Research conducted by Answers in Genesis staff scientists or sponsored by Answers in Genesis is funded solely by supporters’ donations.
Abstract
I evaluate 25 astronomical arguments for recent origin for the solar system that biblical creationists have used. Some of the arguments are found to be wanting, and I thus recommend discontinuing their use. Other arguments for young age appear to be sound. Further work on even the good young-age indicators is desirable, along with development of new arguments for recent creation.
Introduction
Contrary to popular misconception, evolution is not confined merely to biology. Rather, when discussing the physical world, evolution can be divided into three broad categories:
- Biological
- Geological
- Astronomical
One also can discuss concepts of societal evolution, such as the fields of government and law. There are evolutionary theories for how government and law came about that, as in the physical world, are in stark contrast with what the Bible has to say about these things.
Evidence of recent origin has been a major part of the argument for special creation. The reason for this is simple—it provides a discriminator between the evolution and creation models. We do not observe the sort of changes required if evolution is the explanation for origins. Therefore, if evolution is true, then it must proceed too slowly for us to see it taking place. Very briefly, the evolutionary time frame is that the universe began with the big bang 13.8 billion years ago, the solar system formed 4.5 billion years ago, life appeared on earth about 3.5 billion years ago, complex life arose in the Cambrian explosion 540 million years ago, with the arrival of man in the past million years. On the other hand, the Genesis 1 creation account reveals that God made everything in six days. When combined with biblical genealogies and chronologies, we conclude that the creation was about 6,000 years ago.
To that end, creationists have developed many evidences of recent origin. These evidences cover a wide range of topics, but they all attempt to identify things that we observe in the world that indicate ages much younger than the evolutionary worldview can account for. However, many of these arguments for recent origin do not directly indicate an age of 6,000 years. That is because many of the arguments for recent origin yield a maximum age. The actual age may be far less than the maximum, but it can be no older. If one is considering two possible explanations, such as evolution or biblical creation, evidence that eliminates one possibility leaves only the other possibility. One may argue that there may be other possibilities, and this is true. But until any other possibilities are seriously proposed, they are irrelevant to the discussion.
Many arguments for recent origin are scattered throughout the creation literature, while others are found in oral presentations. A few of the arguments for recent origin have undergone critical evaluation, but most have not. There is need of discussion and critical evaluation of all the arguments in one place. Thus far, there have been only two attempts at a broad discussion of young-age arguments. One (Ackerman 1986) was a popular-level book that discussed several young-age arguments. The other book (Rybka 1993) was a more technical treatment that treated more young-age arguments, but it was far from exhaustive.
To partially remedy this need, in this paper and a subsequent one I will discuss astronomical young-age arguments. I hope that others can tackle the other two broad areas, biological and geological young-age indicators. It would be good if all three areas, biological, geological, and astronomical, eventually could be incorporated into a single resource. For completeness, such a resource probably ought to include a discussion of biblical arguments for recent creation as well.
I have arranged the discussion of astronomical age indicators into two broad categories:
- The solar system
- The sun, stellar, galactic, and extragalactic
There are 25 age indicators in the first group and 23 in the second, for a total of 48 age indicators. I discuss the first group, the solar system, in this paper. I shall discuss the second group in a subsequent paper.
Within either group, I attempted to arrange them in some sort of logical order, such as starting with nearby objects and moving to progressively more distant objects. In many cases, there was some overlap of material. In a few cases, I combined into one age indicator age indicators that were discussed separately in the creation literature. In other cases, I thought it best to separate some age indicators into two related ones. For all age indicators, I referenced what I thought were the more definitive discussions in the creation literature and summarized their arguments. If a critical evaluation existed in the creation literature, I referenced it and briefly described its conclusions. However, in most cases no critical evaluation existed. For those age indicators, I assessed how good they were.
With a few age indicators, there was no treatment in the creation literature. Rather, they existed in oral presentations, often with little or no published documentation. I felt compelled to include discussion of them here, because one may still occasionally hear such age indicators promoted. However, most of them do not survive scrutiny, which probably is why there was no published discussion of them.
Dodwell Hypothesis
The celestial equator is the plane perpendicular to the earth’s rotation axis. The earth’s equator lies in this plane. A second plane, the ecliptic, is the plane of the earth’s orbit around the sun. The axis of the earth’s orbit is perpendicular to the ecliptic. Nearly everyone knows that the earth’s rotation axis is tilted about 23.4° to its orbital axis, and that this tilt is responsible for the seasons. Since the two axes are tilted with respect to one another, the celestial equator and ecliptic must be inclined to one another by the same angle. For this reason, astronomers prefer to call the earth’s axial tilt the obliquity of the ecliptic. While most people are aware of the earth’s axial tilt, less well known is the fact that the obliquity of the ecliptic is not constant, but rather changes very slowly over time. Astronomers 2,000 years ago were aware of this subtle change, so we have some recorded historical measurements of the obliquity of the ecliptic. Since accurate determination of when the seasons occur is important for agriculture and constructing calendars, some ancient observatories can assist us in determining what the obliquity of the ecliptic was in the past.
There are several ways to measure the obliquity of the ecliptic. One method uses a vertical gnomon. A gnomon is a post whose shadow acts as a marker. For instance, a sundial uses a gnomon to indicate the time of day. The length of the shadow cast by a vertical gnomon depends upon the height of the gnomon and the altitude1 of the sun. Let α be the altitude of the sun, let h be the height of the gnomon, and let l be the length of the gnomon’s shadow. Then tan(α)=h/l.
Clearly, tan (α) and l are inversely proportional. Therefore, on any given day, the shortest shadow will be at local noon,2 when the sun is highest in the sky. At this instant, the shadow will point due north.3 The altitude of the sun at local noon depends upon the time of year, with the highest altitude on the summer solstice and the lowest altitude on the winter solstice.4 The difference in the altitude of the sun measured on the winter and summer solstices will be twice the obliquity of the ecliptic.
A second method to measure the obliquity of the ecliptic relies upon directions defined by the rising or setting sun on the solstices. The alignment of stones in some ancient structures, such as Stonehenge, appear to be with solsticial sunrise or sunset. Alternately, some structures have orientations of passages that may align with the rising or setting of the sun on one of the solstices. For instance, this has been alleged for one of the passages in the temple at Karnak in Egypt. The azimuth of the such a passage depends upon the obliquity of the ecliptic and the latitude of the site.
The late Australian astronomer George Dodwell collected measurements of the obliquity of the ecliptic from various ancient and medieval sources (fig. 1). Additionally, he computed the obliquity from some stone structures, including Stonehenge and Karnak. All of these measurements are greater than the accepted value of the obliquity of the ecliptic today. This is not surprising, because even some ancient sources recognized that the obliquity of the ecliptic has been decreasing for millennia. Gravitational perturbations of other bodies in the solar system cause this change. Several people have modeled this change. Probably the most famous was the functional dependence of the obliquity of the ecliptic derived by Simon Newcomb a century ago. Modern functions of the obliquity of the ecliptic as a function of time deviate only minutely from Newcomb’s formula. However, Dodwell’s measurements show a marked deviation from Newcomb’s formula at early epochs, indicating a much larger value of the obliquity of the ecliptic than Newcomb’s formula.
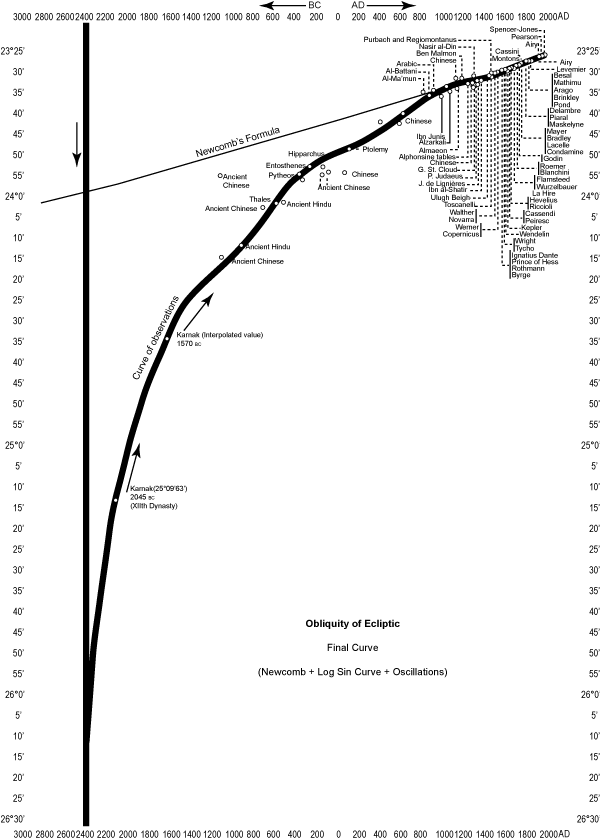
Fig. 1. Plot of Dodwell’s data versus time. (From Dodwell).
From this, Dodwell concluded that the conventional understanding of the obliquity of the ecliptic was in error. Dodwell proposed that the earth was struck by a catastrophic event that radically altered the earth’s tilt, from which the earth has only recently recovered. By fitting a curve to his measurements of the obliquity of the ecliptic, Dodwell dated this event to 2345BC±5 years, close to the biblical date of the Flood.5 Dodwell readily equated this catastrophic event with a large meteoroid impact at the time of the Flood, an impact that probably acted as a trigger for the Flood. Of course, this proposal proved to be popular with creationists (Ackerman 1986, 88–96; Setterfield 1983; Wieland 1983). Furthermore, Dodwell believed that the earth’s tilt was nearly 0° prior to the Flood, an idea that remains popular with many creationists. Unfortunately, Dodwell died in 1963, leaving an unpublished manuscript of his work. For decades, it was hoped that his work could be published, but it never was. The manuscript, with a few editorial comments clearly marked, became available online just a few years ago (Setterfield 2010). It is important to note that from one of his comments, it is very clear that Setterfield believes the pre-Flood obliquity of the ecliptic was greater than today.
The only critical analysis of the Dodwell hypothesis is that of Faulkner (2013). That analysis discussed the various factors that affected the measurements of the obliquity of the ecliptic, along with likely errors of those measurements. The key datum supporting the Dodwell hypothesis is the earliest point from Karnak (see fig. 1). This point comes from the assumption that there was an alignment with the rising sun on the summer solstice. However, there are good reasons for this not to have been the case. If that one point is removed, much of the argument for the Dodwell hypothesis is removed. On the other hand, there is considerable evidence for such an alignment at Stonehenge. Interestingly, Dodwell did not plot the obliquity of the ecliptic deduced from Stonehenge, preferring instead to use it as a test of his hypothesis. This is ironic, because since Dodwell did his work, the date of the early construction of Stonehenge has been reevaluated (in a manner that had nothing to do with solsticial alignment). With the corrected earlier date, the Stonehenge alignment disproves the Dodwell hypothesis.
From this analysis, it is not at all clear that there was a catastrophic event that altered the obliquity of the ecliptic. Therefore, the Dodwell hypothesis is not a viable evidence for recent origin/catastrophe.
