The views expressed in this paper are those of the writer(s) and are not necessarily those of the ARJ Editor or Answers in Genesis.
Abstract
The median mass of a dinosaur is determined to be 630 kg (1389 lb), or the size of an American bison, based on the largest published and most accurate data set to date. Most dinosaurs seem to have stayed very small (0–60 kg [0–132 lb]) or grew very large (1081–56,000 kg [2383–123,459 lb]), with less species in the medium-sized range. The dinosaurs buried in the lower Flood layers (Upper Triassic series) were mostly small. Although there was an average size increase upward into younger Flood layers, it was not universal or statistically strong. There were many small dinosaurs buried throughout the entire spectrum of dinosaur-bearing rocks. The largest sauropodomorphs were buried in the Upper Jurassic series, about midway through the Flood record of dinosaurs. Whereas, ornithopods showed an increase in size from earliest to latest Flood deposits, attaining greatest size in the Upper Cretaceous series. Theropods generally showed an increase in size from the earliest deposits to the later Flood deposits, but had a small peak in size in the Middle Jurassic deposits also. The sizes of dinosaurs buried at different times in the Flood seem to have been influenced by several factors, including habitat elevation, mobility, and reaction to danger or intelligence. The larger brain size and mobility of the coelurosaur theropods may explain their prevalence in the Cretaceous system. Higher mobility of theropods in general may explain their greater than expected proportion of footprints also.
Keywords: dinosaur mass, dinosaur size, Sauropodomorph, Theropod, Marginocephalia, Ornithopod, Thyreophoran, Ornithischia
Introduction
It has often been stated in the literature that the average dinosaur was only the size of a sheep or large dog. This notion probably originated in the secular scientific literature where Horner and Lessem (1993) have claimed that most dinosaurs were smaller than bulls, and in popular literature where Crichton (1995) has stated that they were the size of sheep or a pony. Unfortunately, most of these estimates have been based on very little empirical data. It is critical that creationists base their statements on the best available data, so this contribution hopefully assists that endeavor.
There are about 1350 species of dinosaurs, with several new named each year (O’Gorman and Hone 2012). Most of these are named on incomplete specimens, so occasionally a species becomes combined with another, reducing the overall number of species. Benton (2008) estimated that the overall success rate in naming a new dinosaur species has been about 50%. A lot of this uncertainty is due to the fragmentary nature of most discoveries.
Fig. 1 shows one way to classify dinosaurs. Dinosauria are first divided into two clades (Orders) by hip style, the Ornithischia (bird-hipped) and the Saurischia (lizard-hipped). These two groups are further subdivided into five suborders based on additional skeletal similarities, the Thyreophora, the Marginocephalia, the Ornithopoda, the Sauropodomorpha and the Theropoda. Currently accepted infraorders are also shown for each suborder. The data set used in this study occasionally differentiated down to the Family level.
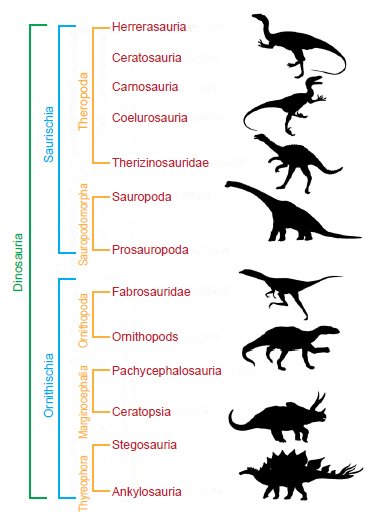
Fig. 1. Current secular classification hierarchy for dinosaurs.
Determining the exact mass of a dinosaur is something we’ll never be able to do without a living specimen. Scientists can only make estimates using skeletal data. For this reason, mass estimates for some dinosaurs have changed dramatically over the years. The earliest models and paintings portrayed dinosaurs as fat, sluggish, lethargic-looking reptiles. Most of the first reconstructions of upright dinosaurs were in the classic, kangaroo-like pose, with the tail dragging on the ground behind. More recent portrayals have made dinosaurs into leaner and more agile-moving creatures. Detailed computer analyses, footprint data, showing a lack of tail-dragging marks, and movies such as Jurassic Park have made these slimmer reconstructions mainstream.
