It often contains gypsum beds up to 60 m in thickness. Baars (1979) believes the Meseta Blanca Sandstone Member of the Yeso Formation is equivalent to the De Chelly of eastern Arizona. Baars suggests that it is also equivalent to the Schnebly Hill Formation in the Sedona area of Arizona. Baars (1979) believes the Fort Apache Limestone tongue within the Schnebly Hill Formation is an extension of the Yeso dolomite-gypsum facies into Arizona. In describing the basal member of Yeso (Meseta Blanca), Dinterman (2001, 108) noted cross-bedded sandstone (interpreted as eolian) in the northeastern part of New Mexico in which he found muscovite. |
Appendix 3
Details and references for selected Permian sandstones occurring in Canada, Europe, South America, and Saudi Arabia. Paul A. Garner contributed some of the research and text contained in this table, especially for some of the UK sandstones, but any errors are my responsibility. Some material was found in Rodríguez-López et al. 2014, Supplementary Table S2 (Compilation of main Cambrian, Ordovician, Silurian, Devonian, Carboniferous, and Permian eolian sand systems). Some material comes from the table in Whitmore and Strom’s 2018 ICC paper.
Sandstone | Conventional age | Location and maximum thickness, if known (m) | Primary reference(s) | Description and notes |
---|---|---|---|---|
Andapaico Formation | Permian | Argentina, 5 | Correa, Carrevedo, and Gutiérrez 2012 | Correa, Carrevedo, and Gutiérrez (2012) identify a variety of facies in this 430 m-thick package of siliciclastic sediments including eolian, beach, river, shoreface, barrier island and lagoon. There are two eolian sequences, each about 5 m thick. |
Bridgnorth Sandstone | Lower Permian | England, 180 | Karpeta 1990; Mader and Yardly 1985; Shotton 1937, 1956 | The Bridgnorth was formerly known as the “Lower Bunter Sandstone.” The unit displays large-scale cross-bedding and is considered to represent a large erg with complex dune types. Karpeta (1990) found average dips of 26° in the Bridgnorth area. A variety of different deposits were identified including grainfall, grainflow and ripple laminae. Karpeta states (1990, 61): “Soft-sediment deformation structures are quite common and include stretched out laminae, fade outs, overturned folds, thrusts, pull-aparts and rare sand breccias. In general, compressive structures predominate over tensional. It is estimated that this stratification type makes up 70% (by thickness) of the Bridgnorth Sandstone Formation.” |
Brumund Formation | Permian | Norway, 800 | Larsen et al. 2008 | The Brumund Formation which is a northern extension of the Rotliegendes sands of Europe is found in the Oslo Rift. Larsen et al. (2008) report that the formation has yellow, large-scale cross-stratified beds that are interpreted as eolian deposits. However, other facies are present as well such as fluvial, lacustrine and floodplain deposits. |
Champenay Formation | Upper Permian | France, 110 | Swezey, Deynoux, and Jeannette 1996 | Swezey, Deynoux, and Jeannette (1996) report that the Champenay consists of a variety of lithologies including breccias, sandstones, conglomerates and mudstones. Many of the sands are poorly sorted and contain mica. The authors believe the sandstones in this formation represent a variety of environments including deltaic, lacustrine margin, alluvial and eolian. The eolian sands were identified because the authors believed some of the sediments resembled eolian ripples based on coarsening-upwards laminae and facies relationships with the other sediments in the outcrops. |
Corncockle Sandstone | Lower Permian | Scotland, 1000 | Brookfield 1977, 1978; McKeever 1991 | The Corncockle Sandstone is thought to have been deposited as dome-shaped and barchanoid dunes. Vertebrate tracks were reported from this formation by McKeever (1991). |
Corrie Sandstone | Lower Permian | Scotland, 700 | Clemmensen and Abrahamsen 1983; Gregory 1915; Piper 1970 | The Lower Permian Corrie Sandstone of the Isle of Arran in southwestern Scotland is at least 700 m thick (Clemmensen and Abrahamsen 1983). Piper (1970) described the sandstones in the type section at Corrie, Scotland as medium-grained, very well-sorted, rounded and with frosted grains. The Corrie Sandstone has long been regarded as eolian in origin (Gregory 1915) and more recent workers have agreed with this assessment. Clemmensen and Abrahamsen (1983) proposed that the sandstone was deposited as part of a small erg system bounded to the northwest by alluvial fans. Borsch et al. (2018) found mica in their thin sections of this sandstone. |
Dawlish Sandstone | Upper Permian | England, 120 | Newell 2001 | The Dawlish Sandstone is interpreted to be a mix of eolian and fluvial deposits; the eolian facies consisting of well-sorted, fine- to medium-grained sand with small to medium cross-bed sets of 1–5 m. Newelll (2001) identified grainfall and grainflow deposits up to 30 mm thick with foreset dips up to 35°. The sandstone also contains low-angle sand sheets up to 2 m thick which are also attributed to eolian processes. |