Lack of Meteorites in Geologic Strata
Many tens of thousands of meteorites have been recovered from the surface of the earth. The total mass of recovered meteorites must be hundreds of tons. Because much of Antarctica receives little annual precipitation, meteorite collection there is relatively easy, as any meteorites that have fallen on the ice remain atop the ice. As of 2016, the US Antarctic Meteorite program had collected 22,000 meteorites.6 All the meteorites recovered on the earth’s surface must have fallen within the past few centuries, or, at most, in the past few millennia. Consequently, if sedimentary rock layers gradually formed over hundreds of millions of years, then meteorites ought to be common in sedimentary rocks. However, if most sedimentary rock layers were laid down quickly during the Flood, then we would expect to find very few meteorites in their strata. Creationists have argued that meteorites are so rare in sedimentary rocks as to be non-existent (Ackerman 1986, 27–28; Armstrong 1978; Rybka, 1993, 46–47; Steveson 1975), conforming to the expectation of recent creation and a catastrophic flood.
But is this good evidence of recent origin? In the creation literature, Snelling (2012) has reported on the discovery of a meteorite shower field in Ordovician limestone. There have been similar reports of meteorites found in other strata. Meteorites can decompose, and they may dissolve to some degree from water percolating through permeable rock layers. Since meteoroid impacts may have contributed to the initiation of the Flood (Faulkner, 1999; Spencer 1998, 1999), creationists may expect that there be some meteorites in the geologic column, as within the evolutionary model. The question is, given the introduction and destruction rates, what density of meteorites would one expect within either model? This is poorly known on both counts. Therefore, until creationists further develop this, it is not a good indicator of recent origin.
Lunar Dust
For a long time, lunar dust has been an argument for young age used by recent creationists (Ackerman 1986, 15–23; Boardman, Koontz, and Morris 1973, 150–152; Brown 2008, 39, 418–420; DeYoung 2010, 23–24; DeYoung and Whitcomb 2003, pp. 63-64; DeYoung and Whitcomb 2010, 41; Morris 1985, 151–153; Rybka 1993, 50–53; Slusher 1980, 41–42; Whitcomb and DeYoung 1978, 94–95). Studies in the late 1950s measured the influx of meteoritic material on the earth. Assuming the moon was subjected to a similar influx for billions of years, it appeared that the moon might be covered with an extremely deep layer of fine dust. There was some concern that spacecraft sent to the moon would sink into this dust. Supposedly, this is the reason the Lunar Excursion Module (LEM) that Apollo astronauts used to land on the moon had such large landing pads on each of its four legs. However, in 1966–1968, five Surveyor spacecraft softly landed on the moon. The video cameras and scoops on the Surveyors indicated at most a very thin layer of dust on the lunar surface. This was confirmed by the six Apollo landings (fig. 2). Creationists were emboldened by the failure of the prediction based upon billions of years, and understandably used the thin layer of dust on the moon as an argument that the moon was far younger than billions of years old.
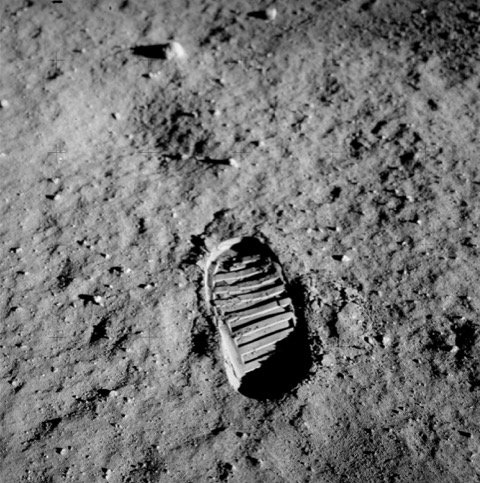
Fig. 2. Footprint of Apollo 11 astronauts Buzz Aldrin’s boot, showing a thin layer of fine dust on the moon.
However, the early measurement of meteoritic influx was indirect, and it turned out to be orders of magnitude too high. By the 1970s, direct measurements of the meteoritic influx were available, but those attracted little attention among recent creationists. Finally, in the early 1990s, references to the newer measurements began to appear in the creation literature (Kuban 1991; Rybka 1993, 50–53). Snelling and Rush (1993) did a comprehensive examination of the data. Their conclusion was that the newer, direct measurements of meteoritic influx were consistent with a moon that is billions of years old, so they recommended against using this argument for recent origin.
Lunar Ghost Craters
We can employ several principles to determine relative ages of lunar features. One principle is stratigraphy: if one feature appears to be on top of another feature, we safely infer that the feature on top is younger than the feature underneath. Consider craters. Most lunar craters appear to be the result of impacts. Where two craters overlap, one crater clearly is on top of the other crater. We say that the more recent crater has modified the older crater. See fig. 3 for examples of overlap of craters. A second principle of determining relative ages of lunar craters is to examine their morphology. Craters are subject to various erosion processes, the most pronounced being churning by additional impacts. When a crater first forms, it appears very sharp, but over time erosion softens that appearance. Therefore, there is an inverse relationship between relative ages of craters and the sharpness of their morphology. See fig. 4 for an example of difference in morphology of craters. A third principle for determining the relative ages applies to lunar regions: some regions have higher crater density, while other regions have lower crater density. Presumably, impacts on the moon are randomly distributed over large areas, so if two regions display different crater densities, it probably is because the region with lower crater density is younger. Therefore, there is an inverse relation between crater density and age.
Let us apply this third principle to the two types of lunar topographies, the maria and the highlands (fig. 5). As the name suggests, the highlands are at higher elevation than the maria. Even the name maria (Latin for “seas”) suggests that they are at lower elevation, but that is not why the maria are named so. The maria are the darker regions on the moon, while the highlands are the lighter regions (to the naked eye, these disparate regions give the impression of the “man in the moon” when the moon is full). The highlands are saturated with craters, but the maria have very low crater density, making them appear relatively smooth. When astronomers first examined the moon with telescopes four centuries ago, they thought that the relatively smooth, dark maria might have been bodies of water, hence the name. Since the maria crater density is far less than that of the highlands, we conclude that the maria must be younger than the highlands. The morphology of craters in the respective regions is consistent with this conclusion. The morphology of highland craters ranges from very sharp (young) to very rounded (old), but maria craters have sharp morphology, suggesting that they are relatively younger than most highland craters.
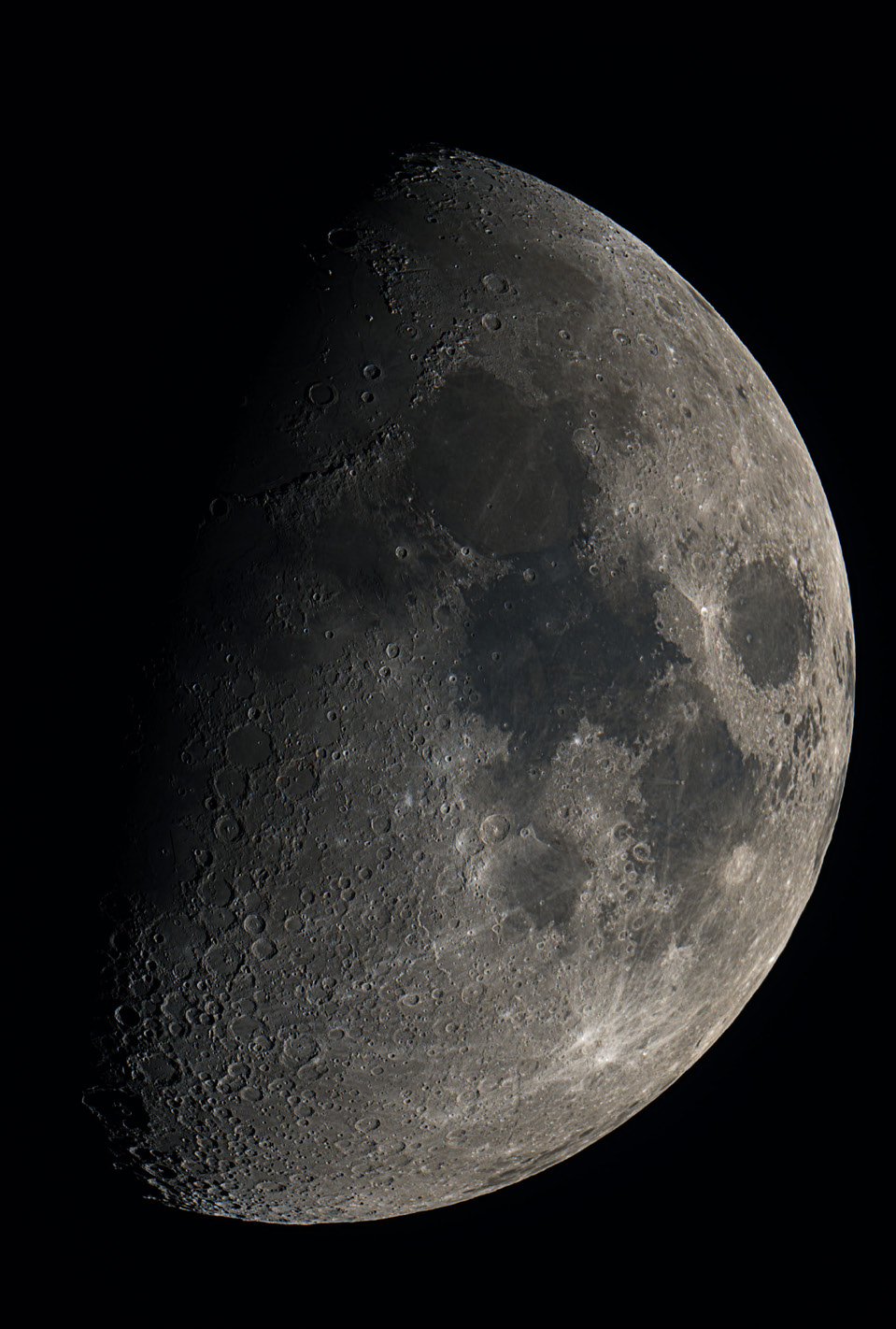
Fig. 3. Notice many examples overlap of craters in the lower (southern) part of the moon. Where a crater clearly sits atop another crater, the principle of stratigraphy establishes that the crater sitting atop is younger than the crater underneath. We say that the younger crater has modified the older crater. (Photo credit: Jim Bonser).
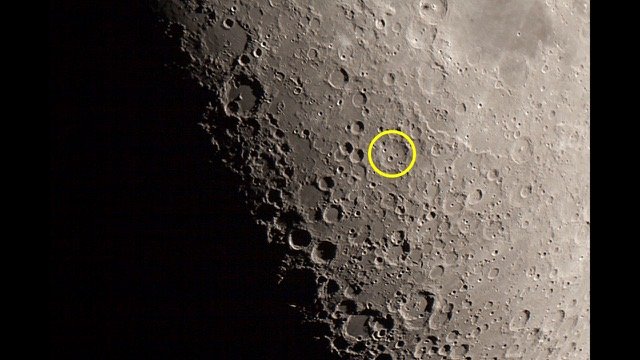
Fig. 2. Notice the morphology of the large crater within the circle. The crater is very indistinct, indicating that it has undergone much erosion since it formed. Contrast the morphology of the large crater to the smaller craters that have modified the large crater. They appear much sharper, indicating relatively little erosion since they formed. Thus, the smaller craters are deemed to be younger. Additionally, stratigraphy indicates that the smaller craters are younger too. (Photo credit: Jim Bonser).
