The views expressed in this paper are those of the writer(s) and are not necessarily those of the ARJ Editor or Answers in Genesis.
Abstract
Granite plutons within Grand Canyon’s Precambrian crystalline basement were likely created supernaturally on Creation Days 1–2. They were then uplifted by the upheaval on Creation Day 3. These granites were not generated during the Flood because at the onset of the Flood they were already in place within the schists and were beveled before Flood sediment layers were deposited unconformably over them. Sixty-eight samples were collected from ten granite plutons and two pegmatite complexes. Radiohalos in these samples were identified and counted, only 27 (~40%) contain 238U radiohalos, compared to 46 (~68%) which contain 210Po radiohalos and 22 (~32%) which contain no radiohalos. Two models proposed previously to resolve the Po radiohalos enigma are creation by fiat of the host granites with their Po radiohalos during Creation Days 1–2, or rapid transport of the Po atoms by hydrothermal fluids expelled from rapidly crystallizing granite magmas while grossly accelerated radioactive decay was occurring during the Flood. Since these are supernaturally created granite plutons any radiohalos in them likely were annealed by the Creation Day 3 upheaval and the onset of the Flood. Thus, the origin of these radiohalos is consistent only with the hydrothermal fluid transport model for their formation during the Flood. Water trapped in the Flood-deposited sediment layers was heated by their burial and the residual heat generated by grossly accelerated radioactive decay. The favored model is that these hydrothermal fluids circulated into the underlying basement granites where they transported Po atoms generated by grossly accelerated decay of 238U within zircon crystals inside the granites’ biotite flakes. Some of these Po atoms were scavenged from solution at defect sites along cleavage planes within the biotite flakes and produced Po radiohalos, many without adjacent 238U radiohalos. Compared with a similar process that occurred in granite magmas that crystallized and cooled also during the Flood, this process in already solid Creation Week granites was a slower, less effective process that likely lasted for months. The 210Po atoms being longer-lived than 218Po and 214Po atoms were transported further by these hydrothermal fluids of lesser volume and less vigorous circulation than hydrothermal fluids expelled from granite magmas that were generated and crystallized during the Flood.
Keywords: Grand Canyon, inner Granite Gorges, Precambrian, granites, biotites, 238U radiohalos, 210Po radiohalos, zircons, fiat creation, hydrothermal fluids, the Flood
Introduction
Grand Canyon is a classic region for the study of geology from an evolutionary perspective, because its rocks span much of the earth’s claimed long history. From the granites and schists of the crystalline Precambrian basement in the inner Granite Gorges one can traverse upward through the tilted overlying Precambrian Grand Canyon Supergroup sedimentary strata sequence within the inner Granite Gorges, across the Great Unconformity marking the Precambrian-Phanerozoic boundary, then up through the flat-lying Paleozoic fossil-bearing sedimentary strata in the walls of the outer Canyon. To the north one can then climb the Grand Staircase through Mesozoic and Cenozoic sedimentary strata of the Colorado Plateau. Thus, the Grand Canyon region is an amazing place to study the sequence of strata produced during the earth’s history, making it a showcase for the uniformitarian slow-and-gradual billions-of-years (deep time) interpretative framework.
Therefore, the same Grand Canyon-Grand Staircase region provides a unique opportunity and challenge for Creation-Flood geologists to investigate the same strata sequence within a catastrophic Creation-Flood interpretative framework, demonstrating that it confirms the biblical account of earth’s history. Many research studies have already been undertaken and published. For example, it has been shown that many of the flat-lying Paleozoic sedimentary layers contain evidence of their rapid deposition, and that they can be traced beyond the Colorado Plateau as a stacked sequence of comparatively thin “pancake” layers that spread right across the North American continent, as would be expected from deposition in the global Flood cataclysm (Austin 1994; Clarey 2020; Clarey and Werner 2023; Snelling 2009, 2021a, b, 2022a, b; Whitmore et al. 2014; Whitmore and Garner 2018). But the scope of the Canyon’s geology means that this task is ongoing.