De la Cuesta Formation | Permian | Argentina, 1600 | Limarino and Spalletti 1986; Spalletti, Limarino, and Piñol 2010 | Limarino and Spalletti (1986) and Spalletti, Limarino, and Piñol (2010) report the De la Cuesta Formation as a fine to very fine-grained sandstone with crossbed dips averaging between 20–24°. Some ripple marks were observed on lower-angle cross-beds. The formation contains a variety of lithologies including mudstone, fine sandstone and evaporite facies. They report laminae formed from grainflow, grainfall and ripple migration. Both tangential [tabular?] and planar cross-beds are present. |
Hopeman Sandstone | Permian | Scotland, 60 | Maithel, Garner, and Whitmore 2015; Ogilvie, Glennie, and Hopkins 2000; Peacock 1966; Peacock et al. 1968 | Borehole data suggest a maximum thickness of 60 m for this sandstone (Ogilvie, Glennie, and Hopkins 2000). The formation is characterized by large-scale cross-bedded sandstones with wellrounded quartz and feldspar grains and minor amounts of mica (Peacock et al. 1968) which have been interpreted as the products of eolian deposition. Coarse pebbly sandstone lenses with small-scale cross-bedding also occur (Peacock 1966) which are interpreted as water-deposited. Contrary to other published reports, Maithel, Garner, and Whitmore (2015) found that the sandstone was not as well-sorted or the grains as well-rounded as previously reported. They noted that K-feldspar and muscovite in the formation could suggest non-eolian depositional processes for these facies. |
Juruá Formation | Lower Pennsylvanian | Brazil, 30–40 | Elias et al. 2004 | The Juruá Formation is one of the most important hydrocarbon reserves in Brazil. Elias et al. (2004) believe the formation, consisting of sandstones, mudrocks, evaporites and dolostones, was deposited in a coastal sabkha environment (p. 191). Microcrystalline dolomite is found especially in some of the sandstones that are interpreted to be eolian. Some of the sand is identified as eolian because of the presence of what are interpreted to be climbing translatent ripple strata. Some mica was reported in the “eolian” sandstones. |
La Colina Formation | Lower Permian | Argentina | Limarino and Spalletti 1986 | Limarino and Spalletti (1986) report the La Colina as a fine- to very fine-grained sandstone with largescale tabular cross-bedding with average dips of 26–31°. They report that Glossopteris casts give the formation a Lower Permian age. |
Locharbriggs Sandstone | Permian | Scotland, 1000 | Brookfield 1977, 1978; McKeever 1991 | The Locharbriggs Sandstone (Lower Permian) is known from outcrops in the Dumfries Basin of southwestern Scotland (Brookfield 1977) and is thought to have been deposited as transverse dunes (McKeever 1991). The overall thickness of the unit may be around 1000 m and it consists of large-scale cross-bedding and well-sorted fine- to medium-grained sand (Brookfield 1978). Vertebrate tracks were reported from this formation by McKeever (1991). Borsch et al. (2018) found muscovite in their thin sections. |
Los Reyunos Formation | Permian | Argentina | Limarino and Spalletti 1986; Mancuso et al. 2016 | Limarino and Spalletti (1986) report average crossbed dips of 20–23° and the presence of some trough cross-bedding. The sandstone is yellow to brick-red in color and contains frosted grains. Mancuso et al. (2016) report vertebrate tracks in cross-beds that dip from 15 to 20°. |
Ojo de Agua Formation | Permian | Argentina | Limarino and Spalletti 1986 | Limarino and Spalletti (1986) report eolian sandstones in the top of this formation, but the formation also contains mudstone, other sandstone and evaporite facies. |
Patquía Formation | Carboniferous to Permian | Argentina, >150 | Caselli and Limarino 2002; Krapovickas et al. 2010; Limarino and Spalletti 1986 | According to Caselli and Limarino (2002) the formation consists of a variety of different facies including conglomerates, breccias, sandstones (fine, medium and coarse), mudstones and tuffs. Eolian beds are represented by very fine to medium cross-bedded sandstones. Krapovickas et al. (2010) report a variety of fossils including the trace fossil Rusophycus and disarticulated fish remains. Of special interest are tetrapod footprints Chelichnus which are similar to other prints in Permian sandstones including the Coconino. |
Penrith Sandstone | Lower Permian | England, 100–400 | Arthurton, Burgess, and Holliday 1978; Lovell et al. 2006; Smith 1884; Waugh 1970a, 1970b | The formation reaches a maximum thickness of over 400 m in the Appleby-Hilton area (Arthurton, Burgess, and Holliday 1978). Published petrographic and grain size studies have reported that it is a well-sorted, well-rounded orthoquartzite, with subordinate K-feldspar and rock fragments (Waugh 1970a, 1970b). Detrital clay minerals and mica have been reported to be absent (Lovell et al. 2006) but Whitmore and Garner (2018) found mica in their thin sections. The large-scale crossbedding in the Penrith Sandstone is mostly wedge-planar with some tabular-planar and lenticular-trough units and foreset dips from 20° to 33° (Waugh 1970a, 1970b). Smith (1884) reports footprints from the Penrith. |
Pirambóia Formation | Permian | Brazil, 20–-200 | Delorenzo, Dias, and Scherer 2008; Francischini et al. 2018 | Delorenzo, Dias, and Scherer (2008) report the formation consists of three different facies: eolian sand sheets, eolian dunes and various interdune facies. The sand sheets are 4–9 m thick and are composed of fine- to very coarse-grained sand that is sorted and contains low-angle cross-bed dips. The dune facies have fine to medium, well-sorted, red sand. The grains are well-rounded and the cross-beds are large-scale. The authors recognize grainfall, grainflow and wind ripple strata in these facies. Francischini et al. (2018) report Chelichnus tetrapod tracks in the formation, similar to those found in the Coconino Sandstone. |
Rotliegendes and Wiessliegendes | Permian | Western continental Europe, UK, 225–1500 | Glennie 1972; Kiersnowski and Buniak 2016; McKee and Bigarella 1979 | The Lower Permian of Europe is characterized by an extensive red sandstone facies (the Rotliegendes) which is overlain by sandstones that are typically white or grey in color (the Wiessliegendes). The sands act as oil and gas reservoirs. Vertebrate tracks in the Rotliegendes are similar to tracks described in other sandstones of this age. McKee and Bigarella (1979) attribute this to eolian origin on account of its “steep” and extensive cross-beds, textural purity of well-sorted and rounded quartz grains (at least for the larger grains), and the association with salt, anhydrite and mud-cracked clays. Kiersnowski and Buniak (2016) describe the sandstone’s association with alluvial plain and marginal marine playa deposits. Glennie (1972) reports the sands as being deposited in a huge basin 2,000 km by 500 km. |
Santa Brígda Formation | Permian | Brazil | Jones, Scherer, and Kuchle 2016 | Jones, Scherer, and Kuchle (2016) describe the Caldeirão Member of the Santa Brígida Formation in eastern Brazil as eolian dune and interdune deposits. The facies interpreted as eolian is a subarkosic fine-grained sand that is well-sorted and well-rounded with high sphericity. Three types of strata are reported, that are characteristic of eolian deposits: grainflow (60%), translatent wind ripples (39%) and grainfall deposits (1%). Cross-bed sets are reported to be 1.0–3.5 m thick and the azimuths have a northeasterly direction. Some of the reported soft-sediment deformation features resemble pipes and others resemble parabolic recumbent folds. |
Unnamed sandstone in Maritimes Basin | Permian | Eastern Canada, Prince Edward Island area, 800 | Calder, Baird, and Urdang 2004; Gibling et al. 2008; Lavoie et al. 2009 | An “unnamed sandstone” is illustrated in Fig. 9 and 17 of Gibling et al. (2008, 221 and 231), with a thickness of about 800 m. Unfortunately, no further details are given. Calder, Baird, and Urdang (2004) described some trackways from sandstone in the Hillsborough Bay Formation; it is not known whether the “unnamed sandstone” is the same. The occurrence of trackways in this little-known rock unit is important because it may be helpful in demonstrating ties to the Permo-Triassic of Europe and the Permian sandstones of the western United States. Lavoie et al. (2009) mention an unnamed early Permian eolian sandstone on the Magdalen Islands in the Gulf of St. Lawrence. It is not known if this is the same sandstone described by Gibling et al. (2008). |
Unayzah A member | Middle Permian | Saudi Arabia, 45–90 | Melvin, Sprague, and Heine 2010 | There are several units described by Melvin, Sprague, and Heine (2010), primarily from drill core in Saudi Arabia. Eolian sandstones are primarily identified by well-sorted sand, fine-grains and frosting. Some of the laminations are at high angles. |
Yellow Sands | Lower Permian | England, 20 | Pryor 1971; Steele 1983; Versey 1925 | The Lower Permian Yellow Sands is usually described as fine- to coarse-grained and are said to consist of well-sorted, well-rounded to subangular clasts with common “frosting” of grain surfaces. Versey (1925) claimed that the Yellow Sands were the product of eolian processes, which is still the dominant view. However, Pryor (1971) challenged the eolian interpretation and argued that the Yellow Sands were deposited as a series of submarine sand ridges comparable to those on the modern North Sea shelf. He presented petrographic data showing that the Yellow Sands are in fact only poorly- to moderately-sorted, mostly subrounded, with >15% of the constituent grains being well-rounded and substantial amounts of subangular and angular grains. He documented the presence of muscovite and found crossbed dips were about 18°. Pryor (1971) argued that these features were indicative of a shallow marine origin, although his reinterpretation has not been generally accepted. |