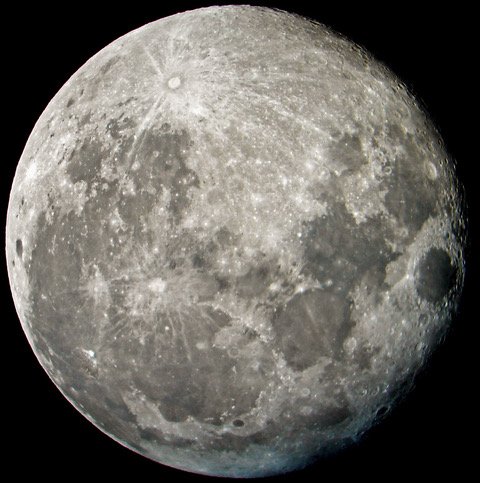
Fig. 2. A nearly full moon illustrating the difference between the lunar highlands and maria. The highlands are the lighter, heavily cratered terrain, while the maria are the darker, smoother terrain. Notice that the maria have roughly circular shapes (Photo credit: David Rives).
We may use this conclusion, along with a few other bits of information, to infer a lunar history. Presumably, the entire lunar surface was exposed to intense bombardment early in its history, leaving the entire surface of the moon heavily cratered. Secular astronomers call this event the Early Heavy Bombardment (EHB). At some point, volcanic eruptions introduced lava that flowed over portions of the moon that covered, or modified, the lunar surface in those regions. The lava cooled and hardened, leaving a relatively smooth surface on which further impacts were preserved as more recent craters. Why is there a color difference between the unmodified (highlands) and modified (maria) regions on the moon? The primordial rocks on the moon likely resembled terrestrial granites, while the volcanic flows probably more closely resembled terrestrial basalts. This conclusion was reached more than a half-century ago but was confirmed by chemical analysis of samples returned to earth by the Apollo astronauts. The difference in color between granite and basalt accounts for the albedo difference between the highlands and maria. Furthermore, the establishment of hydrostatic equilibrium between the different densities of granite and basalt explains the altitude difference between the highlands and maria.
One more clue helps establish a lunar history—the maria tend be circular or overlapping circles. This gives them the appearance of being very large craters. Planetary scientists propose that after the EHB, that there was a brief episode of very large impacts, an event called the Late Heavy Bombardment (LHB), after which the rate of impacts greatly reduced. The LHB resulted in very large craters called impact basins, large circular depressions, on the moon. The energy released by the large impacts fractured the moon deeply enough to reach molten material within the moon. The fractures acted as conduits to permit magma to reach the lunar surface, filling, and in some cases overflowing, the impact basins to create the maria. Notice that this history establishes relative ages, not absolute ages, of lunar features. Absolute ages must be determined by other means. Faulkner (1999, 2000, 2014a) and Samec (2008a, 2008b) have proposed that recent creationists can adapt this inferred lunar history within a biblical timeline. This proposal associates the EHB with the formation of the moon and other astronomical bodies on Day Four and the LHB with a catastrophic event at the time of the Flood
Faulkner (1998; 2017, 119–120) and Samec (2008a) have suggested that ghost craters imply a recent origin for the moon. Ghost craters (fig. 6) appear as faint circular outlines in the otherwise smooth looking maria, with small rugged segments of their walls occasionally protruding. All lunar maria contain at least some ghost craters. From their appearance, it is obvious that ghost craters were modified by the volcanic overflow that formed the maria. Therefore, these craters must have predated the volcanic overflow. However, the ghost craters in each mare must have postdated the formation of the mare’s impact basin, or otherwise the impact would have obliterated the craters. If we believe the volcanic overflow was triggered by the fracturing resulting from the formation of the impact basin, then one logically would conclude that the volcanic overflow rapidly followed the creation of the impact basin. This would seem to establish a rather short timescale between the volcanic impact and subsequent volcanic overflow. Most astronomers assign a time-varying cratering rate based upon the supposed 4.5-billion-year age of the moon and solar system. However, the existence of ghost craters directly challenges this rate, because the inferred cratering rate is far too low to account for the density of ghost craters in the maria. The solution has been to allow for hundreds of millions of years between the creation of impact basins and the subsequent volcanic overflow. The time delay between the two events for each mare is fixed by the density of ghost craters observed in each mare.
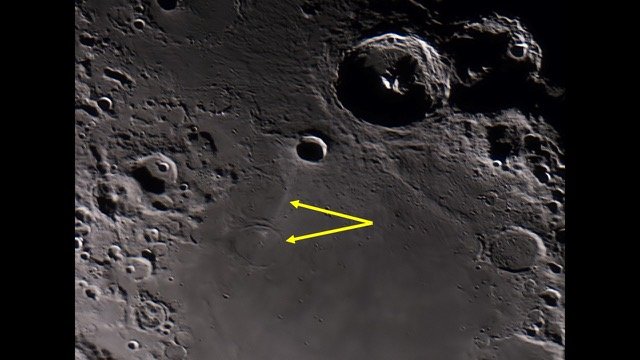
Fig. 6. The arrows point to two ghost craters. Notice that while the circular shapes of the craters persist, nearly all other crater features appear to have been flooded. (Photo credit: Glen Fountain).
However, if the world is only thousands of years old, then the relatively high crater density on the lunar surface requires that the cratering rate in the past must have been many orders of magnitude higher than is inferred from the assumption of an age that is billions of years old. A much higher cratering rate in the past would account for the many ghost craters that exist in the lunar maria. Therefore, this is a good argument for recent origin.
Rock Flow on the Moon
Morton, Slusher, and Mandock (1983) claimed that the viscosity of lunar rocks would cause crater walls to flow downward, eliminating impact craters on the moon on a timescale of thousands, or, at most, millions of years. Thus, they argued, lunar craters cannot be billions of years old, as is generally thought. Ackerman (1986, 51–53) and Brown (2008, 39), repeated this claim. However, there are problems with this analysis. Early in their paper, Morton, Slusher, and Mandock mentioned the allto-commonly believed misconception that glass flows at temperatures that we normally encounter. They repeated the frequently cited example of old glass panes being noticeably thicker at their bottoms. This is not true, as glass does not flow except at very high temperatures (Brill 2011). Slusher, Mandock, and Morton went on to list viscosities of various rocks and other substances, though they did not reference many of them. Rock viscosities are very high, and hence they are difficult to measure, except at very high temperatures, and the measurements generally are not from direct laboratory measurements. From the table of viscosities in Morton, Slusher, and Mandock, it is immediately clear that something is amiss, for the earth’s mantle is listed as having viscosity of 1022 poise, while other rocks, such as granite (1021 poise) and limestone (1020 poise), have lower viscosities. When one considers the considerably higher temperature and pressure in the mantle, this makes no sense. The authors must have misinterpreted data, because their study was based upon estimates of viscosity that are orders of magnitude too low. Therefore, there is no basis for arguing that rock flow would reduce lunar craters on short timescales.
Transient Lunar Phenomena
Most planetary scientists think that the moon formed 4.5 billion years ago along with the rest of the solar system. In this view, most lunar craters are the result of impacts during the first half-billion years of the moon’s history, with relatively few impacts since. The moon’s surface shows no clear evidence of ongoing geological activity. Given its modest size, the moon’s primordial heat would have dissipated long ago. Its low density suggests that the moon lacks the long-lived radioactive isotopes that could heat the moon’s interior sufficiently to produce geological activity. Therefore, in the evolutionary paradigm, the moon has been geologically dead for a long time. However, there are numerous reports of transient lunar phenomena (TLP, though some authors prefer LTP) on the lunar surface going back at least two centuries. A TLP is a brief emission of light, change in color, or the appearance of a cloud in a localized area of the moon. The most obvious interpretation is that TLPs are volcanic activity on the moon. This possible explanation kindled interest in TLPs during the 1960s over concern of the safety of Apollo astronauts landing on the moon. This prompted NASA to commission Project Moon-Blink, a system of large amateur telescopes equipped with high-speed cameras to capture images of regions of the moon most prone to TLPs. The Johnson Telescope at Johnson Observatory at the Creation Museum in northern Kentucky was part of Project Moon-Blink.
If the moon is geologically active, then that would be of great interest to creationists. Whitcomb and DeYoung (1978, 105–127) devoted an entire chapter to TLPs, and it remains the most complete discussion of TLPs in the creation science literature, though there are others (DeYoung 2003; DeYoung and Whitcomb 2003, 66–67; DeYoung and Whitcomb 2010, 43–44; Henry 2006c; Rybka 1993, 54–55). How has the general scientific community responded to TLPs? Once the notion of an ancient, geologically inactive moon became entrenched in the early twentieth century, few scientists paid much attention to TLPs. Thus, most planetary scientists dismiss TLPs as anomalies that are best explained by poor seeing or other effects, such as sunlight briefly glinting off lunar features. However, a few astronomers remain very open-minded about the question. For instance, see the discussions of Cameron (1972, 1991). Crotts (2009) argued that the statistics of numerous TLPs associated with particular lunar locations makes it unlikely that all TLPs can be explained by anomalies of seeing. Robinson (1991) listed 11 possible causes of TLPs, apart from seeing effects. In recent years, astronomers have detected meteoroid impacts on the moon. However, while impacts may explain some TLPs, it is unlikely they are sufficient to explain most TLPs.
If TLPs are real and indicate geological activity on the moon, then TLPs are difficult to explain on the evolutionary timescale. However, if the moon is very young, geological activity is possible on the moon. Therefore, TLPs could offer good evidence for recent lunar origin. This subject warrants further study. With recent rapid improvements in camera and software technology, it is now feasible to monitor the moon almost continuously. Consequently, interest in TLPs appears to be rising, with several modest programs devoted to monitoring the moon for TLPs on a regular basis. This could be a productive area of original research by creationists. However, until more definitive conclusions can be reached, I advise caution in this matter.
Lunar Recession
Gravitational force varies with the inverse square of the distance. However, tidal force is the differential force of gravity, so it varies by the inverse cube of the distance. Consequently, while the sun’s gravitational force on the earth is greater than the moon’s (owing to its much greater mass), the moon’s tidal force is greater than the sun’s tidal force. Therefore, tides raised by the moon dominate over tides raised by the sun. The moon’s tidal force causes a high tide on either side of the earth, with low tides in between. The tidal bulges (high tides) on either side of the earth ought to align with the moon. However, the earth’s rapid rotation carries the two tidal bulges forward so that at most locations, high tides occur before the moon reaches the meridian.
This advancement of the tidal bulges from the line connecting the centers of the earth and moon leads to a complex long-term interaction usually referred to as tidal evolution. The advancement introduces an axis that acts as a handle for the moon’s gravity (note that it’s gravity, not tidal force) to pull on. The moon pulls both tidal bulges toward itself, but since the earth’s center is between the two tidal bulges, the moon’s gravity on either tidal bulge produces opposite torques on the earth. Since one tidal bulge is closer to the moon, the torques are not equal. Taking the direction of the earth’s rotation as positive, the larger torque is negative, while the lesser torque is positive. The net result is a negative torque, leading to a slowing rotation for the earth. Hence, the length of the day slowly is increasing. At the same time, Newton’s third law of motion requires that the tidal bulges act on the moon with an equal but opposite force. This accelerates the moon forward in its orbit, causing the moon slowly to spiral away from the earth.