Perhaps the biggest challenge is dealing with the granites and schists of the Precambrian crystalline basement within the young-earth time framework of the biblical account of only 6,000–7,000 years total for the earth’s history. That history included two unique periods when today’s rates for geological processes did not apply—the Creation Week when God created a fully-formed, fully-functioning universe within six literal 24-hour days, and the year-long global Flood cataclysm when geological process rates were greatly accelerated.
The granites and schists of the Precambrian crystalline basement by virtue of being foundational to the overlying sedimentary strata sequences have thus been interpreted as Creation Week rocks, formed by God supernaturally during Days 1–2 (Austin 1994; Snelling 2009, 2022b). The fact that those rocks appear to have formed via processes such as melting and metamorphism of pre-existing rocks respectively would seem irrelevant because God created them supernaturally. When Jesus the Creator (John 1:1–3; Colossians 1:15–17; Hebrews 1:1–2) miraculously created more bread and fish from five loaves and two fish to feed 5,000 men plus women and children (John 6:1–13) those loaves and fish created by fiat looked the same as the ones in the boy’s lunch. Thus we would expect granites and schists created supernaturally to look like those formed, for example, during the Flood cataclysm.
So how would an investigator today know whether a granite was created supernaturally by God or was produced by crystallization from a magma? It was for this reason that when they were identified in granites, polonium radiohalos were called God’s “fingerprints of creation” due to the parent Po isotopes only having fleeting existences and the granites containing them were thus regarded as created (Gentry 1988). However, when Wise (1989) and Snelling (2000) highlighted that the Po radiohalos (microscopic halos due to crystal damage by radioactive decay) were also found in granites that intruded into Flood-deposited, fossil-bearing sedimentary layers, such granites had to form relatively rapidly during the Flood and thus could not have been created by fiat during the Creation Week. Therefore, Snelling and Armitage (2003) and Snelling (2005) proposed a hydrothermal fluid transport model for the formation of Po radiohalos that has subsequently been successfully tested.