There are three generally accepted ways to estimate dinosaur mass: 1) use of leg bone circumferences, 2) use of fleshed-out scale models, and 3) use of leg bone lengths. Some paleontologists prefer to use scale models (Lucas 2007), but there are distinct advantages and disadvantages to all methods. The beauty of the circumference method is in its simplicity. All a paleontologist needs is a couple of leg bones, which are often well preserved, in order to make a reasonable estimate of body mass, and the same is true of the leg-length method.
Anderson, Hall-Martin, and Russell (1985) developed a method to estimate mass of a quadruped based on the circumferences of the humerus and femur bones, measured at the midpoint where the bones are the thinnest. They summed the two circumferences and plotted the value against body mass on a logarithmic scale and found a straight-line relationship and corresponding best-fit equation for us to use. A similar method involving the circumference of only the femur was also developed for bipedal dinosaurs (Anderson, Hall-Martin, and Russell 1985).
The scale model method requires a more complete skeleton to begin with in order to make an estimate and may be less accurate due to a possible greater variation in the fleshed-out volume of the model (Benson et al. 2014). The leg bone length method of estimating dinosaur mass is also thought to be less reliable compared to the circumference method (Benson et al. 2014).
There have been several large studies that have tried to address the question of average dinosaur size. However, because most dinosaur specimens are incomplete and many do not possess sufficient leg bones, an average dinosaur size has been difficult to determine. An early attempt by Peczkis (1994) used 220 dinosaur genera. Peczkis determined that the mass of dinosaurs varied across six orders of magnitude with the modal mass range falling in the 1–10 tonnes (1.1–11.02 US short tons, 0.98–9.8 British long tons) category. More recent research has examined individual species instead of genera. O’Gorman and Hone (2012) used 329 species of dinosaurs, but relied heavily on less accurate methods of mass estimation, including bone lengths and scale models. Benson et al. (2014) exclusively used leg bone shaft circumference measurements of 441 species of dinosaurs and fossil birds to compile their mass estimates.
Methods
The data used in this study was taken from the species listed in Dataset S1 of Benson et al. (2014). These data include species name, suborder classification, some infraorders, a mass estimate (kg) if leg bone material was available and the level of occurrence in the rock strata. This is likely the most accurate, and comprehensive, data set of dinosaur mass estimates to date. Unfortunately the data set also included many fossil birds.
Before beginning our study, we eliminated all mass estimates that were classified as birds (Avialae) and five arguable bird-like species that were classified as theropods such as Microraptor gui. We also dropped all species that had no given mass estimate, paring down our analysis to a final total of 350 dinosaur species. This final data set included some mass estimates from different species within the same genus when leg bone material was available. We also left in 10 theropods and one sauropodomorph with multiple (two, and sometimes three) mass estimates from the same species, but determined using different specimens.
We were able to extract classification information from the published data set to place each of the dinosaurs into one of the five suborders listed in Fig. 1. Further classification into infraorders was not attempted as the numbers in some of these categories were quite small, making statistical analysis less meaningful. We also used the geologic occurrence information given for each specimen to place the dinosaurs into one of six, accepted geologic series, the Upper Triassic, the Lower Jurassic, the Middle Jurassic, the Upper Jurassic, the Lower Cretaceous, and the Upper Cretaceous, in ascending Flood depositional order. Two of the 350 dinosaur species used in this study did not have any geologic series information provided. Statistics and graphing of data were done using both MS Excel and the R statistical programming language.
Results
Basic mass trends
Table 1 shows the overall basic statistics for the dataset. Adult dinosaur masses were found to range from 0.14 kg (0.3 lb) to 56,000 kg (123,459 lb) in this data set, with a mean of 4282 kg (9440 lb) and a median of 630 kg (1389 lb). This mean is approximately equivalent to a small adult African elephant and the median mass is equivalent to an adult American bison. However, median size is probably a better measure of the average adult dinosaur size as it is not skewed as much by the high number of sauropodomorph specimens in the data base, and their extreme size, compared to the mean. Table 1 also shows that there are small dinosaurs (less than 10 kg [22 lb]) in every suborder of dinosaurs, except the Thyreophora. As expected, the median and mean are highest for the sauropodomorphs and smallest for the theropods.
Table 1. Basic mass (kg) statistics for the five major dinosaur orders.