While it is relatively straightforward application of physics to understand this process, we cannot model it, because the exact value of the tidal evolution of the earth-moon system depends critically upon the distribution of the continents and the continental shelves, and details of how tidal flow interacts with landmasses. Furthermore, there is an interaction within rocks in the upper mantle and crust, because, in addition to water tides, there are tides raised in the earth itself. However, we can measure the rate at which the earth’s rotation is slowing and the moon is receding from the earth. The former is deduced from historical total solar eclipse records. We can compute precisely the tracks of past total solar eclipses if tidal evolution has not occurred. Comparison of those calculated tracks with observed paths determines the accumulated slowing in the earth’s rotation since the epoch of each eclipse. Studies show that the earth’s rotation is slowing by about 0.0016 of a second per century. The rate of lunar recession is fixed by reflecting laser lights off mirrors that Apollo astronauts left on the lunar surface. Half the transit time gives the distance between the observatory and the lunar reflectors. The result is not as straightforward as that, because there are several other factors that continually perturb the moon’s orbit. However, all those terms are well understood, so when they are removed, the residual is the lunar recession. The best value for lunar recession is about 4 cm/year.
There are at least two common misconceptions one must avoid in discussing the tidal evolution of the earth-moon system. One misconception is to assume that the leap second that is added to clocks worldwide at roughly 18-month intervals is due to this lengthening of the day. However, there is a second factor that is changing the length of the day. The tidal evolution portion is called the secular change, and it always acts to increase the length of the day.7 The other factor is called the periodic change, because it alternates between increasing and decreasing the length of the day. Currently, the periodic change has the same sign as the secular change, and its amplitude is greater than the secular change, so the leap seconds occasionally added to our time standards primarily are due to the periodic, not the secular, change.
The other misconception is to assume the rate of lunar recession is linear. With this assumption, over 4.5 billion years the moon would have receded approximately 170,000km. The moon’s current average distance from the earth is nearly 400,000km. So, if the earth-moon system were 4.5 billion years old, then the moon’s distance at the beginning would have been about half its current distance. However, rather than being linear, the force driving tidal evolution goes as the inverse sixth-power of the distance. This is a very steep function of distance. This means that in the past, when the moon was closer to the earth, the rate of lunar recession was much higher. Therefore, assuming a linear function will work only over the short term. Barnes (1974, 1982) was the first to discuss the recession of the moon as an indication of recent origin in the creation literature. DeYoung (1990) plotted the moon’s orbital radius as a function of time based upon the inverse sixth-power dependence of the tidal evolution. The plot showed a nearly linear trend over the past billion years. However, at earlier time, the plot makes a drastic plunge toward zero. To quantify this, DeYoung solved the inverse sixth-power of distance differential equation, treating the current measured rate of lunar recession as a boundary condition. His solution showed that the earth and moon would have had zero separation 1.37 billion years ago. Apparently unaware of DeYoung’s work, Rybka (1993, 42–45) assumed to a constant rate of tidal evolution to obtain a flawed result. More recently, Henry (2006b) reached a similar conclusion to DeYoung. Of course, working backward in time, contact would have occurred at a time slightly less than this, with tidal distortion resulting in disruption of the moon at an even slightly earlier epoch. However, with the steep functional dependence of time, it is no matter, because to a good approximation, all these catastrophic events would have been about 1.4 billion years ago. Furthermore, catastrophic tides a mile high would have prevailed approximately a billion years ago. No one believes such tides ever existed. With these considerations, lunar recession sets an upper limit to the age of the earth-moon system that is far less than the generally assumed 4.5-billion-year age for the earth and moon.
I must stress several important points. First, recent creationists do not claim that the tidal evolution of the earth-moon system directly proves that the earth is thousands of years old. Rather, we point out that it demonstrates an upper limit of 1.4 billion years, about 30% of the generally assumed age of 4.5 billion years. That is, while the expected tidal evolution of the earth-moon system is consistent with the recent creation model, it is incompatible with an age of 4.5 billion years (Rybka 1993, 56–57). Second, while simple, DeYoung’s analysis is basically correct. A few decades before DeYoung’s paper, several noncreationists had made the same calculation, reached a similar upper limit to the age of the earth-moon system, and were asking how this could be, if the earth and moon were 4.5 billion years old. Third, numerous proposals to explain this problem have appeared in the secular literature. Most of these proposals suggest that we happen to be living at a time of unusually high tidal evolution. However, how likely is that, particularly when other studies suggest that the rate of lunar recession has been nearly constant for 900 million years (Faulkner 1998)? Furthermore, Lisle (2013) has pointed out that these solutions violate the assumptions of uniformitarianism and naturalism, without which there is no reason to believe the in an old age for the earth and moon to begin with. Psarris (2010) has pointed out that this is a good argument for recent creationists to use, but he stressed the need in the creation literature for a thorough discussion of the various solutions evolutionists have proposed to solve this problem for them.
Mercury’s Atmosphere
The planet Mercury has a very tenuous atmosphere. According to Pollack (1981), surface pressure on Mercury is approximately 10-15 bar, and the atmosphere is 98% helium and 2% hydrogen. Rybka (1993, 62–64) argued that, based upon the relatively weak surface gravity and high temperature due to its proximity to the sun, any atmosphere that Mercury had must rapidly dissipate. Therefore, he concluded, even the very thin atmosphere that Mercury possesses must mean that Mercury is young. Rybka offered no quantitative assessment. He also cautioned that we need await further data, but opined that in the interim, this is a good argument for recent origin.
Rybka’s argument was based upon data from the Mariner 10 flybys of Mercury in 1974–1975. Later data eventually arrived with the much more robust Mercury Messenger mission that orbited the planet between 2011 and 2015. According to Williams (2018) the Mercury’s surface atmospheric pressure is approximately 5×10-15 bar, a result consistent with, but an improvement upon, the Mariner data. However, the earlier composition estimate was completely wrong. Table 1 gives the number density per 106 particles per cm2 for Mercury’s atmosphere.
Element |
Abundance |
---|---|
Na |
12,000-200,000 |
Mg |
100,000 |
O |
< 40,000 |
H |
5,000 |
K |
800-1,300 |
Ca |
300-1,000 |
Fe |
< 300 |
Al |
15 |
In addition, there are trace amounts of Ar, CO2, H2O, N2, Xi, Kr, Ne, and He. The total mass of the atmosphere is estimated to be approximately 10,000kg. To put this in perspective, at pressure of one bar, the earth’s atmosphere contains this much mass in 8,000m3, the volume of a cube 20m on a side. The nuclei of most of these elements are very heavy compared to hydrogen and helium, so it is plausible that they may represent a permanent atmosphere around Mercury. Obviously, the amount of hydrogen and helium is far less than earlier estimates, so it also is plausible that the two lightest elements may be in steady state from the solar wind particles temporarily captured by Mercury’s gravity (that was the original explanation, when the atmosphere was thought to contain far more hydrogen and helium).
At any rate, it does not appear that this is a good argument for recent origin.
Tall Mountains on Venus
Brown (2008, 29) has called attention to tall, dense mountains on Venus that appear to be out of hydrostatic equilibrium. As on earth, the surface of Venus floats atop the upper mantle, a plastic layer deeper inside the planet. Any tall, relatively dense surface features are out of hydrostatic equilibrium, and so they ought to sink into the upper mantle to reestablish hydrostatic equilibrium. This process takes some time, but its timescale is short compared to the billions of years assumed to be the age of Venus. Brown specifically mentioned Maat Mons, a 9km tall shield volcano and second-highest mountain on Venus (fig. 7). However, Mouginis-Mark (2016) has shown that there is good evidence of recent volcanic activity on Maat Mons. Furthermore, another paper (Robinson, Thornhill, and Parfitt 1995) suggested volcanic activity on Maat Mons could explain variations in the sulfur dioxide and methane content of the venereal atmosphere. Therefore, it is very likely that this mountain is very young, but that does not require that the entire planet be young.
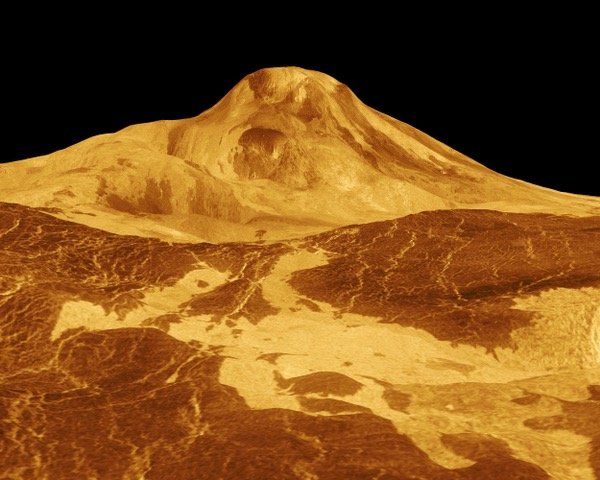
Fig. 7. A false-color image of Maat Mons. The vertical scale is exaggerated by a factor of 22.5.
With these considerations, the height of Maat Mons is not a good argument for the recent origin other than the mountain itself. However, further study of other mountains on Venus might be productive.
High Winds on Venus
Space probes to Venus have revealed that there are very fast winds in its upper atmosphere. The wind speeds are approximately 100m/s, comparable to jet stream speeds in the earth’s upper atmosphere. However, unlike earth, Venus rotates on its axis very slowly, taking 243 days to complete one rotation. Since it takes these high-altitude winds only four (earth) days to circle Venus, they are called superrotational. The earth’s jet stream is not superrotational, because they similarly take four days to circle the planet, because their speed is only one-quarter the earth’s rotation period.
Rybka (1993, 65–66) argued that Venus’ superrotational winds indicate that the planet is young. He reasoned that since the high altitude venereal winds are opposite to the direction of Venus’ rotation, friction with the surface of Venus and internal viscosity in its atmosphere should have long since died down. But this raises two problems. First, granting that the winds oppose Venus’ rotation, what is the timescale on which the winds would dampen? Rybka offered no calculation of this, but the timescale likely is far shorter than 6,000 years. If so, then this would be a problem even within the recent creation model. The second problem is that Rybka apparently misinterpreted the wind direction on Venus. While Venus’ winds are described as retrograde (Markiewicz 2007), this term has a specific meaning within the solar system. Retrograde motion is defined as orbital or rotational motion that is opposite to the direction (prograde) that most solar system bodies rotate or revolve. The prograde direction is CCW as viewed from above the earth’s North Pole, while retrograde is CW as viewed from above the earth’s North Pole. Venus is one of two planets with retrograde rotation (Uranus is the other). Therefore, the winds on Venus are in the same direction as its rotation. Hence, it does not appear that this is a good argument for recent origin.
Dust Storms on Mars
Rybka (1993, 67) suggested that dust storms on Mars might indicate that Mars is young, but he did not explain his reasoning. However, he acknowledged that differential heating on Mars could explain this. Indeed, differential heating is the explanation for all atmospheric winds in the atmospheres of planets. Therefore, this is not a good argument for recent origin.
Phobos (and Deimos)
Phobos is the innermost of two small satellites that orbit Mars (fig. 8). Tidal forces raised by Mars are robbing Phobos of orbital energy, so its orbit is decaying. Rybka (1993, 84) reported a maximum lifetime remaining of 60 million years, though he gave no reference for this. Burns (1978) determined a range of 30–50 million years, though Bills et al. (2005) concluded that this is a maximum limit. However, these considerations do not reveal how long Phobos could have orbited Mars in the past. When farther from Mars, the tidal dissipation was less, so the time from the earliest epoch to now could have been considerable. And even if that maximum age exceeds the supposed 4.5-billion-year age of the solar system, the scenario for Phobos’ origin is that it is an asteroid that Mars’ gravity captured. Therefore, at first glance, this might not to be a good argument for recent origin.
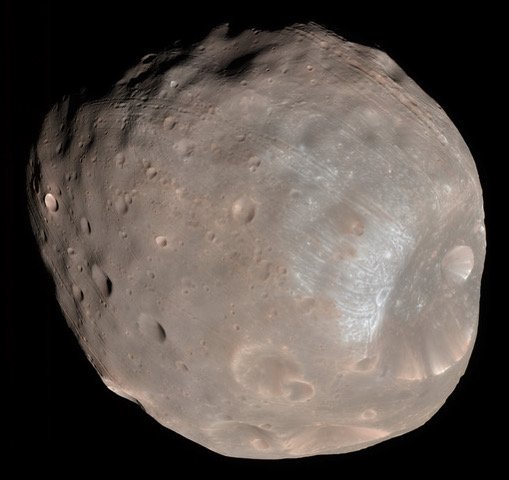
Fig. 8. Phobos, the larger satellite of Mars.
However, there is more to the story. The reason the orbit of Phobos is decaying is that its orbital period is shorter than the rotation period of Mars. On the other hand, Deimos, the other, smaller satellite of Mars has an orbital period that exceeds Mars’ rotation period. Consequently, Deimos is spiraling away from Mars, much as the earth’s moon is receding from earth. How long this will take is uncertain. The orbital period of Deimos is less than six hours longer than the rotation period of Mars. Since synchronous rotation is the break-even point for tidal evolution, with satellites with shorter orbital period infalling and those satellites with longer orbital periods receding, one must ask how long Phobos and Deimos have been in their current states. Deimos could not have been receding for a very long time, for this would have placed Deimos’ orbital period less than the rotation period Mars in the not-too-distant past. And Phobos’ orbit could not have been shrinking earlier than the time that Phobos’ orbital period equaled the rotation period of Mars. This would seem to impose an upper limit for the age much less than billions of years.
The usual response is that two satellites were asteroids that Mars recently captured. Capture events typically result in highly inclined, very elliptical orbits. But both Phobos and Deimos have nearly circular, low inclination orbits. There are mechanisms that can circularize and lower inclinations of orbits, but these mechanisms take considerable time and don’t appear to be applicable to the Martian system. So, if Phobos and Deimos are captured asteroids, why are their orbits this way (Faulkner 2014b)? The issue of the orbits of Phobos and Deimos may provide a good argument for recent origin after all. This warrants further study.
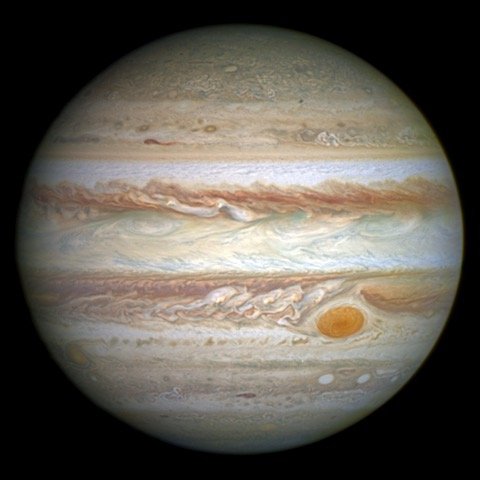
Fig. 9. Jupiter. The banded structure, as well as the various spots and swirls are a result of strong winds in the upper atmosphere of Jupiter.
Rybka (1993, 68–69, 72–73) has proposed that these large winds are the result of the planets’ recent creation and hence amount to evidence of their recent origin. Rybka associated the high winds with the internal heat that these planets have (the next topic to be discussed) and suggested that both planets were created with high winds and internal heat. He further reasoned that the winds eventually would die down, which implies that these two planets are young. However, the association of the winds with an internal heat source may be the key in understanding what is going on. As previously mentioned, winds generally are the result of differential heating. The atmospheres of these two planets differ from the earth in that not only do they receive heat from the sun, but they also receive significant heat from the interiors of the planets. Therefore, as long as the atmospheres of these two planets receive significant heat from their interiors, the winds are likely to persist. Furthermore, there are two other ways in which these planets fundamentally differ from the earth. First, they lack solid surfaces that can support pressure differences at the same depth as the earth does. Second, with their much larger size and faster rotation rates, the Coriolis effect in their atmospheres is much stronger than on earth (the rotation rate on Jupiter’s equator is equal to the escape velocity of the earth). These factors are believed to be the reason there is a banding structure with high relative wind speeds in the atmospheres of the Jovian planets. Therefore, the existence of high winds in the atmospheres of Jupiter and Saturn is not a good argument for recent origin. However, the related factor of internal heat is, as I now discuss.
Heat Loss from Jovian Planets
Three of the four Jovian planets emit far more radiation than they receive from the sun (Samec 2000). The one exception is Uranus (Henry 2001). What is the source of this excess energy? There are two obvious answers. One possibility is that these three planets are radiating primordial heat. However, the timescale for shedding all their primordial heat is far less than the supposed 4.5-billion-year age of the solar system. Another possibility is that these planets are undergoing settling, with denser material falling to greater depth and liberating gravitational potential energy in the process. However, the timescale for this process also is far less than the supposed 4.5-billion-year age of the solar system. Samec (2000) critiqued a desperate attempt to explain this energy crisis in an ancient solar system by invoking unusual conditions within Jupiter that allowed a deuterium-deuterium nuclear reaction. The internal conditions of Jupiter would seem to rule out this possibility. Furthermore, this proposed mechanism would not explain the energy surplus of Saturn and Neptune.
Of course, either the presence of primordial heat or settling works within the timescale of recent creation, so this is a good argument for recent origin.
Volcanism on Io
In 1979, the Voyager spacecraft revealed many volcanic eruptions on the surface of Io, the innermost Galilean satellite of Jupiter. The extent of this activity has been more fully documented by the more recent Galileo probe (fig. 10). This work revealed that Io is the most volcanically active body in the solar system. Volcanism requires an internal heat source. As with the Jovian planets, primordial heat is a possible source of the heat, but the timescale for this mechanism is far too short to work if the age of Io is 4.5 billion years (Ackerman 1986, 43–45; Rybka 1993, 70–71; Steidl 1983, 89–90). The problem is even worse for smaller members of the solar system such as Io, because their heat loss rate is so much greater than for larger objects. The earth’s internal heat is explained by the likely presence of radioactive isotopes within the earth’s interior. However, the radioactive isotopes required for this typically are dense, and the low density of Io makes this an unlikely heat source. To explain Io’s internal heat, secular scientists have resorted to tidal flexing as a heat source. Spencer (2003) has reviewed the tidal mechanism to explain Io’s internal heat and found it wanting. Therefore, volcanism on Io provides good evidence that it cannot be billions of years old.
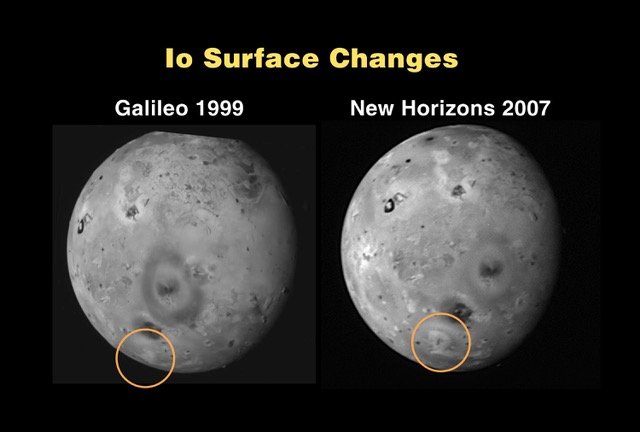
Fig. 10. A comparison of changes in the surface of Io over eight years, from the Galileo mission (1999) to the New Horizons mission (2007). The changes were due to volcanic activity.
Cryovolcanism on Enceladus and Triton
Like Jupiter’s satellite Io, Saturn’s even smaller satellite Enceladus shows evidence of volcanism. The Cassini probe to Saturn revealed eruptions on the south pole of Enceladus (fig. 11). To clarify, the molten material on Enceladus primarily is water, not sulfur, as on Io, or rock, as on earth. Since the melting and boiling points of water are so much lower than those of rock and sulfur, it is more proper to refer to these eruptions coming from geysers rather than volcanoes. Planetary scientists have coined the term cryovolcanism to refer to eruptions where the erupting material is water (or other volatiles, such as methane or ammonia) as on Enceladus. Evidence of past cryovolcanism exists on other satellites of the outer planets, and in 1989 the Voyager 2 spacecraft observed geyser eruptions on Neptune’s satellite Triton. This raises the question of what the source of energy is to power the cyrovolcanism on Enceladus. As with the volcanism on Io, tidal flexing has been invoked. However, as with Io, tidal flexing is inadequate to explain this, as has been acknowledged in the secular literature (Porco et al. 2006).
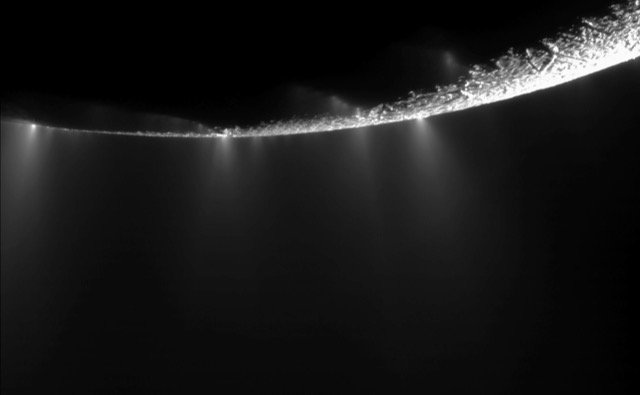
Fig. 11. Cryovolcanic eruptions near the South Pole of Enceladus.
While this appears to be a good argument for recent origin of Enceladus, it has received scant mention in the creation literature (Coppedge 2006; Spencer 2015b), but there has been no discussion of Triton. Neither satellite has been discussed in this context in the technical creation literature. There is great need for a discussion of Enceladus, along with Triton, and other small, active bodies in the solar system, in the technical creation literature.
Hydrogen and Methane in Titan’s Atmosphere
Titan is the only satellite in the solar system with an appreciable atmosphere. Surprisingly, even with far less mass, and hence less surface gravity, than the earth, Titan’s atmosphere is more substantial than earth’s atmosphere. For a body to possess an atmosphere, the average speed of the molecules making up the atmosphere must move significantly less than the escape velocity of the body. Being nearly ten times farther from the sun than the earth is, Titan’s temperature is much less than the earth’s temperature, so the molecules in Titan’s atmosphere are moving more slowly than they would if Titan were much warmer, making Titan’s atmosphere possible.
Titan’s atmosphere is more than 90% nitrogen, with much of the remainder being methane, and 0.1–0.2% being molecular hydrogen (Coustenis and Taylor 2008, 154–155). Rybka (1993, 81–83) noted that the hydrogen molecule is so light that its average speed at the atmospheric temperature of Titan is fast enough to escape. Therefore, he reasoned, the presence of hydrogen in Titan’s atmosphere indicates a recent origin. Rybka acknowledged the possibility of creation of hydrogen in Titan’s atmosphere from photodissociation from organic molecules by solar ultraviolet light. But Rybka dismissed that, based upon Titan’s great distance from the sun (at Titan’s distance from the sun, it receives only 1% the radiation the earth does per unit area), and so he concluded that until a satisfactory rate of photodissociation is shown, the existence of hydrogen in Titan’s atmosphere is a good indicator of youth. There undoubtedly is at least some photodissociation of hydrogen from organic molecules in its atmosphere, but there are two other mechanisms that contribute to the photodissociation, energetic charged particles in Saturn’s magnetosphere and cosmic rays. Therefore, it is likely that the small amount of hydrogen in Titan’s atmosphere is the steady-state, and so this is not a good argument for recent creation.
However, this brings up a related point. Solar radiation effectively breaks up methane into other hydrocarbons. The time required to do this is about 50 million years, so why is there still methane in Titan’s atmosphere, if it is billions of years old? The proposed solution to this problem is that methane is released from geological processes on Titan so that methane destroyed is continually replaced (Coustenis, 2005). But Spencer (2011) argues this process is not adequate to explain the Methane content of Titan’s atmosphere. Therefore, it appears that is may be a good indicator of recent origin.
The Orbit of Triton
Neptune’s satellite Triton has a most peculiar orbit (Faulkner 2014a). It is the only major satellite that orbits retrograde. It also has a large inclination to both the orbital and equatorial planes of Neptune. Except for its size, these characteristics would classify Triton as an irregular satellite, which suggests that it is an asteroid captured by Neptune’s gravity. However, Triton’s very low orbital eccentricity would argue against that. There is much debate as to how Triton came to be. Steidl (1979, 57) and Rybka (1993, 84–85) stated that tidal processes cause Triton’s orbit to decay, with a collision with Neptune likely within 10–100 million years, thus implying Triton’s orbit as a possible young-age indicator. However, the thinking must have changed on this, because a more recent study (Chyba, Jankowski, and Nicholson 1989) concluded that this will happen in approximately 3.6 billion years. If this is true, then this age indicates the timescale of the evolution of Triton’s orbit is quite long, and hence might not be a good argument for recent origin.
Pluto/Charon
The New Horizons mission flew past the Pluto/Charon system in July 2015, revealing surface features in detail for the first time (Spencer 2015a). The greatest surprise was the sparseness of craters on the surface of either body. Based upon the assumption of a naturalistic origin and history spanning billions of years, astronomers thought that the surfaces of Pluto and Charon would be saturated with craters. Most notable was a large heart-shaped light-colored region on Pluto that appears completely devoid of any craters. Most likely, this region has been reworked with ice, but how and when did this happen? A process that could rework a surface of a body once it formed requires heat, and given Pluto’s small size and low density, there is no known heat source. Other surprises included a thin Plutonian atmosphere that appears to be dissipating, and large mountain ranges on both Pluto and Charon. It may be premature to identify these discoveries as evidence of recent origin. However, at this time there is no scenario based upon billions of years that can explain the surface of Pluto.
Planetary Rings
Biblical creationists have long used the existence of planetary ring systems as evidence of recent origin (Ackerman 1986, 45–47; Boardman, Koontz, and Morris 1973, 149–150; Henry 2006a; Rybka 1993, 74–80; Slusher 1980, 65–72; Snelling 1997; Steidl 1983, 92–96; Tippets 1979). Saturn’s rings (fig. 12) were discovered four centuries ago, shortly after the invention of the telescope. Until four decades ago, most astronomers thought that Saturn’s rings were a long-lived feature. However, the discovery in the 1970s that all three of the other Jovian planets have rings challenged that view. The rings of the other three Jovian planets are very difficult to directly image on earth, and have been successfully imaged only in recent years, sometime after their discovery. So how were the rings of the other three Jovian planets discovered? Two ring systems (those of Uranus and Neptune) were discovered by occultations of stars. Thinking that ring systems may be a feature common to all four Jovian planets, the Voyager team used the camera aboard that spacecraft to search successfully for a ring system around Jupiter. The sparseness of the three ring systems discovered in the 1970s indicated that there are significant erosion processes acting to destroy the rings. Furthermore, the Voyager flyby missions and the orbital Cassini missions documented substantial changes in the rings in relatively short time. It is interesting that there were data going back more than a century earlier suggesting rapid changes in Saturn’s rings, though astronomers largely ignored that data, based upon the assumption that the rings were stable over the very long lifetime of the solar system (derived from the assumption of naturalism and uniformitarianism).
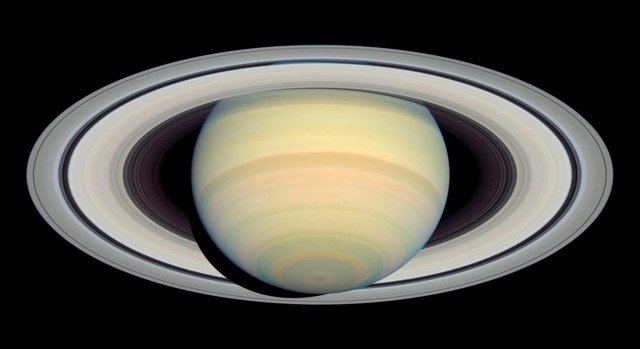
Fig. 12. Cassini probe image of Saturn, showing much structure in the planet’s atmosphere and in the ring system.
There are several mechanisms that erode rings:
- Collisions between ring particles
- Gravitational perturbations of satellites
- Collisions from meteoroids
- Radiation on small ring particles
- Magnetic effects on small charged ring particles
The revolution beginning in the 1970s resulted in the virtually unanimous conclusion that planetary rings are transitory phenomenon, lasting at most a few million years. Presumably, rings of the Jovian planets have formed and dissipated, only to be replaced by new rings that repeat the process. Otherwise, what is the probability that in a 4.5 billion-year-old solar system, we just happen to be living at a time when all four Jovian planets have ring systems?
How do evolutionists suggest ring systems form? They propose that a body, most likely a wayward asteroid or perturbed satellite, ventures too close to the planet (within the planet’s Roche limit), and is shredded by tidal forces raised by the planet. If ring systems repeatedly form and dissipate, then each of the Jovian planets likely have had hundreds or even thousands of ring systems over the past 4.5 billion years. A candidate body cannot be too small, for small bodies are held together by cohesive forces of chemical bonds, but the Roche limit applies only to bodies held together by self-gravity. Therefore, the body that is shredded must have some appreciable size. How many candidate bodies do the Jovian planets now have, and how many have they had in the past?
This argument for recent origin warrants continued monitoring and response as new information and models are proposed. However, for now it appears to be a good argument for recent origin, at least for ring systems. It does not necessarily follow that the earth and the solar system must be young as well. However, if the solar system is 4.5 billion years old, one must question whether the process of ring formation has happened countless times and presumably will continue indefinitely, or if we just happen to live at an epoch when this rare event has happened for each of the Jovian planets.
Poynting-Robertson Effect
There is much dust in the plane of the solar system. This dust manifests itself most distinctly as the zodiacal light (fig. 13) as the dust particles scatter sunlight. Scattering of sunlight by dust is a major contributor to background light in a moonless, dark, clear, sky. The dust particles typically are 10–300μm in size. Solar radiation blows particles smaller than 10μm outward from the sun. This is why dust tails of comets are directed away from the sun. The fate of larger particles is very different. The Poynting-Robertson effect is a very subtle process that acts on particles larger than 10μm. One can elucidate the Poynting-Robertson effect either classically (as John Henry Poynting did) or using general relativity (which Howard P. Robertson did).
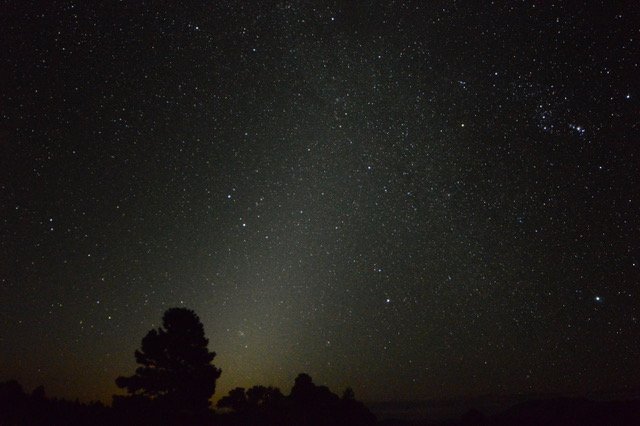
Fig. 13. Photo of the zodiacal light taken by the author on the morning of September 1, 2019. The zodiacal light is the glow to the right of the tall tree and extending upward and slightly to the right.
Consider a dust particle orbiting the sun. From the reference frame of the particle, there is a small component of sunlight in the direction it is moving, similar to the aberration of starlight. Thus, absorption of sunlight in the direction of motion results in a small force opposite the direction of the particle’s motion. The absorbed radiation from the sun is reemitted in all directions, so that process yields no net force. Therefore, the resultant force retards the particles forward motion. Alternately, from the sun’s perspective, the absorption of sunlight is entirely radial, so there is no change in the particle’s angular momentum. However, in this frame, the reemission of energy absorbed from the sun is not isotropic. Instead, there is a net component in the direction of the particle’s motion. Either way, the particle is robbed of angular momentum, so the particle falls to a lower orbit, whereupon the force increases. Eventually, the particle spirals into the sun, or at least close enough to the sun that intense solar radiation destroys the particle. The timescale for infall is related to particle size as well as orbital size. For small particles within the earth’s orbit, the infall time is on the order of thousands of years.
Given this short timescale and that there is abundant dust in the solar system, the Poynting-Robertson effect has been invoked by biblical creationists as an argument for recent origin (Ackerman 1986, 31–35; Rybka 1993, 90–95; Samec 1975; Slusher 1971; Slusher 1980, 55–64; Steidl, 1979, 60–61). The most complete treatment in the creation literature is that of Slusher and Robertson (1982). They computed various times of infall for particle sizes and orbital distances and reported that in two billion years, all particles with less than 188cm diameter within the earth’s orbit, all particles less than 7.6cm diameter within Jupiter’s orbit, and all particles less than 0.22cm within Neptune’s orbit would be removed. This obviously is not the case, suggesting that the solar system is far younger than two billion years (let alone 4.5 billion years). Brown (2008, 40) has further noted that the Poynting-Robertson effect not only cleanses the solar system, but that it also ought to filter and segregate particle size in the solar system, but that this is not observed to be the case.
Is there a response to this difficulty from those who think that solar system is billions of years old? Yes. It is supposed that as dust is removed, it is replaced by dust produced by collisions of minor planets and the disintegration of comets. For a long time, it appeared that these mechanisms are too modest to balance the loss of dust (Steidl 1983, 103–104). However, in recent years astronomers have discovered that the number of comets has been grossly underestimated, with the development of LINEAR (LIncoln Near-Earth Asteroid Research), Pan-STARRS (Panoramic Survey Telescope and Rapid Response System), and the now defunct Catalina Sky Survey (CSS) that succeeded in finding many comets that were too faint to detect in the past. It may be that the greater number of objects that previously had eluded detection may address this deficit.
The primary references to the Poynting-Robertson effect in the creation literature are decades old. However, the topic is not discussed much in the conventional astronomical literature either. This subject warrants an updating in the creation literature, but in the meantime, it appears to be a good argument for recent origin of the solar system.
Dispersion of Meteor Showers
Comets are flimsy objects, losing many small, solid particles with each perihelion passage (see the discussion of comets below). This disintegration tends to spread debris along the orbital path of the comet. Throughout the year, the earth experiences meteor showers as it crosses the orbits of various comets and collides with the debris scattered along their orbits. We know of the association between shower meteors and comets, because by observing meteor trails from two locations simultaneously, we can infer the motions of the meteoroids prior to entering the earth’s atmosphere. The orbits of these meteoroids have characteristics of comet orbits. Indeed, some meteor shower streams are identified with the orbits of known comets. Presumably, those meteor shower streams unassociated with any known comet are debris of now-defunct comets.
