The views expressed in this paper are those of the writer(s) and are not necessarily those of the ARJ Editor or Answers in Genesis.
Abstract
A literature review of eye evolution theories was completed. It was concluded that all attempts to explain the existence of the vertebrate eye by Darwinian evolution have failed. The eye is an excellent example of intelligent design and effectively illustrates the concept of irreducible complexity. In spite of numerous attempts during the past 150 years since Darwin, evolutionists have failed to offer a viable theory of eye evolution. The areas reviewed include its anatomy, physiology, genetics, and fossil record.
Introduction
The enormous success of vertebrates is linked to the camera-style eye and its sophisticated visual system (Gabbott et al. 2016). Eyes are critical for success because, for the “vast majority of animals,” vision is their “most important link to the world” outside (Sinclair 1985, xi). In the absence of reliable data from fossils, scenarios for the evolutionary assembly of the vertebrate eye have been based on evidence from embryo development, molecular genetics, and comparative anatomy of living vertebrates (Bredemeier 2016).
One of Darwin’s main concerns about his theory was the difficulty of both explaining and documenting eye evolution: “When Charles Darwin (1859) presented his theory of evolution he anticipated that the eye would become a favourite target for criticism. He openly admitted that the eye was by far the most serious threat to his theory” (Nilsson and Pelger 1994, 53). Darwin wrote:
To suppose that the eye with all its inimitable contrivances for adjusting the focus to different distances, for admitting different amounts of light, and for the correction of spherical and chromatic aberration, could have been formed by natural selection, seems, I confess, absurd in the highest degree. (Darwin 1859, 186)
He then attempted to answer his own challenge, concluding that many small, slight variations could eventually produce even a camera-type eye. Darwin’s attempt to build his case relied on a set of “ifs” and similar terms I have signified in bold italics to emphasize the fact that Darwin recognized that his entire case was very tenuous:
if numerous gradations from a perfect and complex eye to one very imperfect and simple, each grade being useful to its possessor, can be shown to exist; if further, the eye does vary ever so slightly, and the variations be inherited, which is certainly the case; and if any variation or modification in the organ be ever useful to an animal under changing conditions of life, then the difficulty of believing that a perfect and complex eye could be formed by natural selection, though insuperable [a difficulty impossible to overcome] by our imagination, can hardly be considered real. (Darwin 1859, 186–187)
As Darwin recognized, his scenario has been documented to be implausible by the observations and research reviewed below. A major problem is that a chasm exists between the proposed precursor of the vertebrate eye, (the eyespot), and the simplest known vertebrate eye (Gehring 2004). Melnick concluded that the eye is a marvel and that “its immense complexity and diversity in nature, as well as its beauty and perfection in so many different creatures of the world, defies explanation even by macroevolution’s most ardent supporters.” (Melnick 1981, 26)
Evolution from the Eyespot to the Camera Eye
No shortage of articles that discuss Darwin’s eye evolution theory exists, and this review focuses on one specific eye design, the camera eye. Specifically, a camera eye is far more complex than its proposed eyespot precursor (a bulge or convex protrusion of the cellular layer basically similar to a cup with a pinhole) (Schwab 2018, 304). The camera eye has numerous parts, including a cornea, a lens, a set of extraocular muscles, and ocular adnexa (the parts adjoining the organ), all of which must be functional and highly integrated to be able to work as a unit. The retina, optic nerve, and the processing center in the occipital lobe of the brain are only briefly mentioned here, and each of these innovations deserves an entire article of its own.