N | Range | Mean | Median | |
---|---|---|---|---|
Sauropodomorphs | 91 | 1.2 to 56,000 | 12,640 | 9800 |
Theropods | 152 | 0.14 to 7700 | 652 | 99 |
Marginocephalia | 25 | 3 to 14,000 | 2305 | 120 |
Thyreophora | 18 | 610 to 7400 | 2858 | 2300 |
Ornithopoda | 64 | 0.73 to 17,000 | 2189 | 415 |
All dinosaurs | 350 | 0.14 to 56,000 | 4282 | 630 |
Fig. 2 shows the number of dinosaurs in various mass categories in the data set. This graph suggests adult dinosaur masses exhibit a bimodal distribution, either very light (0–60 kg [0–132 lb]) or very heavy (1081–56,000 kg [2383–123,459 lb]). The fewest dinosaurs in the data set fall in the 61–360 kg (134–794 lb) range. So, yes there were a lot of small dinosaurs, but there were even more large and extremely large dinosaurs. For some reason though, the medium-sized dinosaur was the least common. This may have to do with the ecological niches that small and large dinosaurs were able to fill, or it could be a designed survival tactic. A really small dinosaur could hide more easily, and a large dinosaur was less likely to be threatened by predators. It was likely an advantage to be one or the other. On the other side, small predators could hunt small prey and large predators could hunt larger prey, possibly explaining why there were fewer medium-sized predators also.
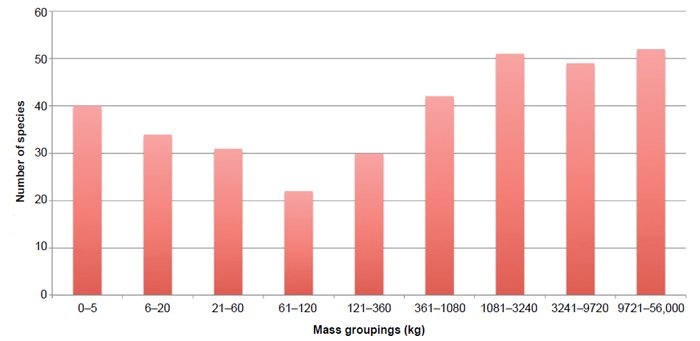
Fig. 2. Numbers of dinosaur species by mass groupings. Each successive group in the series incrementally increase by ~3×.
These data show that it is unlikely that the average dinosaur was the size of a large dog or pony as often quoted in the literature. Instead, a median-sized adult dinosaur was comparable to the mass of a modern adult bison. However, just because the median size of an adult dinosaur was fairly large, it doesn’t mean there was a room problem on Noah’s Ark during the global Flood. Most dinosaur pairs on the Ark would likely have been juveniles or young adults, not the largest and oldest dinosaurs so often seen in museum specimens. The dinosaurs taken on the Ark might have only averaged 70–100 kg (154–320 lb), or about sheep sized.
All dinosaurs went through a year or two when they grew very rapidly, a growth spurt similar to teenage humans (Erickson, Rogers, and Yerby 2001). Dinosaurs were possibly taken on the Ark about a year prior to this growth spurt, thereby needing less to eat over the course of the year-long Flood. After the Flood and their release to land, the dinosaurs would have experienced their growth spurt, rapidly maturing to adult size and sexual maturity, and therefore able to fulfill God’s plan to repopulate and fill the earth (Genesis 9:7).
Results of Dinosaur Mass by Order of Flood Burial
We also examined the data set for mass distribution patterns with order of deposition in the Flood sediments. It has been suggested that the larger dinosaurs might have survived longer as they were more mobile and able to withstand the earlier Flood events compared to smaller ones (Snelling 2014). This implies a relationship to size and timing of burial in the Flood, and that dinosaurs should become larger with time, sorting themselves upward in the Flood strata.
In order to test this hypothesis, we analyzed the total dinosaur (n = 348) distribution by stratum, and the individual suborders of the Sauropodomorphs, Theropods, and Ornithopods by their order of deposition. We did not examine the Marginocephalia (n = 25) and Thyreophora (n = 18) suborders individually by order of their burial because there were so few specimens in these categories.
Table 2 lists the means and medians for all 348 dinosaur taxa and selected suborders by geologic series or depositional sequence. In this analysis, the mean is probably the most significant number as it minimizes the large mass ranges within the dinosaur groups.
Table 2. Means in kg (median in parentheses) of dinosaurs by geological series.