The Poynting-Robertson effect ought to segregate meteoroids within a meteor shower stream according to size on a relatively short timescale, yet this generally is not observed (Rybka 1993, 96–97; Slusher 1980, 60–63). Hence, the lack of segregation may indicate recent origin. Complicating the situation is the addition of new particles as comets continue to shed them. A detailed analysis of the processes involved is most desirable. But in the meantime, this may be a good indication of recent origin.
Comets
Biblical creationists have long used the existence of comets (fig. 14) has evidence for recent origin (Ackerman 1986, 35–38; Armstrong 1971; Faulkner 1997, 1998; Rybka 1993, 85–89; Slusher 1971; Steidl 1979, 58–60; Steidl 1987; Stillman 1990). It is indisputable that individual comets have lifetimes far less than the supposed 4.5 billion-year age of the solar system. Comets suffer from three primary loss mechanisms:
- Evaporation
- Collisions with planets
- Ejection from the solar system
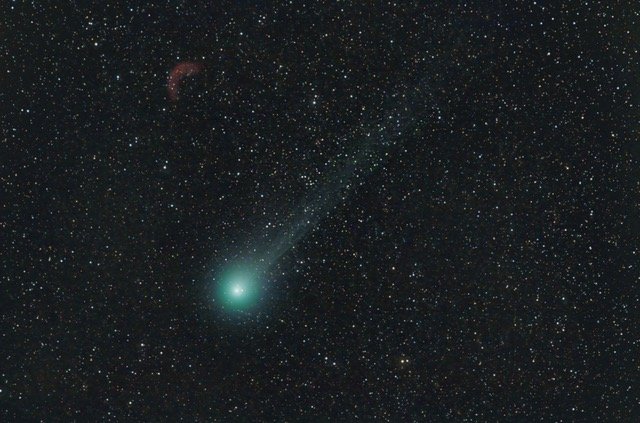
Fig. 14. Comet Lovejoy. (photo courtesy Jim Bonser)
All three mechanisms have been observed. The first loss mechanism generally is gradual, while the other two are catastrophic. Considering evaporation alone, the maximum number of orbits a comet could complete and still survive is perhaps one hundred (and this is a liberal estimate). The maximum orbital period that a comet could have and still be bound to the solar system is on the order of a few million years. Therefore, it is difficult to conceive that any comets could exist, if the solar system is billions of years old.
This difficulty was recognized by astronomers long before creationists began using the existence of comets as an argument for recent origin, for one of the primary solutions to the problem, the Oort cloud, was proposed in 1950. The Oort cloud is a hypothetical spherical distribution of comet nuclei orbiting the sun at great distance. Occasional gravitational perturbations from objects outside the solar system are thought to alter the orbits of Oort cloud objects, causing some of them to assume orbits that plunge them into the inner solar system once each orbit. When these nuclei are close to the sun, solar radiation sublimes volatile materials briefly to produce the bright coma and tail of the comet. In this manner, new comets supposedly replace old comets as they are eliminated.
Astronomers recognize two types of comets:
- Short-period comets
- Long-period comets
Short-period comets generally are defined to have orbital periods of less than 200 years, while long-period comets generally are defined to have periods greater than 200 years. Despite the names of this classification, the more important distinction is the types of orbits the two groups follow. Short-period comets tend to have relatively low eccentricity, low inclination, prograde orbits (orbiting the same direction planets orbit the sun, CCW as viewed from above the earth’s North Pole). However, long-period comets have high eccentricity, high-inclination orbits, with about half being prograde and half being retrograde. To illustrate that these generalities have exceptions, the most famous comet, 1P/Halley, has a period of about 75 years, but with an inclination of 162.3°, its orbit is highly inclined (alternately, 1P/Halley has an inclination of 17.7° and orbits retrograde).
Jan Oort had devised his namesake cloud in terms of long-period comets, but where do replacements for short-period comets come from? For the first three decades after Oort proposed the Oort cloud, astronomers thought that gravitational perturbations of the planets (mostly Jupiter) altered long-period comet orbits further, converting long-period comets into short-period comets. However, by the 1980s, simulations showed that the conversion mechanism was far too inefficient to transform a significant number of long-period comets into short-period comets. Therefore, in the 1980s, astronomers resurrected the Kuiper belt, something proposed by Gerard Kuiper about the time that Oort proposed the Oort cloud. The Kuiper belt is a hypothetical toroidal-shaped distribution of comet nuclei beyond the orbit of Neptune. Ironically, Kuiper had proposed that the Kuiper belt had existed in the early solar system, but no longer was there, because gravitational perturbations of the newly formed Jovian planets rapidly depleted its members as they were elevated to the Oort cloud. However, in the 1980s this view changed, as astronomers continued to believe that the Oort cloud had been populated by the Kuiper belt, but that there were many objects still in the Kuiper belt. Therefore, for more than three decades, astronomers generally have believed that the Oort cloud is the source for long-period comets, while the Kuiper belt is the source for short-period comets.
Do the Oort cloud and Kuiper belt exist? There is no evidence for the Oort cloud, nor could there be, given the very small size of comet nuclei and their extreme distance from both the sun and earth that hypothetical denizens of the Oort cloud would be. In the 1990s, astronomers realized that Kuiper belt objects, if they exist, could be detected with technology that then available, so they mounted efforts to look for them. These searches have yielded many small bodies orbiting beyond the orbit of Neptune. These discoveries were hailed as confirmation of the Kuiper belt’s existence, with many astronomers referring to them as KBOs (Kuiper Belt Objects). Soon an alternate, more descriptive and less interpretation laden name arose, TNOs, (Trans Neptunian Objects). For obvious reasons, I prefer the term TNO over KBO, and I suggest its use in the creation literature over KBO whenever possible. Interestingly, I have noticed a gradual increase in the use of TNOs over KBOs in the conventional astronomy literature, but I don’t know why.
Does the Kuiper belt exist? Several papers have appeared in the creation literature questioning this interpretation of TNOs (Faulkner 1997; Newton 2002; Oard 2005; Worraker 2004). There are many problems with TNOs being KBOs, such as the extremely large size of many of them (Pluto and Charon, for instance), far larger than any observed comet nucleus. And there are questions about the Oort cloud as well, beyond the fact that it has not been observed (Faulkner 2001; Spencer 2014). Creationists generally have done a good job keeping up with changes in this field and responding to them, something that we, unfortunately, are not always so quick to do. Despite much work on the part of evolutionists to explain the existence of comets in an ancient solar system, comets remain a good argument for recent origin. However, any discussion of this evidence ought to include mention of the Kuiper belt and Oort cloud.
Earth-Crossing Asteroids
As their name suggests, earth-crossing asteroids are asteroids whose orbits cross earth’s orbit, introducing the potential of collisions. Impacts of asteroids of sufficient size would produce significant craters that should survive in some form for considerable time. Indeed, many astroblemes, or “fossil craters” have been identified on earth (Spencer 1998; 1999). However, there appear to be fewer astroblemes than can be accounted for on the evolutionary timescale (Austin 1984; Rybka 1993, 96; Steidl 1983, 101–102). This suggests that the evolutionary timescale is too long. Coincidentally, recent creationists interpret astroblemes in terms of intense cratering at the time of the Flood. This appears to be a good argument for recent origin.
Lifetimes of Certain Asteroid Orbits
Rybka (1993, 84) mentioned the asteroids 2060 Chiron and 944 Hidalgo as examples of objects that likely will be ejected from the solar system on relatively short timescales compared to the supposed 4.5-billion-year age of the solar system. Rybka stated that Chiron is likely to be ejected within a few hundred thousand years, while Hidalgo was likely to be ejected in 1–2 million years, and so he concluded that these objects imply a recent origin.
Rybka was correct in inferring a recent origin for these bodies, at least in terms of how long they have been in their current orbits. The reason for their orbital instability is that they have eccentric orbits that cross the orbits of the two most massive planets in the solar system (Hidalgo crosses Jupiter’s orbit, while Chiron crosses Saturn’s orbit). The orbits of these two asteroids have high probability of experiencing gravitational perturbations of Jupiter and Saturn that can dramatically alter their orbits, either greatly shortening their orbits or lengthening their orbits, with the latter possibly leading to ejection from the solar system. This is the same process that ejects comets from the solar system. Therefore, the existence of Chiron and Hidalgo in their current orbits merely proves that they have been in those orbits on timescales far less than billions of years. Presumably in the past they were perturbed from previous orbits into their current orbits. Therefore, this is not a good argument for recent origin.
Conclusion
I have evaluated 25 arguments for recent origin from the solar system that biblical creationists have used. I have omitted discussion of the magnetic fields of other planets, as well as the earth’s magnetic field, because I thought it best that that topic be covered in a separate work dedicated to geological evidences for recent origin. I will discuss arguments for recent origin coming from the sun, stars, the galaxy, and extragalactic astronomy in a subsequent paper.
I discourage the use of those young-age indicators that do not survive scrutiny. Some young-age indicators appear to be strong, but biblical creationists must be vigilant to keep abreast of new developments that may affect even the good arguments. Other biblical creationists may disagree with some of my evaluations. I certainly encourage further discussion of the existing young-age arguments, as well as the development of new arguments for recent origin.
References
Ackerman, Paul D. 1986. It’s a Young World After All: Exciting Evidences for Recent Creation. Grand Rapids, Michigan: Baker.
Armstrong, H. 1971. “Comets and a Young Solar System.” Creation Research Society Quarterly 8, no.3: 192–193.
Armstrong, H.L. 1978. “Missing Meteorites.” Creation Research Society Quarterly 15: 68.
Austin, Steven A. 1984. Catastrophes in Earth History: A Source Book of Geologic Evidence, Speculation and Theory. ICR Technical Monograph 13. El Cajon, California: Institute for Creation Research.
Barnes, Thomas G. 1974. “Physics: A Challenge To ‘Geological Time.’” Impact no. 16, July 1.
Barnes, T.G. 1982. “Young Age for the Moon and Earth.” Acts & Facts 11, no.8, August 1.
Bills, Bruce G., Gregory A. Neumann, David E. Smith, and Maria T. Zuber. 2005. “Improved Estimate Of Tidal Dissipation Within Mars From MOLA Observations Of The Shadow Of Phobos.” Journal of the Geophysical Research 110, E07004. doi:10.1029/2004JE002376.
Boardman, William W., Robert F. Koontz, and Henry M. Morris. 1973. Science and Creation Creation-Science Research Center: San Diego, California.
Brill, Robert. 2011. “Does Glass Flow.” September 29. http://www.cmog.org/article/does-glass-flow.
Brown, Walt. 2008. In the Beginning: Compelling Evidence for Creation and the Flood. 8th ed. Phoenix, Arizona: Center for Scientific Creation.
Burns, Joseph A. 1978. “The Dynamical Evolution And Origin Of The Martian Moons.” Vistas in Astronomy 22, part 2: 193–210.
Cameron, Winifred Sawtell. 1972. “Comparative Analysis Of Observations Of Lunar Transient Phenomena.” Icarus 16, no.2 (April): 339–387.
Cameron, Winifred Sawtell. 1991. “Lunar Transient Phenomena.” Sky and Telescope 81, no.3:265–268.