The simplest “eye,” the eyespot, is actually not an eye, but rather a set of pigmented cells used for phototaxis. Phototaxis is the bodily movement of motile organisms in response to light, either toward the source of light (positive phototaxis) or away from it (negative phototaxis). The eyespot involves light-sensitive cells in the epidermis (skin) of some invertebrate animals including worms and starfishes (Arendt 2003; Arendt, Hausen, and Purschke 2009). Beyond sensing light, eyespots do not serve the same functions as an eye. Phototaxis functioning requires an enormously complex mechanism, thus documenting that the claimed first step in eye evolution is problematic. For an organism lacking a functional eyespot to develop a primitive eyespot functional enough to confer an evolutionary benefit, the
organism would need to solve several problems to gain this minimum functionality. It would need to be able to detect the presence of light (the eyespot), the ability to swim toward that light (the flagella), and a signaling system from the primitive eyespot to the flagella. Otherwise, given that it could swim, and could detect where it wanted to swim, if there are no means of communication between the eyespot and the flagella, there is not a functional advantage. (Siewert and Siewert 2017, 689)
The gap between an eyespot and the camera eye design is so great that Nilsson and Pelger speculated that the evolution from a light-sensitive patch to a fully formed camera-style eye could take as long as 364,000 years (Nilsson and Pelger 1994, 58). The problem is that the “just-so” stories which are created to fit this scenario are just that: stories that fail to explain how each step of small continuous change could be functional and improve vision. In fact, the vast majority of changes would produce a non-functional structure unable to achieve usable vision and prone to dysfunction. The most pervasive evidence for this conclusion is the well-documented fact that the vast majority of mutations are near neutral or harmful. Very few produce changes that are beneficial (Jones 2014, 1).
The Eye Muscles
For the human eye to function properly, all six extraocular muscles (medial rectus, superior rectus, inferior rectus, lateral rectus, superior oblique, and inferior oblique) must have both proper origin and insertion connections, and functional neuronal connections to the brain. Lacking one extraocular muscle would cause the eye to move in an uncoordinated way. Even lack of coordination between the six extraocular muscles is a problem, as illustrated by the condition called strabismus, a form of lazy eye. Strabismus is often caused by abnormal weakness of extraocular (or oculomotor) muscles due to genetic myopathies, pathologies of neurotransmitter transmission at the neuromuscular junction, or abnormal development of the extraocular muscles (Quoc and Milleret 2014, 1–4). These conditions illustrate the fact that proper eye function is dependent on both muscle and neural development and health.
In contrast to one headline that proclaimed “Darwin’s Greatest Challenge Tackled: The Mystery Of Eye Evolution,” the belief that the evolution-of-the-eye problem has been solved is grossly premature. For example, University of Vienna professor and biologist Kristin Tessmar-Raible claimed to have solved the problem by studying a “living fossil,” a marine worm that she assumed resembled our early ancestors which lived as long as “600 million years ago,” the Platynereis dumerilii. She attempted to determine
the molecular fingerprint of the cells in the worm’s brain. She found an opsin, a light-sensitive molecule, in the worm that strikingly resembled the opsin in the vertebrate rods and cones. “When I saw this vertebrate-type molecule active in the cells of the Playtnereis brain—it was clear that these cells and the vertebrate rods and cones shared a molecular fingerprint. This was concrete evidence of common evolutionary origin. We had finally solved one of the big mysteries in human eye evolution.” (European Molecular Biology Laboratory 2004)
The opsin molecule must be designed a certain way to produce functional opsin. This molecule is responsible for daylight vision, (in contrast to night vision), called phototropic vision. Only a very small number of molecular designs are capable of functioning in the retina to detect light (Hecht, Shlar, and Pirenne 1942). This and other facts do not support the hypothesis, discussed below, that the eye evolved several times.
The major contention used to explain the lack of evidence for eye evolution, (that soft tissue does not preserve well in the fossil record), has been negated by the recent soft tissue preservation examples. One example is “the recent discovery of fossil fish eyes with ultra-structural fidelity of preservation [which] has shown that, albeit rarely, the fossil record may capture detailed aspects of eye anatomy” (Gabbott et al. 2016). Another example is the fossil of a jawless fish, the lamprey, dated by evolutionists to be “300 million years old,” which had eyes “so well preserved that the retina and lens can be seen in high magnification” (Beall 2016). All such examples located to date have not supported eye evolution claims.
Eye Designs
The compound eye design family consists of hundreds to thousands of ommatidia, which are independent photoreception units. Each unit consists of a cornea, a lens, and photoreceptor cells. This system is employed by most insects and many crustaceans. It produces poor image resolution but is an excellent design for detecting movement in the front and to the side of the animal. The following is a description of different types of compound eyes:
The apposition eye is a compound eye that is characteristic of diurnal insects and employed on all arthropods and some annelids (segmented worms) and bivalves (clams, oysters, and scallops). Light reaches the retina of each ommatidium as a single spot and the image is a composite of all of these spots. In another kind of apposition eye, the schizochroal compound eye, each lens forms an image. These separate images are then combined in the brain. A superposition eye is used in insects that fly at night or in twilight. The pigment received from neighboring facets overlaps to absorb more light. The refracting superposition eye has a gap between the lens and the rhabdom (the translucent, light-sensitive receptor component of the arthropod eye), and lacks a side wall. Each lens absorbs light at an angle and reflects it to the same angle on the other side. The result is an image of half the radius of the eye, but it produces images up to 1,000 times brighter than the equivalent apposition eyes, though with much reduced resolution. These eye types are of such different design that many evolutionists have proposed that they evolved separately.
The Camera-Type Eye
Evolutionists claim that the camera eye most likely evolved from the compound eye. The problem is that the contrast between the camera-type eye and the compound eye, discussed above, is dynamic. Compare fig. 1 of a compound eye and fig. 2 of a camera-type eye). Since all vertebrate eyes are basically similar in structure, the human eye is used as the example in this paper (Goin and Goin 1966, 181). Light in a camera-type eye enters the cornea, passes through a single lens into the liquid vitreous humor and, lastly, strikes the retina. The opsin molecule, noted above, is the molecule responsible for the conversion of light energy into electrical energy for transmission via the optic nerve to the appropriate area of the brain for processing.
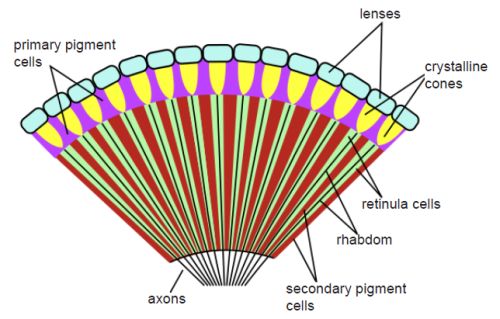
Fig. 1. A compound-type eye. The illustration shows a cross-sectional diagram of an insect’s compound eye. From Wikimedia Commons. https://upload.wikimedia.org/wikipedia/commons/thumb/0/01/Insect_compound_eye_diagram.svg/591px-Insect_compound_eye_diagram.svg.png.
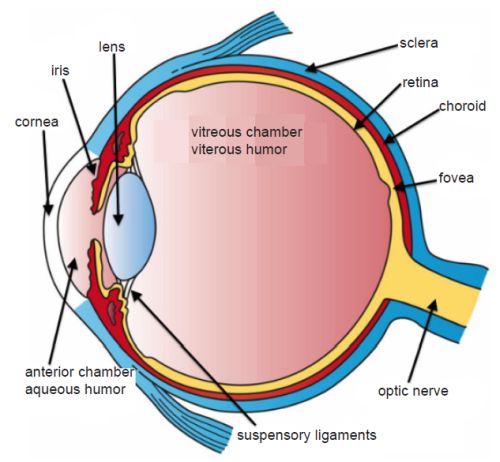
Fig. 2. A camera-type eye showing a cross section. Note these two eye designs are very different. From Wikimedia Commons. https://upload.wikimedia.org/wikipedia/commons/d/d0/Three_Main_Layers_of_the_Eye.png. Artwork by Holly Fischer.
The iris diaphragm can open or close to control the amount of light allowed into the eye. The opening that allows light into the eye, called the pupil, appears black because most of the light entering the eye is not reflected back. The cornea protects the structures inside the eye, contributes to the eye’s refractive power, and helps to focus light rays on the retina with minimum scatter and optical degradation.
The flexible crystalline lens changes shape by the relaxation and contraction of the ciliary muscles to allow the eye to focus on objects at varying distances from the viewer. This process is called accommodation. The lens shape is changed by ciliary muscles connected at the side of the lens system. The ciliary muscle surrounds the lens in a circumferential fashion and is attached to the lens capsule by the zonules of Zinn. The retina contains six million cones that allow us to see the world in all its colorful hues. They work together with 120 million rods, which provide black and white vision.
The cones are concentrated in the part of the eye’s retina called the macula, which enables small details to come into sharp focus. The three cone types are the red-sensing cones (60%), green-sensing cones (30%), and blue-sensing cones (10%). These three cone types, each of which can register about 100 different color shades, allow us to see close to a million different colors. The human camera eye is designed to resolve at a level of detail higher than most other eye designs can achieve.
The Evolution of the Camera Eye
The earliest known vertebrate eye is that of the conodonts, an eel-like vertebrate that “had rather large and well-organized eyes,” so well-developed that the author declared that they document “how quickly eyes can emerge” by evolution (Schwab 2012, 65). The problem is that no evidence exists of their evolution. All examples that have been examined are fully developed eyes, and this includes both those used in invertebrates and vertebrates. Even the so-called “simple eye,” such as the shrimp eye, is problematic in that though
this animal’s eye is the simplest compound-eye morphology, in some ways, it is the most complex as the eye in an invertebrate, and probably in the animal kingdom. . . . Fossils closely resembling this animal have been [claimed by evolutionists] . . . to be 400 million years old, and it is likely that this animal has changed minimally over that range of time. (Schwab 2012, 54)
The Case of the Lamprey
Another early, fully developed eye is that of the cyclostome, (the lamprey), which had photoreceptors, bipolar cells, an optic nerve, and ganglion cells. It is called “a complex structure which must have had a long history, but we know nothing of that history” (Goin and Goin 1966, 183). In other words, the lamprey eye is almost identical to the modern vertebrate eye, but instead of acknowledging the possibility that the first eye was a modern, fully functional eye, evolutionists must
Nor do we have a viable “just-so” story that postulates how the eye could have evolved from some hypothetical light-sensitive cell. For adult lampreys, “vision is critical” for its survival (Schwab 2012, 68). In short, all vertebrates, including the most primitive of all known vertebrate species, the lamprey, have an eye that is “remarkably similar to our own” and possess a “sophisticated eye with a lens, iris and eye muscles” like our camera eye (Gabbott et al. 2016, 6; Lamb, Collin, and Pugh 2007; Schwab 2012, 68). The southern lamprey has all four known major types of visual pigments as do humans. Furthermore,
Lampreys, which represent the oldest group of living vertebrates (cyclostomes), show unique eye development. The lamprey larva has only eyespot-like immature eyes beneath a non-transparent skin, whereas after metamorphosis, the adult has well-developed image-forming camera eyes. (Suzuki and Grillner 2018)
Lampreys also “possess[es] numerous features (including the expression of opsin genes) that are very similar to those of the eyes of jawed vertebrates” (Lamb, Collin, and Pugh 2007, 4). Thus, it has been argued that it reflects a “rudimentary intermediate evolutionary grade in the gradual assembly of the vertebrate eye” (Gabbott et al. 2016, 1).
The Hagfish Example
Another suggested evolutionary intermediate between the invertebrate eyespots and the vertebrate camera eye is the hagfish. Critical evaluation of the hagfish has shown that “the hypothesis that hagfish eyes reflect, or [are] a faithful vestige of such an ancestral state” is also questionable (Gabbott et al. 2016, 6). The most detailed study has determined that the eyes of extant hagfish are degenerate, not primitive and simple. Furthermore, evolutionary
steps in the transition from a light-sensitive ‘eyespot’ in invertebrate chordates to an image-forming camera-style eye in jawed vertebrates are constrained only by hagfish and lampreys (cyclostomes), which are interpreted to reflect either an intermediate or degenerate condition. (Gabbott et al. 2016)
According to the researchers, “Numerous features of hagfish eyes are far simpler than those of vertebrate eyes, and . . . the eyes of extant hagfish are likely to be similar to the eyes possessed by our own ancestors, some 530 Mya” (Lamb 2016, 1). Lamb concluded that this eye did not exhibit image-forming capabilities, and that its function was instead non-visual, possibly for regulating circadian cycles occurring in approximately 24-hour periods (Lamb, Collin, and Pugh 2007). Thus, we are comparing the gap between a non-vertebrate structure and all vertebrate eyes:
The modern vertebrate eye was proposed to have evolved from an eye similar to the modern Hagfish eye. Thus the problem is to determine the evolution of the Hagfish, which have a skull but no defined vertebral column.