Upper Triassic | Lower Jurassic | Middle Jurassic | Upper Jurassic | Lower Cretaceous | Upper Cretaceous | |
---|---|---|---|---|---|---|
Sauropodomorphs | 1015 (805) | 1,950 (460) | 12,382 (8900) | 22,508 (16,000) | 12,660 (11,500) | 13,386 (9050) |
Theropods | 53 (16) | 223 (195) | 642 (560) | 557 (72) | 551 (20) | 854 (180) |
Ornithopoda | 17 (17) | 16 (6) | 20 (7) | 301 (150) | 2386 (800) | 3093 (3200) |
All dinosaurs | 575 (30) | 1097 (260) | 6009 (1400) | 9866 (2300) | 3108 (260) | 3221 (675) |
All dinosaurs (minus Sauropodomorphs) | 46 (31) | 166 (88) | 617 (360) | 1216 (375) | 1165 (51) | 1782 (420) |
Results show that only smaller varieties of dinosaurs seem to have become buried in the lowest dinosaur-bearing strata. The mean mass for all dinosaur taxa buried in the Upper Triassic series is 575 kg (1268 lb). In the next stratum above, the mean mass of all dinosaurs goes up dramatically, with a mean of 1097 kg (2418 lb) in the Lower Jurassic series. This trend in mean mass continues to increase through the Middle Jurassic (6009 kg [13,248 lb]) and Upper Jurassic (9866 kg [21,751 lb]) series until the Lower Cretaceous, when the mean dinosaur mass drops to 3108 kg [6852 lb]. Finally, the mean mass stays nearly constant through the last of the dinosaur-bearing stratum, the Upper Cretaceous series, where the mean mass is 3221 kg (7079 lb). These data suggest that dinosaur deposition as a whole did not get systematically larger and larger as the period of the deluge progressed.
Although only small varieties of dinosaurs are found in the lowest dinosaur-bearing strata, similar small dinosaurs are found continually throughout all subsequent Flood strata, through the Upper Cretaceous series. The mean mass in each higher level stratum is merely altered by the immense sizes of dinosaurs found in Jurassic and Cretaceous strata.
We also conducted linear regression analysis of the 348 dinosaur taxa to see if there was any statistical correlation between dinosaur mass and strata. The R-value was nearly zero at −0.0097 due to the inclusion of many small dinosaurs in every stratum (Fig 3). Overall, it can be said that there is no significant correlation between dinosaur mass and strata. However, a visual inspection of the plots indicated that the sauropodomorphs were potentially masking any detectable linear trend. After their removal, the data set and regression analysis gave a positive, but low correlation of R = 0.19.
Both the theropods and the ornithopods exhibit the largest mean masses in the Upper Cretaceous strata (Table 2). In contrast, the sauropodomorphs show a peak in mean mass in the Upper Jurassic and a dramatic drop in mean mass in the final two dinosaur-bearing series. This peak was almost double the mean mass of the previous series (Middle Jurassic) and was again, nearly double the mean mass of each subsequent series (Lower and Upper Cretaceous).
Because the sauropodomorphs make up a substantial amount of the data set (n = 91), and their mass distribution pattern was completely different from the other suborders, we also calculated the mass of all other dinosaur suborders collectively, excluding the sauropodomorphs (Table 2). Results of this analysis showed a different pattern with burial compared to our earlier analysis of all the dinosaurs. The mean mass of dinosaurs failed to show a clear, systematic increase with time of burial even without the influence of the sauropodomorphs. Although the mean mass generally increases with time, there is still an observable drop in mean mass in the Lower Cretaceous series.
However, we do not just observe large dinosaurs in the last of the dinosaur-bearing strata. As shown in Fig. 3, there are many small dinosaurs in every rock layer, from the Upper Triassic through the Upper Cretaceous, complicating statistical relationships. Fig. 3 does show the larger dinosaurs getting larger with time, boosting the mean masses and contributing to the observed weak statistical significance. But this relationship is skewed by the very largest sauropodomorphs which peaked in the Upper Jurassic.
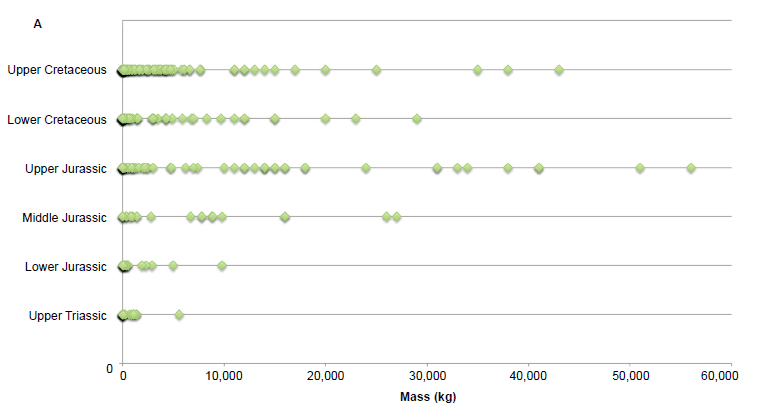
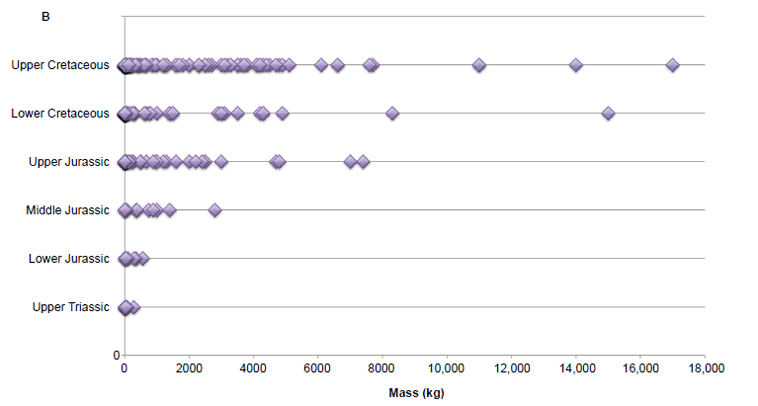
Fig. 3 (A). Plot of all individual dinosaur masses by geologic strata. (B). Plot of all individual dinosaur masses by geologic strata omitting sauropodomorphs.
Discussion and Conclusions
Where does this all lead? Are we any closer to explaining the fossil record of dinosaurs? Yes and no. Yes, we now have a fairly accurate median adult dinosaur size of 630 kg (1389 lb), based on the largest, most accurate survey of the currently available data on dinosaur taxa. This is a lot heavier than many earlier estimates of a dog or pony, and closer to that of an American bison. We would suggest that creationists should be careful not to state that the “average” dinosaur was necessarily small. There were many small dinosaurs, but just as many large dinosaurs, boosting the median size considerably.
And no, there is no simple explanation to the distribution of dinosaurs in the Flood rocks—the model is clearly multi-factor. In fact, Snelling (2014) suggested there were at least three factors that determined the burial order of organisms in the Flood: 1) their elevation of habitat, 2) their reaction to danger (and possibly intelligence), and 3) their mobility. If we assume the dinosaurs were living at about the same elevation and habitat, we can essentially eliminate the first factor, leaving us with two main variables. A fairly similar elevation of habitat is possibly why dinosaurs are found only in limited Flood strata (exclusively in the Mesozoic Erathem). Obviously, there was some variation in their habitat elevations, but overall, it may not have been as significant as the other two factors discussed below.
Dinosaur reaction to danger may be partly related to their intelligence. Dinosaurs exhibited large variations in brain-to-body ratios (Lucas 2007). Most dinosaur suborders, the sauropodomorphs, thyreophorans, and ceratopsians had relative brain sizes much less than that of a modern lizard. Whereas, the ornithopods had relative brain sizes similar to modern lizards, or slightly larger. Theropods had the largest brain-to-body ratios of any dinosaur group, with most of them possessing brains larger than a comparable-sized lizard (between 1.0–1.8 times greater; Lucas 2007). And the coelurosaurs (a subcategory of the theropods which includes the “raptors”) had by far the largest brains of any dinosaur category, with brains about 5.8 times larger than a comparable modern lizard (Lucas 2007). The reason so many small coelurosaur dinosaurs were found in higher Flood strata, like the Lower and Upper Cretaceous systems, may be because these dinosaurs were the most intelligent. They likely recognized and reacted to the dangers of the rising Floodwaters quicker and more efficiently than many other dinosaurs, and thus, survived longer before eventually succumbing to burial.