Nevertheless, Po radiohalos are also found in Precambrian crystalline basement granites that are regarded as the foundation rocks from and on which today’s continents were formed (Snelling 2000, 2023b; Wise 1989). Thus, to understand these radiohalos better a careful systematic study was undertaken of radiohalos present in the granites of Grand Canyon’s Precambrian crystalline basement, particularly the polonium radiohalos, to assess their significance within the earth’s history, especially within the biblical framework.
The Grand Canyon Precambrian Granites
The east-west trending Grand Canyon presents spectacular exposures for 200 km of the Lower Proterozoic (Paleoproterozoic) rocks of the crystalline basement beneath the Colorado Plateau (Karlstrom et al. 2003). In the Upper Granite Gorge, these rocks are continuously exposed from river mile 78 to 120 (downstream from Lees Ferry), while there are discontinuous exposures in the Middle Granite Gorge from mile 127 to mile 137 (fig. 1) (Ilg et al. 1996; Karlstrom et al. 2003). The Lower Granite Gorge contains near-continuous outcrops from mile 207 to mile 261.
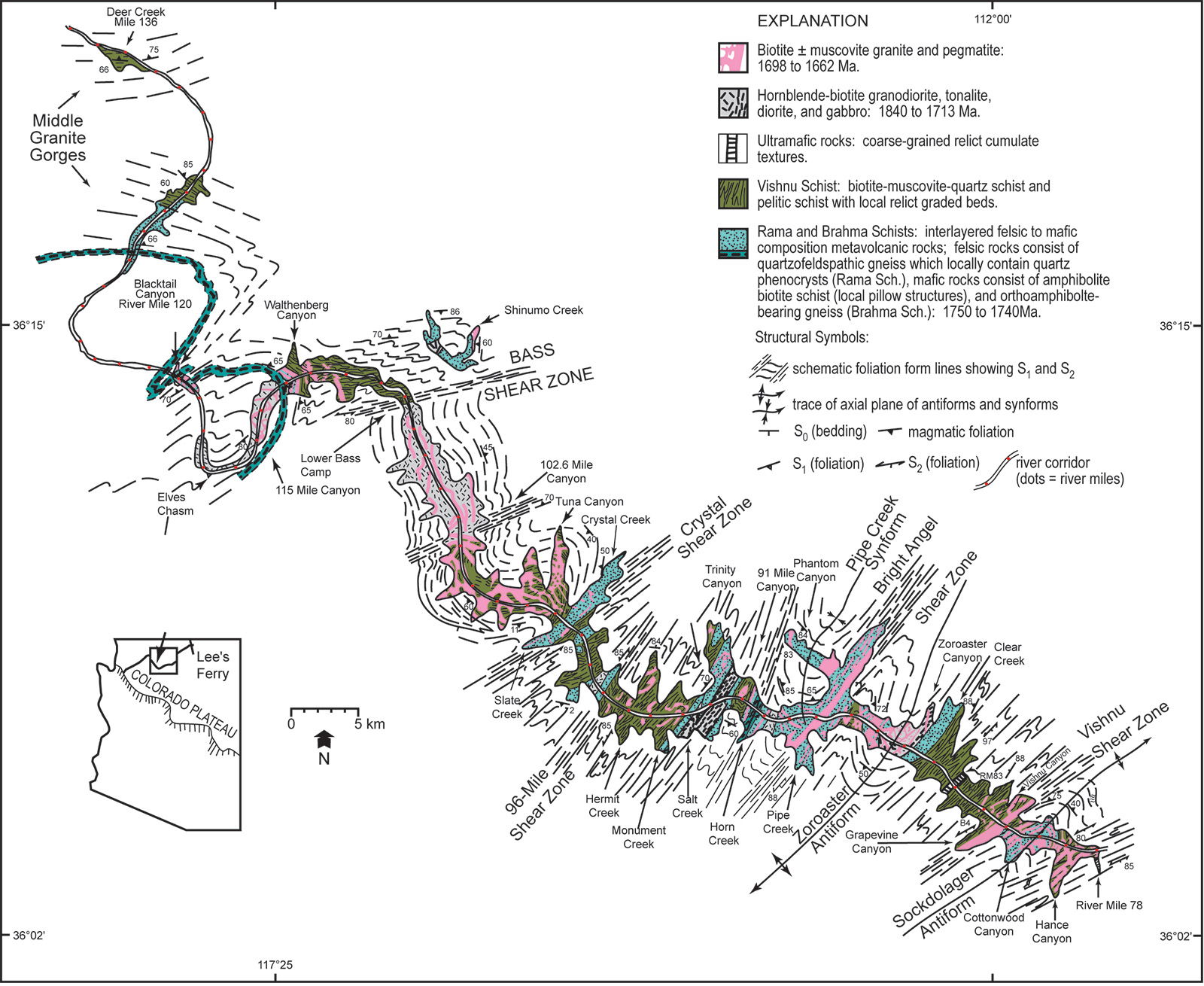
Fig. 1. Simplified geologic map of Paleoproterozoic (Lower Proterozoic) rocks in the Upper and Middle Granite Gorges, Grand Canyon, northern Arizona (after Ilg et al. 1996; Karlstrom et al. 2003). Form lines outside the Paleoproterozoic exposures show their interpretation of the trace of the regional foliation on the map surface. The transect is divided into metamorphic domains that are generally separated by shear zones.
Powell (1876) was the first to identify the Precambrian “granite” and “Grand Canyon schists.” Walcott (1889) identified the Vishnu “terrane” as a complex of schist and gneiss. Since then, the subdivisions of the Precambrian rocks have continued to be refined by numerous workers beginning with Noble and Hunter (1916). They identified domains of contrasting rock packages and recognized that differences reflected the presence of both metasedimentary and intrusive igneous rocks, and that some gneisses possibly were a basement on which the metasedimentary units were deposited. Subsequently, Campbell and Maxson (1938) identified different mappable units of the Vishnu and Brahma “Series” but underestimated the structural complexities and probably overestimated the stratigraphic thickness. Brown et al. (1979) also emphasized the complex deformational features and lumped all the metasedimentary and metavolcanic rocks under the name “Vishnu Complex,” while Babcock et al. (1979) described some of the granite plutons that intruded those metamorphic rocks. Finally, Babcock (1990) summarized the complex geology of the Precambrian rocks in the granite gorges as then mapped and understood.