Along with lampreys, hagfish are jawless; the two form the sister group to jawed vertebrates, and living hagfish remain similar to hagfish from around 300 million years ago. (Wikipedia 2022)
So many major differences exist between invertebrates and vertebrates that Goin and Goin write that computer models and deductive reasoning indicate that “eyes may have evolved as many as 40 times during metazoan development” (Goin and Goin 1966, 181). The problem with this speculation is that it has been shown that it is very unlikely that the eye evolved even once. To evolve 40 times is far beyond probable. Another problem for evolution is that the vertebrate eye is a highly specialized structure that has no true homologue in any other phyla. The closest example is the octopus eye, which is a camera-type eye, but uses a very different retina design.
Tapetum Lucidum in Vertebrates
In addition to the pigmented epithelium, many vertebrates contain a structure called a tapetum lucidum. The tapetum effectively reflects incoming light back to the rods, allowing them to absorb a greater level of light energy, thus providing greater visual acuity in low-light levels (Ali and Klyne 1985). One result is that a cat’s night vision is estimated to be six times better than humans. They can see fairly well in light that humans would perceive as close to pitch black.
The tapetum’s importance is indicated by the fact that the pigment epithelium which covers the tapetum is “always devoid of pigment so that there is no interference with the back reflection of the light” (Ali and Klyne 1985, 125). It is the tapetum that produces the reflective eyes characteristic of nocturnal animals, giving cats, dogs, and deep-sea or turbid-water fish eyes their distinctive night glow called eyeshine. It is an example of a unique, well-designed structure used by various vertebrates.
This structure allows vertebrates to see much better in dim light but at the cost of much poorer resolution and visual clarity during daylight. The cat has a higher concentration of rods to cones compared to humans; and consequently, they are more sensitive to low light. Conversely, compared to humans they have much less resolving power and an inferior ability to detect colors (Land and Nilsson 2005, 38). Animals with a tapetum usually have poor daylight vision and many use highly contractile pupils to protect their retina.
Although evolutionists claim that the tapetum lucidum retro-reflector evolved, animals either have this structure, or lack it, and no evidence exists of an intermediary structure, nor of its evolution. Many different variations of the tapetum exist, but the basic design is the same, even though the tapetal reflectance material used in the eye varies considerably. Differences include tapetum made of crystals, or tapetum made of regularly arranged fibers. Many fish have tapetum made of guanine crystals, carnivores have tapetum made of zinc-cysteine crystals, and herbivores such as the sheep and cow have fibrous tapetum. Conversely, the design is so similar that evolutionists claim that it is an example of convergent evolution (Schwab et al. 2002, 187). A progression fitting evolution has eluded evolutionists. Some animals, such as certain reptiles, possess a guanine tapetum but other similar animals, such as mammal-like reptiles, lack it (Schwab et al. 2002, 194).
Genetic Analysis
The camera eye is produced and regulated by over 1,000 genes and the retina assembly is controlled by approximately 20,000 genes (Williams and Moody 1987). More than 760 eye genes were conserved in both flies and worms, a fact supporting the conclusion that the animal eye, even the most simple one, represents a design chasm from an eyespot design, which has very few of these genes (Ogura, Ikeo, and Gojobori 2004). The transcription factor Pax6 is an important regulator of early animal development. Significantly, loss of “function mutations of pax6 in a range of animals result in a reduction or complete loss of the eye” (Klann and Seaver 2019, 86). The fact that damage to the pax6-eye-specific cascade causes the loss of a functional eye in all animal groups is clear evidence for major genetic similarity of all eye designs. Nor does this finding support the multie-volution theory.
Furthermore, when genetic analysis is used to make eye design comparisons, the “40 separate eye evolutions” claimed by evolutionists is also problematic. One example of this multi-evolution claim is that, “Although the camera eye of the octopus is very similar to that of humans,” phylogenetic and embryological analyses argue that the differences are so great that they evolved separately (Ogura, Ikeo, and Gojobori 2004, 1555). However, Gehring writes that, based on
morphological criteria and the different modes of development of the different kinds of eyes, neodarwinists have postulated that the various eye-types are polyphyletic in origin and that the eyes have evolved independently in the various animal phyla. Recent developmental genetic experiments and molecular phylogenetic analyses cast serious doubts on this interpretation and argue strongly for a monophyletic origin of the eyes from a Darwinian prototype and subsequent divergent, parallel and convergent evolution leading to the various eye-types. (Gehring 2004)
In addition, the pax6 eye-specifying cascade is seen to support the view “that animal eyes evolved from a common, simple precursor, the proto-eye” (Arendt 2003, 563).
Structures and Function of Avian Eyes
The eyes of birds not only produce the sharpest vision of all known animals, but they can form sharp images on all areas of their inverted retina. They also have two to five times the number of cones per square millimeter compared to humans (Gill 2007, 186). As Yale ornithologist Richard Prum concluded: “Avian vision is more complex than we ever realized” (quoted in Dalton 2004, 596).
Birds also rely on a large structure which protrudes into the retina, called a pectin, that replaces the embedded blood vessels in mammals. This system interferes less with vision than would a network of blood vessels and is another reason why birds have unusually high visual acuity (Gill 2007, 188). Many reptiles have a structure similar to the pectin, called a conus papillaris, which is more cone-like, and often differs in other ways from the pectin design.
Birds are also sensitive to light in the near-ultraviolet spectrum. This sensitivity is produced by red oil droplets located in the lower part of their eyeball cavity which enhances the contrast of objects. One benefit is to be able to see otherwise disguised animals in a green foliage background. Furthermore, their eyeball contains yellow droplets in the upper area of the eyeball that enhances objects seen against the sky by filtering out much of the blue background. The two different oils are kept separate by density differences. All of these modifications help animals with camera-type eyes to see in their world, but would be a major handicap to humans in our terrestrial, ground-oriented world.
Summary
Schwab, in an understatement, acknowledged that: “Evolutionary controversy surrounds the eye” such as “did the eye evolve just once, or many times?” The reason evolutionists argue that the eye must have evolved many times is because “the fundamental differences between the basic photoreceptive cells of invertebrates and vertebrates” are so great that the eye must have “evolved more than once” (Schwab 2012, 24). The multiple evolution claim is driven by the fact that one eye type evolving into another type cannot be bridged even by made-up “just-so” stories (Bergman 2008). Evolutionists admit that “the evolution of animal eyes is a story of a stunning rise in complexity, from a very simple common evolutionary precursor, the hypothetical ‘proto-eye’—a simple organ composed of two functionally distinct cells, a light-sensitive photoreceptor cell (PRC) and a shading pigment cell (SPC)—to something as intricate as the human eye or the cephalopod eye” (Arendt, Hausen, and Purschke 2009, 2809). Explaining how this could, or did, happen has proven very difficult.
Although as many as 40 different eye designs exist, the vertebrate eye is in a class by itself. Few differences exist between the different camera eyes employed by vertebrates, but a chasm exists between the vertebrate camera eye and the compound invertebrate eye. Although the origin of the first eye is unknown, the variety existing today is enormous (Schwab 2012, 39).
Many structural similarities exist which are due to design constraints. This is because there are only a few chemical and design possibilities that can be used in designing a seeing eye.
As Arendt concludes: the “evolution of the eye has been debated ever since Darwin’s Origin of Species” and is still being debated among Darwinists (Arendt 2003, 563). For non-evolutionists there is no debate because they know that there exists “scant evidence for understanding the origins and evolution of sensory systems in basal vertebrates” (Gabbott et al. 2016, 2). The fact is that “The evolution of the eye is one of the most complicated and interesting stories for molecular biologists and molecular evolutionists” (Ogura, Ikeo, and Gojobori 2004, 1555). Indeed, the fact of the matter, based on the evidence, is that the Creator made “the seeing eye” (Proverbs 20:12).
References
Ali, M. A., and M. A. Klyne. 1985. Vision in Vertebrates. New York, New York: Springer Publishing.
Arendt, Detlev. 2003. “Evolution of Eyes and Photoreceptor Cell Types.” International Journal of Developmental Biology 47, no. 7–8 (February): 563–571.
Arendt, Detlev, Harald Hausen, and Günter Purschke. 2009. “The ‘Division of Labour’ Model of Eye Evolution.” Philosophical Transactions of the Royal Society 364, no. 1531 (October 12): 2809–2817.
Beall, Abigail. 2016. “The Fish That Went Through a ‘Reverse Evolution’: Blind Hagfish Could See 300 Million Years Ago.” Daily Mail, 3 August. https://www.dailymail.co.uk/sciencetech/article-3720222/The-fish-went-reverse-evolution-Blind-hagfish-300-million-years-ago.html.
Bergman, Jerry. 2008. “Did Eyes Evolve by Darwinian Mechanisms?” Journal of Creation 22, no. 2 (August): 67–74.
Bredemeier, Greg. 2016. The Collapse of Darwinism: How Medical Science Proves Evolution by Natural Selection is a Failed Theory. Bloomington, Idaho: WestBow Press.
Dalton, Rex. 2004. “True Colours.” Nature 428, no. 6983 (8 April): 596–597.
Darwin, Charles. 1859. On the Origin of Species by Means of Natural Selection. London, United Kingdom: John Murray.
Dong, Emily M. and W. Ted Allison. 2021. “Vertebrate Features Revealed in the Rudimentary Eye of the Pacific Hagfish (Eptatretus stoutii).” Proceedings of the Royal Society B, 288 (no. 1942) (13 January): 1–9. https://doi.org/10.1098/rspb.2020.2187.
European Molecular Biology Laboratory. 2004. “Darwin’s Greatest Challenge Tackled: The Mystery Of Eye Evolution.” ScienceDaily, 1 November. www.sciencedaily.com/releases/2004/10/041030215105.htm.
Gabbott, Sarah E., Philip C. J. Donoghue, Robert S. Sansom, Jakob Vinther, Andrei Dolocan, and Mark A Purnell. 2016. “Pigmented Anatomy in Carboniferous Cyclostomes and the Evolution of the Vertebrate Eye.” Proceedings of the Royal Society B, 283, no. 1836 (17 August): 1–8. http://dx.doi.org/10.1098/rspb.2016.1151.
Gehring, Walter J. 2004. “Historical Perspective on the Development and Evolution of Eyes and Photoreceptors.” International Journal of Developmental Biology 48, no. 8–9 (February): 707–717.
Gill, Frank B. 2007. Ornithology. New York, New York: Macmillan Publishers.
Goin, Coleman J., and Olive B. Goin. 1966. Comparative Vertebrate Anatomy. New York, New York: Barnes and Noble.
Hecht, Selig, Simon Shlar, Maurice Henri Pirenne. 1942. “Energy, Quanta, and Vision.” Journal of General Physiology 25, no. 6 (July 20): 819–840.
Jones, Chelsea K., 2014. “The Distribution of Fitness Effects of Spontaneous Mutations in Vibrio fischeri.” B. Sc. Hons., University of New Hampshire. https://scholars.unh.edu/cgi/viewcontent.cgi?article=1196&context=honors.
Klann, Marleen, and Elaine C. Seaver. 2019. “Functional Role of pax6 During Eye and Nervous System Development in the Annelid Capitella teleta.” Developmental Biology 456, no. 1 (December): 86–103.
Lamb, Trevor, Shaun P. Collin, and Edward N. Pugh Jr. 2007. “Evolution of the Vertebrate Eye: Opsins, Photoreceptors, Retina and Eye Cup.“ Nature Reviews Neuroscience 8, no. 12 (December): 960–976.
Land, Michael F., and Dan-Eric Nilsson. 2005. Animal Eyes. New York, New York: Oxford University Press.
Melnick, Jim. 1981. “Vision: An Evolutionary Enigma.” Christian Citizen 1, no. 9 (November): 26.
Nilsson, Dan-Eric, and Susanne Pelger. 1994. “A Pessimistic Estimate of the Time Required for an Eye to Evolve.” Proceedings of the Royal Society B 256, no. 1345 (22 April): 53–58.
Ogura, Atsushi, Kazuho Ikeo, and Takashi Gojobori. 2004. “Comparative Analysis of Gene Expression for Convergent Evolution of Camera Eye Between Octopus and Human.” Genome Research 14, no. 8 (August): 1555–1561.
Quoc, Emmanuel Bui, and Chantal Milleret. 2014. “Origins of Strabismus and Loss of Binocular Vision.” Frontiers in Integrative Neuroscience 8, no. 71 (September 25): 1–19.
Schwab, Ivan, Carlton K. Yuen, Nedim C. Buyukmihci, Thomas N. Blankenship, and Paul G. Fitzgerald. 2002. “Evolution of the Tapetum.” Transactions of the American Ophthalmological Society 100 (February): 187–200.
Schwab, Ivan R. 2012. Evolution’s Witness: How Eyes Evolved. New York, New York: Oxford University Press.
Schwab, Ivan R. 2018. “The Evolution of Eyes: Major Steps. The Keeler lecture 2017: Centenary of Keeler Ltd.” Eye (London) 32, no. 2 (October 20): 302–313
Siewert, Elizabeth A., and Thomas A. Siewert. 2017. “The need for a quantifiable model of evolution.” Journal of Biological Innovations 6, no. 5 (September): 685–694.
Sinclair, Sandra. 1985. How Animals See: Other Visions of Our World. New York, New York: Facts on File.
Suzuki, Daichi G., and Sten Grillner. 2018. “The Stepwise Development of the Lamprey Visual System and its Evolutionary Implications.” Biological Reviews of the Cambridge Philosophical Society 93, no. 3 (August): 1461–1477.
Wikipedia. 2022. “Hagfish.” https://en.wikipedia.org/wiki/Hagfish.
Williams, Robert W., and Sally A. Moody. 1987. “Development and Genetic Control of Cell Number in the Retina.” The Visual Neurosciences, edited by L. M. Chalupa and J. S. Werner, 63–76. Cambridge, Masschusetts: Bradford Book.
Featured Topics
You May Also Like
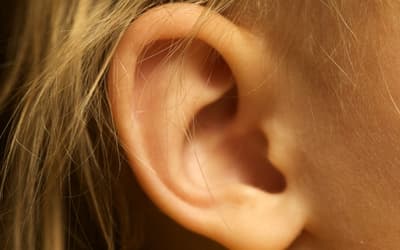
Cartilage Evolution Baffles Evolutionists
Dr. Jerry Bergman • Aug. 17, 2022
This review of attempts to postulate evolutionary theories for the origin and development of cartilage and the cells that produce cartilage.
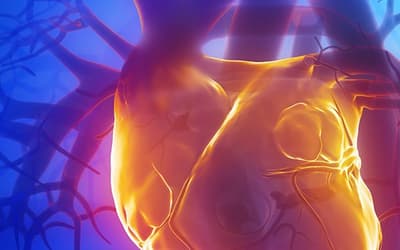
Heart Evolution: The Four Heart Types Are Unbridgeable by Evolution
Dr. Jerry Bergman • June 8, 2022
Not even plausible “just-so” stories are proposed to explain how one heart system evolved into a different system. Evolution is unable to explain their origin.
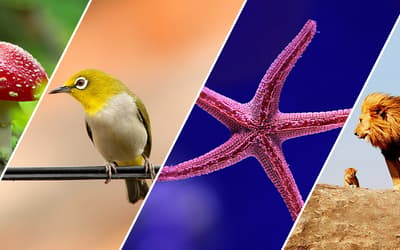
The Evolution of Asexual to Sexual Reproduction: The Queen of Evolutionary Problems
Dr. Jerry Bergman • Dec. 15, 2021
The evidence is overwhelming and widely recognized by evolutionists that evolution by small steps cannot bridge the transition from asexual to sexual reproduction.
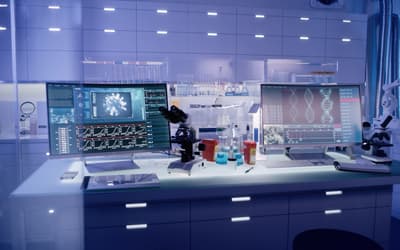
The History of Using Radiation to Speed Up Evolution
Dr. Jerry Bergman • March 24, 2021
The history of the mutation theory as the foundation of the source of new genetic information is reviewed.