Dinosaur mobility also probably played a big role in stratification of the dinosaurs. Few large dinosaurs are found in the lowest dinosaur-bearing strata because the larger ones were able to escape more readily. And yet, the largest sauropodomorphs are found in Late Jurassic rocks, near the middle of the dinosaur-bearing strata. It is likely that the sauropodomorphs could not run as fast or sustain travel as far as many of the other larger dinosaur groups, like the ornithopods and the ceratopsians. As mentioned above, the sauropodomorphs also had the smallest brain-to-body ratios of any dinosaur suborder which probably caused them to react much differently to the impending dangers of the rising water. The large herds (numbering thousands) of ornithopods and ceratopsians that likely were very mobile and could sustain travel for great distances are also found dominantly in the uppermost dinosaur-bearing rocks of the Cretaceous system. Many of the largest theropods are found in the final dinosaur-bearing Flood strata also. It was likely their combination of size, mobility, and intelligence that allowed them to survive as long as they did. It is no surprise, therefore, to find Tyrannosaurus rex fossils near the top of Upper Cretaceous strata.
Dinosaur mobility may also explain why there appears to be an overrepresentation of theropod footprints compared to the bone record of theropods. Bone fossils of theropods make up much less than 10% of the dinosaur fossils (Lockley and Hunt 1995). Tracks at the Purgatoire site in Colorado, in Jurassic system rocks, show a 60% theropod (predator) to 40% sauropodomorph (prey) distribution (Lockley and Hunt 1995). And tracks within the Dakota Group (Cretaceous system) near Denver, Colorado at Dinosaur Ridge exhibit proportions of 25–30% predator to 70–75% prey (Lockley and Hunt 1995).
Why the discrepancy between dinosaur footprint data and the bone data? Higher activity levels of the theropods may be the solution. This makes even more sense if considered in a Flood context, however. During the Flood, the theropods may have used their higher intelligence, speed, and agility levels to disproportionately escape the advancing Floodwaters and leave more tracks, compared to the slower, and less agile, plant eaters. This may be one reason why the proportion of theropod bones does not match the proportion of theropod tracks.
It appears there are competing and complicating factors to the order of dinosaur burial in the Flood. Variables such as reaction to danger and mobility seem to have strongly influenced the burial order of some dinosaur groups, and to a lesser degree, the relative differences in habitat elevation. Although some dinosaur groups seem to follow distinct patterns, it may not be possible to sort out which factor was most dominant in every case.
It is inevitable that future finds on dinosaur bones might change our current conclusions, though we suspect our estimate will not be changed drastically.
Acknowledgments
We would like to thank Dr. Jason Lisle for his review and assistance with data and the Institute for Creation Research for their logistical support.
References
Anderson, J. F., A. Hall-Martin, and D. A. Russell. 1985. Longbone circumference and weight in mammals, birds and dinosaurs. Journal of Zoology (A) 207, no. 1:53–61.
Benson, R. B. J., N. E. Campione, M. T. Carrano, P. D. Mannion, C. Sullivan, P. Upchurch, and D. C. Evans. 2014. Rates of dinosaur body mass evolution indicate 170 million years of sustained ecological innovation on the avian stem lineage. PLOS Biology 12(5):1–11. e1001853.
Benton, M. 2008. New ways of looking at dinosaur evolution. Public lecture at Michigan State University, Natural Sciences Building, October 23, 2008.
Crichton, M. 1995. The Lost World. New York, New York: Ballantine Books.
Erickson, G. M., K. C. Rogers, and S. A. Yerby. 2001. Dinosaurian growth patterns and rapid avian growth rates. Nature 412:429–432.
Horner, J. R., and D. Lessem. 1993. The Complete T. rex. New York, New York: Simon & Schuster.
Lockley, M., and A. P. Hunt. 1995. Dinosaur Tracks and Other Fossil Footprints of the Western United States. New York, New York: Columbia University Press.
Lucas, S. G. 2007. Dinosaurs: The textbook. 5th ed. New York, New York. McGraw Hill Higher Education.
O’Gorman, E. J., and D. W. E. Hone. 2012. Body size distribution of the dinosaurs. PLOS One 7, no. 12:1–12. e51925.
Peczkis, J. 1994. Implications of body-mass estimates for dinosaurs. Journal of Vertebrate Paleontology 14, no. 4: 520–533.
Snelling, A. A. 2014. Paleontological issues: Deciphering the fossil record of the Flood and its aftermath. In Grappling with the Chronology of the Genesis Flood, ed. S. W. Boyd and A. A. Snelling, 145–185. Green Forest, Arkansas: Master Books.