Chyba, Christopher Frank, David Gerard Jankowski, and Philip D. Nicholson. 1989. “Tidal Evolution in the Neptune-Triton System.” Astronomy and Astrophysics 219 (June 1): L23–L26.
Coppedge, David F. 2006. “Enceladus: A Cold, Youthful Moon.” Acts & Facts 35 (11).
Coustenis, Athena. 2005. “Formation And Evolution Of Titan’s Atmosphere.” Space Science Reviews 116, nos.1–2 (January): 171–184.
Coustenis, Athena, and Fredric W. Taylor. 2008. Titan: Exploring and Earthlike World. 2nd ed. World Scientific: Singapore.
Crotts, Arlin P.S. 2009. “Transient Lunar Phenomena: Regularity And Reality.” Astrophysical Journal 697, no.1 (April 30): 1–15.
DeYoung, Don B. 1990. “The Earth-Moon System.” In Proceedings of the Second International Conference on Creationism. Edited by R.E. Walsh, 79–84. Pittsburgh, Pennsylvania: Creation Science Fellowship.
DeYoung, Don B. 2003. “Transient Lunar Phenomena: A Permanent Problem For Evolutionary Models Of Moon Formation.” TJ 17, no.1 (April):5–6.
DeYoung, Donald B. 2010. Astronomy and the Bible: Questions and Answers. Winona Lake, Indiana: BMH Books.
DeYoung, Don, and John Whitcomb. 2003. Our Created Moon: Earth’s Fascinating Neighbor. Green Forest, Arkansas: Master Books.
DeYoung, Don, and John Whitcomb. 2010. Our Created Moon: Earth’s Fascinating Neighbor. Revised ed. Green Forest, Arkansas: Master Books.
Faulkner, Danny. 1997. “Comets And The Age Of The Solar System.” Creation Ex Nihilo Technical Journal 11, no.3 (December): 264–273.
Faulkner, D. 1998. “The Current State Of Creation Astronomy.” In Proceedings of the Fourth International Conference on Creationism. Edited by R.E. Walsh, 201–216. Pittsburgh, Pennsylvania: Creation Science Fellowship.
Faulkner, Danny. 1999. “A Biblically-Based Cratering Theory.” Creation Ex Nihilo Technical Journal 13, no.1 (April): 100–104.
Faulkner, Danny. 2000. “‘Response To Faulkner’s “BiblicallyBased Cratering Theory.”’ Reply By Faulkner.” Creation Ex Nihilo Technical Journal 14, no.1 (April): 47–49.
Faulkner, D. 2001. “More Problems for the ‘Oort Cloud’.” TJ 15, no.2 (August): 11.
Faulkner, Danny R. 2013. “An Analysis Of The Dodwell Hypothesis.” Answers Research Journal 6 (May 15): 179–191.
Faulkner, Danny R. 2014a. “Interpreting Craters In Terms Of The Day Four Cratering Hypothesis.” Answers Research Journal 7 (January 22): 11–25.
Faulkner, Danny R. 2014b. “Anomalies With Planets And Satellites In The Solar System—Indication Of Design?” Answers Research Journal 7 (June 25): 205–214.
Faulkner, D.R. 2017. The Expanse of Heaven: Where Creation and Astronomy Intersect. Green Forest, Arkansas: Master Books.
Henry, Jonathan. 2001. “The Energy Balance Of Uranus: Implications For Special Creation.” TJ 15, no.3 (December): 85–91.
Henry, Jonathan. 2006a. “The Age And Fate Of Saturn’s Rings.” Journal of Creation 20, no.1 (March): 123–127.
Henry, Jonathan. 2006b. “The Moon’s Recession And Age.” Journal of Creation 20, no.2 (August): 65–70.
Henry, Jonathan. 2006c. “Transient Lunar Phenomena: Evidence for a Young, Active Moon.” Creation Matters 11, no.3 (May–June): 5–6.
Kuban, G.J. 1991. “Moon Dust.” Creation Research Society Quarterly 28 (2):74–75.
Lisle, Jason. 2013. “The Solar System: Earth and Moon.” Acts and Facts 42, no.10 (September 30): 10–12.
Markiewicz, W.J., D.V. Titov, S.S. Limaye, H.U. Keller, M. Ignatiev, R. Jaumann, N. Thomas, et al. 2007. “Morphology And Dynamics Of The Upper Cloud Layer Of Venus.” Nature 450 (29 November): 633–636.
Morris, Henry M. 1985 (ed.). Scientific Creationism. 2nd edition. El Cajon, California: Master Books.
Mouginis-Mark, Peter J. 2016. “Geomorphology And Volcanology Of Maat Mons, Venus.” Icarus 277 (October): 433–441.
Morton, Glen R., Harold S. Slusher, and Richard E. Mandock. 1983. “The Age Of Lunar Craters.” Creation Research Society Quarterly 20, no.2 (September): 105–108.
Newton, Robert. 2002. “The Short-Period Comets ‘Problem’ (For Evolutionists): Have Recent ‘Kuiper Belt’ Discoveries Solved The Evolution/Long-Age Dilemma?” TJ 16, no.2 (August): 15–17.
Oard, Michael J. 2005. “Kuiper Belt Woes For Accretion Disk Models.” TJ 19, no.2 (August): 10–11.
Pollack, James B. 1981. “Atmospheres Of The Terrestrial Planets.” In The New Solar System. Editors: J.K. Beatty, B. O’Leary, and A. Chaikin. Sky Publishing: Cambridge, Massachusetts.
Porco, C.C., P. Helfenstein, P.C. Thomas, A.P. Ingersoll, J. Wisdom, R. West, G. Neukum, et al. 2006. “Cassini Observes The Active South Pole Of Enceladus.” Science 331, no.5766 (10 March): 1393–1401.
Psarris, S. 2010. “The Moon’s Recession from the Earth: Still A Valid Young-Age Indicator.” Creation Research Society Quarterly 46, no.3: 230.
Robinson, J.H. 1991. “Some Possible Causes Of LTPs.” Sky and Telescope 81, no.3: 268.
Robinson, Cordula A., Gill D. Thornhill, and Elisabeth A. Parfitt. 1995. “Large-Scale Volcanic Activity At Maat Mons: Can This Explain Fluctuations In Atmospheric Chemistry Observed By Pioneer Venus?” Journal of Geophysical Research 100, E6 (25 June): 11755–11763.
Rybka, Theodore Wesley. 1993. Geophysical and Astronomical Clocks. American Writing and Publishing: Irvine, California.
Samec, R.G. 1975. “Effect Of Radiation Pressure On Micrometeoroids, And Existence Of Micrometeoroids As Evidence Of A Young Solar System.” Creation Research Society Quarterly 12, no.1 (June): 7–13.
Samec, Ron. 2000. “The Age Of The Jovian Planets.” CEN Technical Journal 14, no.1 (April): 3–4.
Samec, Ronald G. 2008a. “On The Origin Of The Lunar Maria.” Journal of Creation 22, no.3 (December): 101–108.
Samec, Ronald G. 2008b. “Is The Moon’s Orbit ‘Ringing’ From An Asteroid Collision Event Which Triggered The Flood?” In Proceedings of the Sixth International Conference on Creationism. Edited by A.A. Snelling, 255–261. Pittsburgh, Pennsylvania: Creation Science Fellowship and Dallas, Texas: Institute for Creation Research.
Setterfield, B. 1983. “An Asteroid Tilts the Earth? – Further Evidence.” Creation Ex Nihilo 5, no.4: 6–8.
Setterfield, B. 2010. “The Obliquity Of The Ecliptic” by George F. Dodwell. http://www.setterfield.org/Dodwell_manuscript_1.html.
Slusher, H.S. 1971. “Some Astronomical Evidences for a Youthful Solar System.” Creation Research Society Quarterly 8, no.1: 55–57.
Slusher, Harold S. 1980. Age of the Cosmos. ICR Technical Monograph 9. Institute for Creation Research: San Diego, California.
Slusher, Harold S., and Stephen J. Robertson. 1982. The Age Of The Solar System: A Study Of The Poynting-Robertson Effect And Extinction of Interplanetary Dust. 2nd ed. ICR Technical Monograph 6. Institute for Creation Research: El Cajon, California.
Snelling, A.A. 1997. “Saturn’s Rings Short-Lived And Young.” Creation Ex Nihilo Technical Journal 11, no.1 (April): 1.
Snelling, A.A. 2012. “Did Meteors Trigger Noah’s Flood?” Answers 7, no.1 (January 1): 68–71.
Snelling, Andrew A. and David E. Rush. 1993. “Moon Dust And The Age Of The Solar System.” Creation Ex Nihilo Technical Journal 7, no.1 (April): 2–42.
Spencer, Wayne R. 1998. “Geophysical Effects Of Impacts During The Genesis Flood.” In Proceedings of the Fourth International Conference on Creationism. Edited by R.L. Ivey, 585–595. Pittsburgh, Pennsylvania: Creation Science Fellowship.
Spencer, W. 1999. “Earth Impacts, The Geologic Column, And Chicxulub.” Creation Research Society Quarterly 36, no.3: 163–165.
Spencer, Wayne R. 2003. “Tidal Dissipation And The Age Of Io.” In Proceedings of the Fifth International Conference on Creationism. Edited by R.E. Walsh, 567–579. Pittsburgh, Pennsylvania: Creation Science Fellowship.
Spencer, Wayne. 2011. “Our Young Solar System.” Creation 33, no.4 (October): 38–41.
Spencer, Wayne. 2014. “Critique Of Modern Oort Comet Theory.” Creation Research Society Quarterly 50, no.3 (Winter): 146–153.
Spencer, Wayne. 2015a. “Rediscovering Pluto.” Journal of Creation 29, no.3 (December): 3–5.
Spencer, Wayne. 2015b. “Warm Icy Moons.” Journal of Creation 29, no.3 (December): 97–103.
Steidl, Paul M. 1979. The Earth, The Stars, And The Bible. Phillipsburg, New Jersey: Presbyterian and Reformed.
Steidl, Paul M. 1983. “Planets, Comets, and Asteroids.” In Design and Origins in Astronomy. Edited by George Mulfinger, 73–106. Creation Research Society Books.
Steidl, Paul M. 1987. “Comets and Creation.” Creation Research Society Quarterly 23, no.4 (March): 153–160.
Steveson, Peter A. 1975. “Meteoric Evidence For A Young Earth.” Creation Research Society Quarterly 12, no.1 (June): 23–25.
Stillman, William E. 1990. “The Lifetime And Renewal Of Comets”. In Proceedings of the Second International Conference on Creationism. Edited by R.E. Walsh and C.L Brooks, 267–278. Pittsburgh, Pennsylvania: Creation Science Fellowship.>
Tippets, M.W., 1979. “Voyager Scientists On Dilemma’s Horns.” Creation Research Society Quarterly 16, no.3 (December): 185.
Whitcomb, John C. and Donald B. DeYoung. 1978. The Moon: Its Creation, Form and Significance. Winona Lake, Indiana: BMH Books.
Wieland, Carl. 1983. “An Asteroid Tilts The Earth.” Creation Ex Nihilo 5, no.3 (January): 12–14.
Williams, David R. 2018. “Mercury Fact Sheet.” https://web.archive.org/web/20151106171436/http://nssdc.gsfc.nasa.gov/planetary/factsheet/mercuryfact.html.
Worraker, Bill. 2004. “Missing: A Source Of Short-Period Comets.” TJ 18, no.2 (August): 121–127.