As a result of renewed detailed field mapping and photogeologic interpretation of high-resolution aerial photographs of the river corridor, Ilg et al. (1996) presented an updated description of the Precambrian granites and a new map of the geology of the inner gorges that incorporated previous work. Ilg et al. (1996) provided new insights regarding the lithostratigraphy and the nature of the protoliths.
In updating the work of Ilg et al. (1996), Karlstrom et al. (2003) followed their strategy in recognizing the need simultaneously to pursue both tectonic and stratigraphic subdivisions of the Proterozoic crystalline basement rocks. The tectonic perspective is that the complex deformation and metamorphism of these rocks make it impossible to measure thickness and to confidently reconstruct regional stratigraphy. There are also important shear zones that are boundaries of unknown displacement, some possibly representing suturing of separate tectonic blocks that may once have been hundreds to even thousands of kilometers apart (Karlstrom et al. 2003). Thus, it is not certain that all the metasedimentary schists are strictly correlative—same age, same basin, same depositional sequence. However, the work of Hawkins et al. (1996), Ilg et al. (1996), and Karlstrom et al. (2003) permits a stratigraphic interpretation in which the metasedimentary rocks within the Canyon’s inner gorges are broadly of similar rock type and age (1.73–1.75 Ga). Thus, the entire metasedimentary-metavolcanic package could have been deposited in a single basin, or in similar but tectonically separate basins. For these reasons those metasedimentary and metavolcanic units have been grouped together as the Granite Gorge Metamorphic Suite.
Early studies in the Grand Canyon suggested that the quartzofeldspathic Elves Chasm Gneiss and Trinity Gneiss were basement to the schists (Noble and Hunter 1916). Subsequent workers recognized that the gneisses were deformed intrusive rocks (Babcock 1990; Brown et al. 1979; Campbell and Maxson 1938), but there was still debate on whether the Elves Chasm and Trinity gneisses are possible basement for the Vishnu Schist. However, the mapping by Ilg et al. (1996) and geochronology studies by Hawkins et al. (1996) indicate that the gneisses have different ages in different areas. Thus, the Trinity gneiss (Babcock et al. 1979) is interpreted as a deformed and metamorphosed granodiorite that intruded into the Granite Gorge Metamorphic Suite with a U-Pb zircon age of 1.73 Ga.
In contrast, the Elves Chasm pluton is 1.84 Ga (Hawkins et al. 1996), apparently the oldest rock in the southwestern United States and likely the basement for the turbidites that are now the Vishnu Schist. It is dominantly hornblende-biotite tonalite to quartz diorite and is distinguished geochemically from other plutons in Grand Canyon (Karlstrom et al. 2003). The contact zone between the Elves Chasm pluton and the overlying Granite Gorge Metamorphic Suite is exposed in several places and is gradational over several meters. The composition of the contact zone is unusual, which suggested to Babcock (1990) that alteration took place during weathering before the overlying sediments were deposited that are now the Vishnu Schist.
Intrusive rocks, primarily granites, make up half the Precambrian crystalline rocks of Grand Canyon’s inner gorges. Campbell and Maxson (1938) thought there was a single major period of granite intrusive activity and this led to lumping all the granites under the single name Zoroaster Granite (Babcock et al. 1979). However, the new mapping (fig. 1) (Ilg et al. 1996) and geochronology (Hawkins et al. 1996) show that the granitic rocks record an apparent long and complex development of the crust at numerous times between 1.84 and 1.37 Ga, with a wide variation in the types of granites based on their different petrology and geochemistry (Karlstrom et al. 2003). Partial crystallization and differentiation as the magmas cooled would have produced granites of different compositions. Thus, a single rock name is misleading, so the names of individual plutons or dike swarms are used. The mapped granite plutons and pegmatite complexes are depicted in cross-section in fig. 2. Some of these, the ones sampled in this study, are listed in table 1.
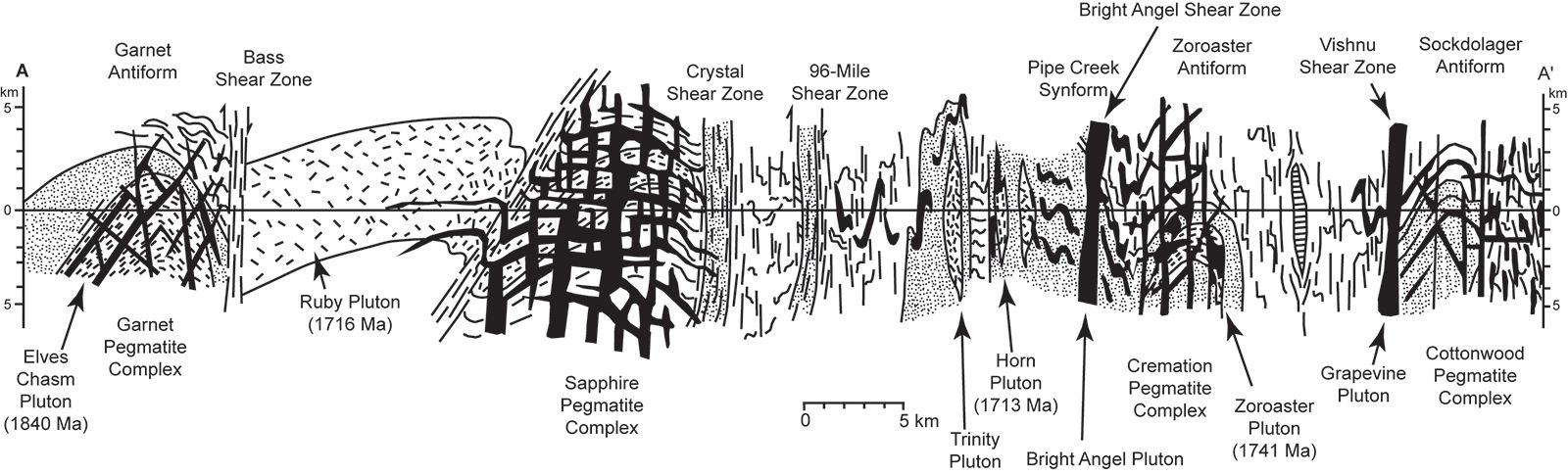
Fig. 2. Geologic cross section of the Upper Granite Gorge, Grand Canyon (as shown in fig. 1) (after Ilg et al. 1996). This cross section was constructed by projecting folds up and down plunge into the section line. It shows their interpretation of the macroscopic geometry of Paleoproterozoic rocks in Grand Canyon before uplift and erosion. The section shows ~10 km of vertical structural relief and large F2 folds that repeat lithologies across the transect. Shear zones are typically developed on the limbs of large folds or adjacent to heterogeneities such as granite plutons or pegmatite dike complexes.
Table 1. Grand Canyon’s Precambrian granite plutons and pegmatite complexes—their locations, ages and compositions.
Name | River Miles | Age (Ma) | Composition |
Cottonwood Pegmatite Complex | 77–82 | 1685 ± 1, 1680 ± 1 | Biotite and biotite-muscovite granite and granitic pegmatite dikes and sills |
Grapevine Camp Pluton | 81.5 | 1737 ± 1 | Medium-grained foliated biotite granite |
Zoroaster Pluton | 84.6–85.5 | 1740 ± 2 | Foliated medium-grained biotite granite to granodiorite orthogneiss |
Bright Angel Pluton | 88 | undated | Coarse-grained friable granite and pegmatitic granite |
Pipe Creek Pluton | 89 | 1690–1740 | Granite to granodiorite, pegmatitic in places |
Horn Creek Pluton | 90–91 | 1713 ± 2 | Foliated medium-grained hornblende quartz diorite to tonalite |
Trinity Pluton | 91.5 | 1730 ± 3 | Medium to coarse-grained biotite granodiorite to granite orthogneiss |
Sapphire Pegmatite Complex | 99–104 | undated | Granite and granitic pegmatite dikes and sills |
Ruby Pluton | 102–108 | 1716 ± 0.5 | Hornblende-biotite granodiorite, diorite and tonalite |
Elves Chasm Pluton | 112–118 | 1840 ± 1 | Lineated and foliated hornblende-biotite tonalite to quartz diorite and granodiorite |
Granite Narrows Pluton | 136 | undated | Coarse-grained friable granite and pegmatitic granite |
Diamond Creek Pluton | 216–227 | 1736 ± 1 | Granodiorite, tonalite and diorite |
As already indicated, the 1.84 Ga Elves Chasm pluton is apparently part of an older basement terrane. The next intrusive event are the 1.74 to 1.71 Ga granodiorite complexes interpreted as arc plutons based on petrologic and geochemical data (Babcock 1990). According to that interpretation these plutons formed from melting above a subducting plate, the melts rising to form large magma chambers that fed volcanic eruptions within island arcs. Karlstrom et al (2003) inferred that they were emplaced at shallow depths because of an absence of contact metamorphic aureoles, but their original shapes are not preserved. Some are now large, folded sheet-like plutons (Zoroaster, Trinity, and Ruby plutons), whereas others are massive, differentiated plutons (Diamond Creek pluton) or smaller stock-like bodies (Grapevine Camp, Pipe Creek, and Horn Creek plutons) (fig. 2). One characteristic of many of these plutons is the presence of enclaves of a range of compositions (gabbro to granodiorite) that likely record co-mingling of magmas within arc magma chambers (for example, Ruby and Diamond Creek plutons). These arc plutons are relatively rich in feldspars and hence were relatively strong during subsequent deformations.
The 1.71 to 1.66 Ga granites and granitic pegmatites have a different composition, intrusive style, and deformational character than the arc plutons (Karlstrom et al. 2003). They are biotite granite, biotite-muscovite (± Garnet) granite, and granitic pegmatite that are interpreted as probably having formed by partial melting of the lower crust during deformation. Melts evidently rose along cracks and shear zones and froze to form dikes or coalesced as small stocks and plutons. It is common for a network of granite dikes to locally make up more than half of the exposed rock volume, blurring the distinction between dike swarms and plutons and indicating these plutons coalesced from dikes. In the Upper Granite Gorge, intrusive complexes are made up of nearly equal proportions of medium-grained granite and granitic pegmatite (for example, the Cottonwood and Sapphire complexes in fig. 2). These granites are commonly stretched (boudinaged), folded, and foliated, but also occur as undeformed cross-cutting tabular dikes and orthogonal dike networks. These relationships suggest that they were intruded during regional deformation.
History and Significance of U and Po Radiohalos
Radiohalos are minute circular zones of darkening (39–70 μm in diameter) surrounding tiny central mineral inclusions or crystals within some minerals. Often concentric darkened rings are distinguishable within the darkened circular areas. While radiohalos appear in fluorite, cordierite, quartz, and to a lesser extent K-feldspars, they are best observed in thin microscope sections of the black mica, biotite, where the tiny inclusions (or radiocenters) are usually zircon crystals. The significance of radiohalos is due to them being a physical, integral historical record of the decay of radioisotopes in the radiocenters over a period of time.
First reported between 1880 and 1890, their origin was a mystery until the discovery of radioactivity. Then in 1907 Joly (1907) and Mügge (1907) independently suggested that the darkening of the minerals around the central inclusions is due to the alpha (α) particles produced by α-decays in the radiocenters. These α-particles are “fired” in all directions like “bullets” and thus damage the crystal structure of the surrounding minerals. It is known as a Frenkel defect (a type of point defect) accumulation in which smaller atoms (the α-particles are essentially helium nuclei) dislodge larger atoms from their places in the crystal lattice creating vacancies in which the smaller atoms accumulate interstitially. This process produces concentric shells of darkening or discoloration (fig. 3). When observed in thin sections these shells are concentric circles with diameters between 39 and 70 μm, simply representing planar sections through the concentric spheres centered around the inclusions (Gentry 1973).
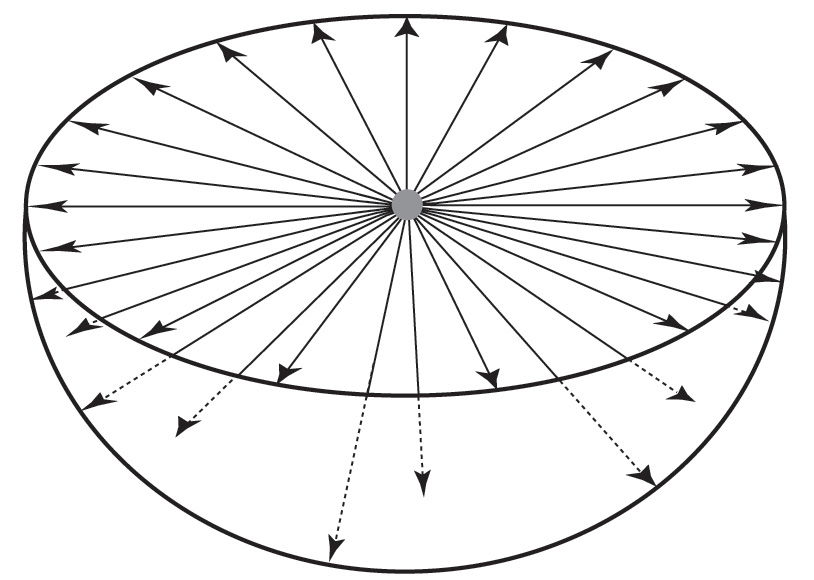
Fig. 3. Sunburst effect of α-damage trails. The sunburst pattern of α-damage trails produces a spherically colored shell around the halo radiocenter. Each arrow represents approximately 5 million α-particles emitted from the radiocenter. Radiohalo coloration initially develops after about 100 million α-decays, becomes darker after about 500 million, and very dark after about 1 billion (after Gentry 1988).
Many years of subsequent investigations have established that the radii of the concentric circles of the radiohalos as observed in thin sections are related to the α-decay energies. This enables the radioisotopes responsible for the α-decays to be identified (Gentry 1974, 1984, 1986, 1988; Snelling 2000). Most importantly, when the central inclusions, or radiocenters, are very small (about 1 μm) the radiocenters around them have been unequivocally demonstrated to be products of the α-emitting members of the 238U decay series to stable 206Pb, and occasionally the 232Th decay series to stable 208Pb. It has been demonstrated that the radii of these rings correspond to the energies of specific α-particles in the 238U decay series and their travel ranges (Gentry 1984). Thus, a fully-developed 238U radiohalo should have eight visible concentric rings which correspond to the eight α-decay steps in the 238U decay series. It has also been determined that such a halo requires between 500 million and 1 billion α-decays to be fully-developed and darkened (fig. 3) (Gentry 1988). The radii of the concentric multiple spheres, or rings in thin sections, correspond to the ranges in the host minerals of the α-particles from the α-emitting radioisotopes in the 238U and 232Th decay series (Gentry 1973, 1974, 1984) (fig. 4). 235U radiohalos have not been observed. This is readily accounted for by the scarcity of 235U (only 0.7% of the naturally-occurring U), since large concentrations of the parent radionuclides are needed to produce the concentric ring structures of the radiohalos.
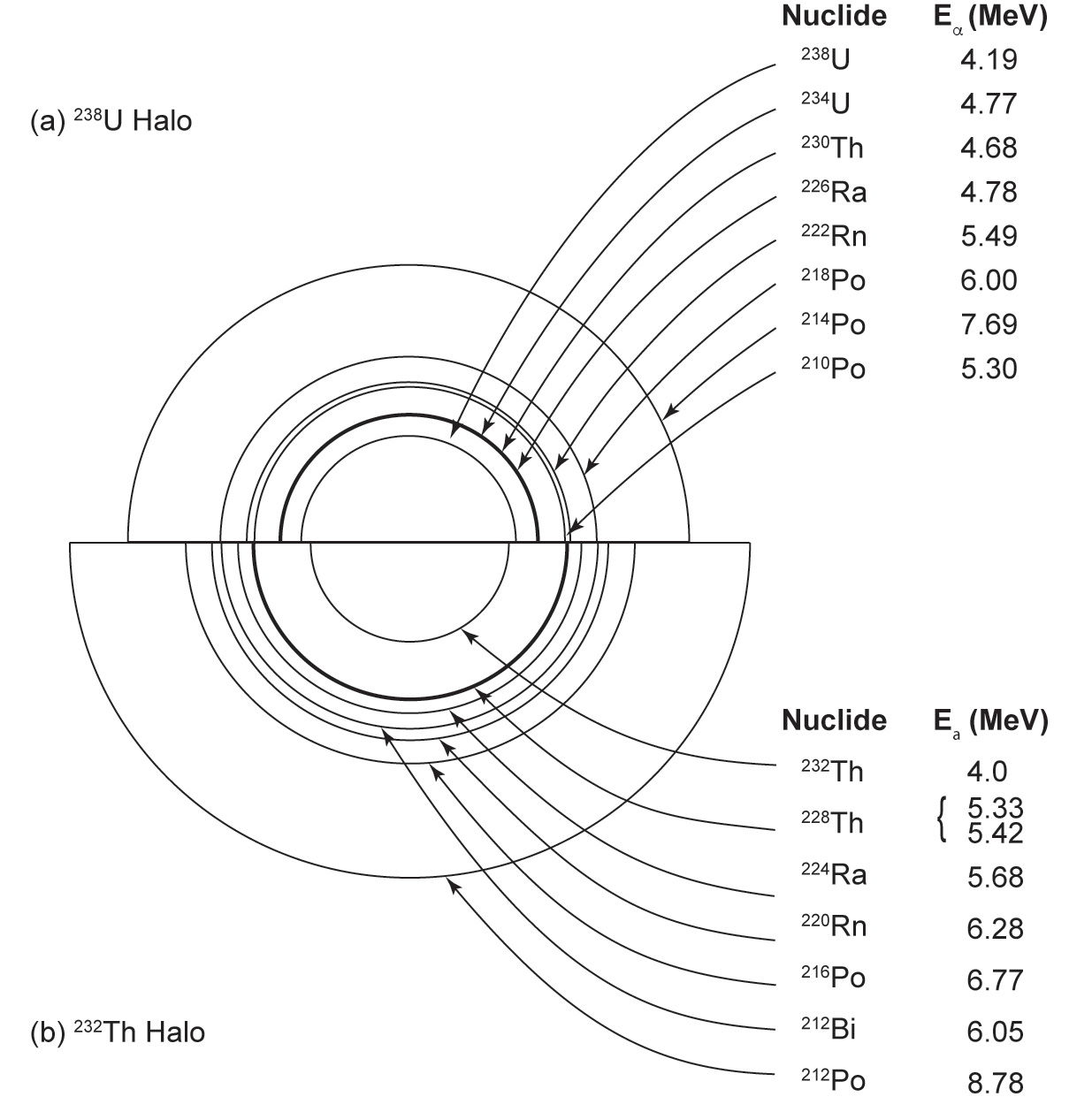
Fig. 4. Schematic drawing of (a) a 238U radiohalo, and (b) a 232Th radiohalo, with radii proportional to the ranges of α-particles in air. The nuclides responsible for the α-particles and their energies are listed for the different halo rings (after Gentry 1973).
Ordinary radiohalos can be defined, therefore, as those that are initiated by 238U or 232Th α-decay, irrespective of whether the actual halo sizes closely match the respective idealized patterns (fig. 4). In many instances the match is very good, the observed sizes agreeing very well with the 4He ion penetration ranges produced in biotite, fluorite, and cordierite (Gentry 1973, 1974).
U and Th radiohalos usually are found in igneous rocks, most commonly in granitic rocks and in granitic pegmatites. While U and Th radiohalos have been found in over 40 minerals, their distribution within these minerals is very erratic (Ramdohr 1933, 1957, 1960; Stark 1936). Thus far there have been no hypotheses proposed to explain the cause(s) of this erratic distribution. Radiohalos have even been found in diamonds (Armitage and Snelling 2008; Gentry 1998; Schulze and Nasdala 2017). Biotite is quite clearly the major mineral in which U and Th radiohalos occur (Bower et al. 2016a, b). Wherever found in ubiquitous large (1–5 mm in diameter) biotite flakes the radiohalos are prolific and are associated with tiny (1–5 μm in diameter) U-bearing zircon grains or Th-bearing monazite grains as the radiocenters. The ease of thin section preparation, and the clarity of the radiohalos in these sections, have made biotite an ideal choice for numerous radiohalo investigations, namely, those of Joly (1917a, b, 1923, 1924), