The views expressed in this paper are those of the writer(s) and are not necessarily those of the ARJ Editor or Answers in Genesis.
Abstract
A literature search for evidence of the evolution of the mollusk shell was completed. Little to no physical evidence exists of the theorized gradual transformation from some proposed common ancestor of mollusks. This is true in spite of the excellent preservation qualities of the shell and a fossil record exceeding most all other Phyla. This putative “simple” organism manifests complexity at such a high level that scientists have been unable to hypothesize its possible evolution. The mollusk shell is also an excellent example of an irreducibly complex structure. The shell is an exoskeleton that is integrated into the design of the entire animal.
Keywords: mollusk shell evolution, clam evolution, oyster evolution, evolution, fossil record, irreducible complexity, calcium carbonate
Introduction
Seashells serve as a home for many kinds of invertebrates, including snails, clams, oysters, and other mollusks (fig. 1). Shelled mollusks live in oceans, freshwater or other aquatic environments, and some even live on land. Phylum Mollusca is one of the largest and most successful animal kingdom phyla. Mollusks’ external morphology and size (ranging from microscopic clams and snails to the giant oceanic squid) are enormously diverse (Seed 1983).
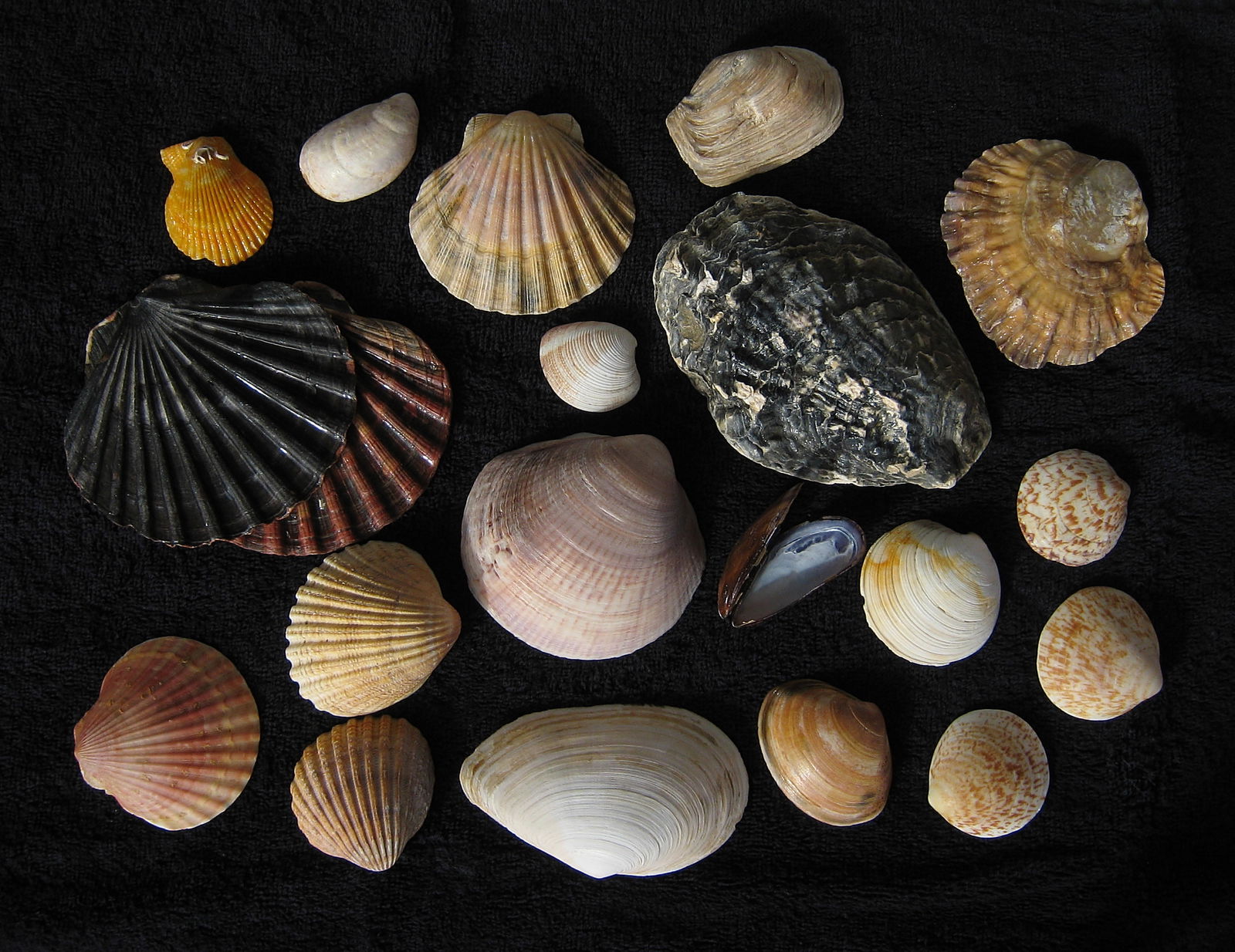
Fig. 1. A picture which illustrates the variety of clam shells. Manfred Heyde, “Different sea shells,” https://commons.wikimedia.org/wiki/File:Shells_Vacation.jpg, GFDL.
Malacology is the scientific study of mollusks as living organisms. Conchology is the branch of malacology devoted to the study of their shells. The mollusk shell is typically a calcareous exoskeleton which encloses, supports, and protects the soft parts of an animal in the Phylum Mollusca (Falini et al. 1996). Other life-forms also produce shells, such as crabs and lobsters, which are very different design then the calcium carbonate mollusk shell. This review covers only those shells produced by mollusks.
Mollusk shells protect all, or most, of an animal and serve as the organism’s first level of protection. Their shells also serve as exoskeletons and, unlike typical animal skeletons, they are not constructed from cells. The shell is produced by, and is part of, the mollusk invertebrate body. They are composed mostly of calcium carbonate (CaCO3), similar to bird eggshells. Two different forms of calcium carbonate are required to produce a mollusk shell: calcite and aragonite (Falini et al. 1996). The crystal system of calcite is trigonal, whereas the crystal system of aragonite is orthorhombic. Calcite is a major ingredient of marble, coral, and limestone.
The mollusk shells also contain small quantities of protein. Although only around 2%, the protein is critical for the shell’s required structure, growth, and function. Specifically, the protein serves the same function that steel rebar serves in concrete, namely it provides shape and support. In concrete, cement produces compressive strength and the rebar produces tensile strength. Likewise, the calcium carbonate in the mollusk shell produces compressive strength, and the protein tensile strength (Ramnath et al. 2018).
How the Shell is Manufactured
The animal absorbs calcium from its marine environment which is then employed to form the shell structure. The mollusk cells, directed by the designed genetic program, construct the shell from numerous raw materials. The shell is a complex structure that must be constructed properly to function in its many critical roles for the mollusk. The shell material is secreted by the ectodermal epithelial cells in the mantle tissue of the mollusk’s body. These cells secrete many different proteins and minerals required to construct the different layers of the shell. They also add minerals to the shell to increase its size and strength as the animal grows.
Hundreds of soluble and insoluble proteins control shell formation (Jackson, Wörheide, and Degnan 2007). The proteins are the major macromolecules that assemble and control the biocrystal synthesis to produce the functional shell. Shell formation requires complex biological machinery, complexity at such a level that the exact process used to create the shell is still poorly understood. Marin et al. writes:
Emerging models try to translate a more complex reality, illustrated by the huge variety of shell proteins characterized so far. The primary structure of many of them is composed of different functional domains, some of which exhibit enzymatic activity, while others may be involved in cell signaling. Many of them have unknown functions. Today, the shell matrix appears as a whole system, which regulates protein-mineral, protein-protein, and epithelium-mineral interactions. These aspects should be taken into account for the future models of shell formation. (Marin, Le Roy, and Marie 2012)
Until the structure of the shell system existed at the level of complexity required to function, the animal could not survive. Consequently, the shell is an excellent example of irreducible complexity. This is one reason why evolutionists have been unable to hypothesize a viable scenario of their evolution from non-shells. Research focusing on the complex biomineral shell structure has identified a large
number of shell matrix protein components. Although the matrix is quantitatively a minor constituent in the shell of mollusks (less than 5% w/w), it is, however, the major component that controls different aspects of the shell formation processes; synthesis of transient amorphous minerals and evolution to crystalline phases, choice of the calcium carbonate polymorph (calcite vs aragonite), [and] organization of crystallites in complex shell textures (microstructures). (Marin, F. et al. 2007)
The Manufacturing of the Shell
Various proteins cause calcium carbonate in the shell to crystallize in different ways (Belcher et al. 1996). This is one of several mechanisms used to create the critical internal differences in the shell design. For example, the innermost shell layer is in contact with the mollusk mantle, which requires a specific design. This smooth iridescent innermost layer of calcium carbonate formed of aragonite crystals is called “nacre” or “mother-of-pearl”. Nacre, although made up of protein and calcium carbonate like the rest of the shell, looks and feels completely different from other shell parts. The proteins in this layer enable the mollusk’s soft body organs to freely move in a friction-free environment within the shell. The middle (prismatic) layer of the mollusk shell requires a very different design. It is composed primarily of calcite, while the outer protective layer, the periostracum layer, is a thin protective cover to help protect the shell’s inner part.
As the mollusk grows, its shell must also grow. For clam or mussel bivalves, growth is at the outer edges where the two shells separate. Snails add to their shell around the opening where the head protrudes. The result is that growth rings are produced like those in a tree, which allow measuring the age of these mollusks. The two halves of the bivalve design are joined by a hinge and kept firmly closed by strong abductor muscles (Abbott 1962, 129).
When a clam dies, the shell opens up, allowing the soft tissue to either be consumed by another animal, or decompose, leaving behind the shell (Horne 2006) (fig. 2). Because the shell is weather-resistant, if buried, it can outlast the mollusk that made it. The abundance of shells is one reason why collecting some of the estimated 100,000 known species of seashells is a very popular hobby today among all social levels (Abbott 1962). Phylum Mollusca is second only to the Arthropoda in terms of total species diversity (Abbott 1991). Most often, though, when the animal inside dies, its shell is pounded against the rocks and sand by the water’s waves. As a result, the shells gradually break down, becoming intermixed as a major constituent among the beach’s quartz sediments.
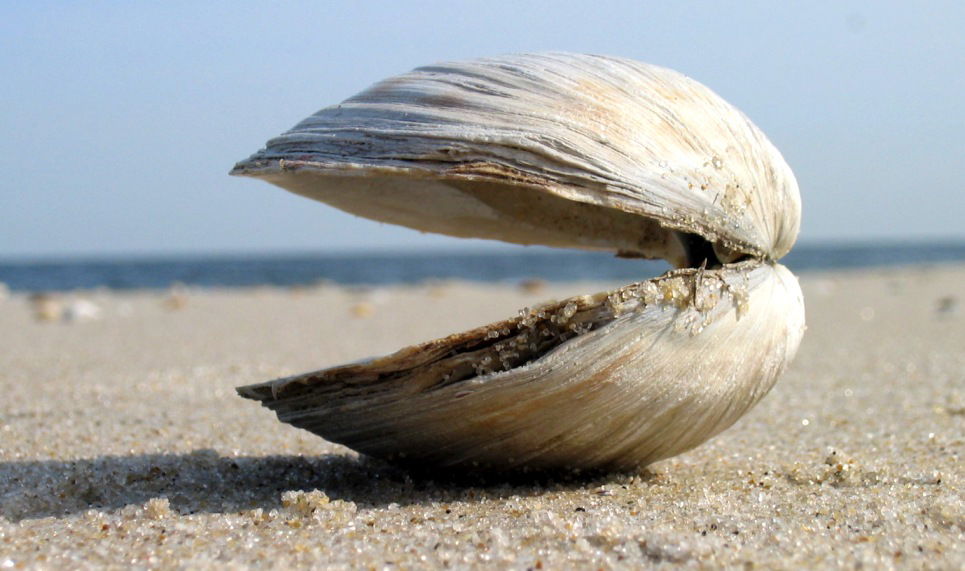
Fig. 2. A clam shell in which the clam has died and the bivalve shell is now open. febb, “Clams on Sandy Hook beaches,” https://en.m.wikipedia.org/wiki/File:Clams_on_Sandy_Hook_beaches_-_panoramio.jpg, CC BY-SA 3.0.
The Mantle
The mollusk shell is formed, repaired, and maintained by a structure, called the mantle, located at the dorsal body wall that covers the visceral body. It usually protrudes slightly beyond the visceral mass itself. All injuries or abnormal mantle conditions are usually reflected in the shell’s shape, form, and color. When the animal encounters conditions limiting its food supply, or otherwise causing it to become dormant, the mantle often ceases to produce the shell substance. When conditions improve and the mantle resumes its role, a “growth line” is produced. The mantle cavity is also a passageway for incoming feeding and respiratory currents, and an exit for waste discharge (Pojeta 1998).
The Mollusk Body
This review focuses on the shell, but a brief note on the mollusk body which produces the shell is required. The body cavity of most mollusks functions as a respiratory chamber and is usually part of the feeding structure. In many mollusks, the mantle cavity is a brood chamber used to nourish the young. Mollusks use an open circulatory system in which body fluid, called the hemolymph, is transported through the animal’s sinuses. Their organs include gills, gonads, liver, heart, muscle, stomach, and intestinal tract (Abbott 1991). Mollusks subsist on algae, zooplankton, phytoplankton, and other organic matter that passes through their filtration system. Mollusks are omnivores that consume both plants and animals, albeit in their simplest forms. They function in the ecosystem as biological vacuum cleaners that are required to reduce detritus in marine environments.
In cephalopods and some bivalves, such as scallops, the body cavity functions as a locomotor organ. The highly muscular mantle contracts, forcing water through its tubular siphon system, called the hyponome (Seed 1983). This system rapidly propels the animal through its watery environment. Gastropods use a “foot” for locomotion over the land surface even though the bivalve foot is a fleshy structure designed more for digging than for locomotion.
The Evolution of the Shell
The strong, well-preserved mollusk shells have left behind a very rich fossil record from the Cambrian to the Pleistocene (Paleontological Research Institution 2022). Many references illustrate a hypothetical phylogeny tree emanating from the single lowest common ancestor, from which all mollusks are believed by some evolutionists to have descended, namely flatworms. Rarely do evolutionists attempt to support their claims with the actual fossil(s) or other supporting evidence.
The leading conchologist, Harvard-trained former Smithsonian Director R. Tucker Abbott, totally ignored discussing mollusk evolution in his five major books on seashells (Abbott 1962; 1982; 1991; Stix, Stix, and Abbott 1968; Stix, Stix, and Abbott 1984). Evolution of mollusks from flatworms must be true, according to Darwinists, given the belief that all life supposedly evolved from simple primordial cells. Consequently, evolutionists assume this evolutionary phylogeny tree is true, hoping one day to find evidence for it. It must be true, they reason, because the assumed “ancient evolutionary history, together with the occurrence of mineralized exoskeletal hard parts in their body plan has resulted in a rich fossil record, at least of the shell-bearing taxa” (Wanninger and Wollesen 2018).
Furthermore, an estimated 100,000 described extant species and about 70,000 described fossil species now exist, providing a wealth of potential examples when attempting to locate evidence of their evolution (Parkhaev 2017, 663). In addition, mollusks are characterized by a large number of contrasting body designs, traits useful to research potential evolutionary pathways. As Wanninger and Wollesen (2015) explain,
Mollusca is the second-most speciose phylum after Hexapoda. However, what makes mollusks particularly interesting from an evolutionary perspective is not their richness in species as such, but rather the huge variety of body plan phenotypes exhibited by its representatives. These include cylindrical, shell-less, spicule-bearing, wormlike, crawling, and burrowing creatures.
The dramatic variations in overall mollusk morphology also make them “an ideal group for comparative studies into how evolution has brought about phenotypic diversity from a common ancestor that roamed the oceans’ seafloors at least 550 million years ago” (Wanninger and Wollesen 2018, 102). In spite of this advantage, the decades spent attempting to find evidence of the “hypothetical ancestral mollusk” have resulted in failure (Wanninger and Wollesen 2015, 109). Their excellent fossil record should tell us a great deal about mollusk evolution, if they had evolved. The record tells us something very different, however, namely that “no Precambrian fossilized exoskeletal elements are known that can be unambiguously assigned to an early mollusk, leaving much room for speculation as to when shell(s) and spicules first arose within the phylum and whether or not the LCAM [lowest common ancestor of mollusks] bore any armor at all” (Wanninger and Wollesen 2018, 102).
One evolutionary solution to the lack of viable transitional forms is to postulate that the source of the enormous molluscan shell diversity seen today does not support the usual story of a lowest common ancestor. Instead, cooption and de novo gene evolution very early in geological history are postulated by some evolutionists to explain the evolution of molluscan shell diversity (Aguilera, McDougall, and Degnan 2017).
Furthermore, as anyone who has spent time hunting fossils or visiting museums soon learns, molluscan fossil remains are enormously abundant in the sedimentary strata all over the world (Parkhaev 2017, 663). Although the hard shell often does not last long under normal conditions, if buried rapidly, such as in a catastrophic flood, they can be preserved for very long periods of time (Best and Kidwell 2000). An enormous number of fossil shells exist dating back to the Paleozoic era (Stix, Stix, and Abbott 1968, 5). If any transitional fossils of shells exist, by far the best place to find them would be in the molluscan fossil record. As the leading expert on mollusk evolution, Professor Pavel Parkhaev observed, “the problem of origin of the phylum Mollusca, which is ‘as old as Malacology itself’” has not been solved, even when we go
down the molluscan stem up to the Precambrian–Cambrian boundary and, therefore, to the level of appearance of skeletal organisms in the paleontological record, . . . [there is] little hope that this problem can be solved solely on the basis of paleontological data, i.e., the finds of “transitional forms” that could connect mollusks and their supposed ancestors, turbellarian flatworms. (Parkhaev 2017, 663)
Although “hypothesized evolutionary pathways of major exoskeletal and muscular subsets” have been proposed, no evidence of their evolution has been forthcoming despite their excellent fossil record that exists today (Wanninger and Wollesen 2018, 104). As no evidence of evolution from turbellarian flatworms has been forthcoming, one solution that evolutionists propose is that they evolved in the Precambrian era, and thus were fully formed in the Cambrian and have not evolved in the 540 million years since then, although many examples have become extinct. Thus lack of evidence is explained away by arguing that it occurred so far back in very early earth history that the evidence would not appear in the fossil record. The problem is that lack of evidence is not evidence.
Hochachka theorized a primitive molluscs archetype existed, a conclusion he based on the observation that “all molluscs broadly conform to the same relatively simple basic organizational plan,” noting “whether such a hypothetical form existed is of course entirely speculation” (Hochachka 1983, 2). The problem is no evidence exists of the evolution of the many different forms from this early primitive “archetype mollusc” because “all the major existing groups occur together in the earliest fossil-bearing rocks” (Hochachka 1983, 6). Because their phylogeny is unconstrained by physical evidence, other interpretations have been proposed, also based on hypothetical scenarios (Yochelson 1978). In other words, the large variety of molluscs all can be traced to the oldest fossil records and no evidence exists of their evolution from the first primitive mollusc to the variety of molluscs existing today.
Another problem for evolution is the complexity of the genetic system required to produce the shell. The reason is because “the genes that contribute to the formation of the mature shell are incredibly diverse . . . . Outside a few conserved genes, such as carbonic anhydrase, a so-called ‘biomineralisation toolkit’ has yet to be discovered” (McDougall and Degnan 2018, 1). Mollusks have also “long been used as models to study biomineralisation . . . [because] they produce an impressive diversity of shell types, are readily accessible, and have a rich fossil record allowing for evolutionary inferences” (McDougall and Degnan 2018, 2). An example of the speculation regarding the origin of biomineralization is as follows, (note the common terms “may have” and “perhaps” are used because no fossil or other direct evidence exists):
Therefore, the observed evolutionary wave of biomineralised structures may have been triggered by the increased availability of these minerals, or perhaps the overall increase in nutrient availability facilitated an acceleration of diversification in general, with expansion of biomineralised forms just one component. It is proposed that this was further driven as a defense against increased predation pressure. (McDougall and Degnan 2018, 3)
As no evidence for the evolution of biomineralization exists in the fossil record, some evolutionists propose that they evolved independently, a proposal that also lacks a fossil record. However, it would seem more likely that one structure design evolved into another structure design, as opposed to it evolving de novo 28 times, as proposed by McDougall and Degnan (2018, 3):
The biomineralised structures produced are functionally diverse and include skeletons (external and internal), teeth, spines, gravity sensing organs, eye lenses and mineral storage units. Taking into account the structures produced and the phylogenetic relationships of the organisms making them, it is likely that many are the result of independent evolutionary events, with calcium carbonate and silica biomineralization evolving at least 28 and 8 times in eukaryotes, respectively.
This position requires numerous independent evolutionary events all leading towards calcium-based biomineralized structures (McDougall and Degnan 2018, 10).
Mollusk’s Repair Ability Stymies Evolutionists
Mollusks have an amazing ability to repair their shells from a wide variety of damage, including cracks, holes, and even damage done by other animals. Martin writes, that in addition to the
normal calcification process, one aspect that made the mollusk shell a true evolutionary success lies in the ability of conchiferan mollusks to rapidly repair shell damages, an undeniable advantage for overcoming external aggressions of different sources: accidental physical shell cracks, active predation by fishes, shell boring by epibiont organisms, such as clionid sponges, or entrapment of foreign bodies between the mantle and the shell. Although the shell is a ‘dead’ non-cellular tissue, it exhibits certain plasticity, and the capacity of mollusks to partly ‘remodel’ their shell is astonishing: this property has been exploited by humankind for a long time. (Marin, Le Roy, and Marie 2012, 1111–1112)
An example of the repair ability that humans value occurs when an irritant works its way into a particular species of oyster, mussel, or clam. In order to protect itself from the irritant, the mollusk secretes a fluid to coat the irritant. Layer upon layer of coating is added until a lustrous and long-treasured pearl is formed. A review of the evolutionary theories developed to explain this repair ability of mollusks was described by a leading mollusk researcher, Martin, as “unsatisfactory” (Marin, Le Roy, and Marie 2012, 1112).
Summary
The mollusk shell provides some of the best evidence to disprove their evolution from some molluscan universal common ancestor. Some evolutionists postulate that the most likely evolutionary scenario is that mollusks evolved from turbellarian flatworms. In spite of one of the best fossil records existing today, boasting literally many millions of excellent fossils assumed to date back to the Cambrian Period, the earliest mollusks look very much like modern examples (Runnegar and Pojeta 1974). This is true even though many mollusks kinds are now extinct.
In summary “We may never know for certain what the characteristics of the ancestral mollusks might have been, and any statement concerning phylogeny and the interrelationships between present-day classes can be little more than hypothesis.” (Hochachka 1983, 48). Mollusk design is also an excellent example of irreducible complexity. In his book The Shell: Five Hundred Millions of Inspired Design the authors see the intelligent design of shells as aiding viewers to “believe in God” (Stix, Stix, and Abbott 1968, Introduction).
References
Abbott, R. Tucker. 1962. Sea Shells of the World: A Guide to the Better-Known Species. New York, New York: Golden Press.
Abbott, R. Tucker. 1982. Kingdom of the Seashell. New York, New York: Bonanza Books.
Abbott, R. Tucker. 1991. Seashells of the Northern Hemisphere. New York, New York: Gallery Books.
Aguilera, Felipe, Carmel McDougall, and Bernard M. Degnan. 2017. “Co-option and De Novo Gene Evolution Underlie Molluscan Shell Diversity.” Molecular Biology and Evolution 34, no. 4 (1 April): 779–792.
Belcher, A. M., X. H. Wu, R. J. Christensen, P. K. Hansma, G. D. Stucky, and D. E. Morse. 1996. “Control of Crystal Phase Switching and Orientation by Soluble Mollusc-Shell Proteins.” Nature 381, no. 6577 (2 May): 56–58.
Best, Mairi M. R., and Susan M. Kidwell. 2000. “Bivalve Taphonomy in Tropical Mixed Siliciclastic-Carbonate Settings: I. Environmental Variations in Shell Condition.” Paleobiology 26, no. 1 (March): 80–102.
Falini, Giuseppe, Shira Albeck, Steve Weiner, and Lia Addadi. 1996. “Control of Aragonite or Calcite Polymorphism by Mollusk Shell Macromolecules.” Science 271, no. 5245 (5 January): 67–69.
Hochachka, Peter W. ed 1983. The Mollusca: Environmental Biochemistry and Physiology. New York, New York: Academic Press.
Horne, Francis. 2006. “How Are Seashells Created? Or Any Other Shell, Such as a Snail’s or a Turtle’s?” Scientific American (23 October). https://www.scientificamerican.com/article/how-are-seashells-created/.
Jackson, Daniel J., Gert Wörheide, and Bernard M. Degnan. 2007. “Dynamic Expression of Ancient and Novel Molluscan Shell Genes During Ecological Transitions.” BMC Evolutionary Biology 7 (10 September): Article number 160. doi:10.1186/1471-2148-7-160.
Marin, Frédéric, Gilles Luquet, Benjamin Marie, and Davorin Medakovic. 2007. “Molluscan Shell Proteins: Primary Structure, Origin, and Evolution.” Current Topics in Developmental Biology 80: 209–276.
Marin, Frederic, Nathalie Le Roy, and Benjamin Marie. 2012. “The Formation and Mineralization of Mollusk Shell.” Frontiers in Bioscience 4, no. 3 (January): 1099–1125.
McDougall, Carmel, and Bernard M. Degnan. 2018. “The Evolution of Mollusc Shells.” WIRES Developmental Biology 7, no. 3 (May/June): e313. doi: 10.1002/wdev.313.
Paleontological Research Institution. 2022. https://www.digitalatlasofancientlife.org.
Parkhaev, Pavel Yu. 2017. “Origin and the Early Evolution of the Phylum Mollusca.” Paleontological Journal 51, no. 6 (17 December): 663–686.
Pojeta, John. 1998. Mollusks. The Paleontological Society. https://www.paleosoc.org/assets/docs/Mollusks.pdf.
Ramnath, B. Vijaya, J. Jeykrishnan, G. Ramakrishnan, B. Barath, E. Ejoelavendan, and P. Arun raghav. 2018. “Sea Shells And Natural Fibres Composites: A Review.” Materials Today: Proceedings 5, no. 1 (January): 1846–1851.
Runnegar, Bruce, and John Pojeta. 1974. “Molluscan Phylogeny: The Paleontological Viewpoint.” Science 186, no. 4161 (25 October): 311–317.
Seed, R. 1983. “Structural Organization, Adaptive Radiation, and Classification of Molluscs.” In Metabolic Biochemistry and Molecular Biomechanics. Edited by Peter W. Hochachka, 1–55. Cambridge, Massachusetts: Academic Press.
Stix, Hugh, Marguerite Stix, and R. Tucker Abbott. 1968. The Shell: Five Hundred Million Years of Inspired Design. New York, New York: Ballantine Books.
Stix, Hugh, Marguerite Stix, and R. Tucker Abbott. 1984. The Shell: Gift of the Sea. New York, New York: Abrams Books.
Wanninger, Andreas, and Tim Wollesen. 2015. “Mollusca.” In Evolutionary Developmental Biology of Invertebrates. Vol. 2. Edited by Andreas Wanninger, 103–153. New York, New York: Springer Publishing.
Wanninger, Andreas, and Tim Wollesen. 2018. “The Evolution of Molluscs.” Biological Reviews 94, no. 1 (February): 102–115.
Yochelson, E. L. 1978. “An Alternative Approach to the Interpretation of the Phylogeny of Ancient Mollusks.” Malacologia 17, no. 2 (17 February): 165–191.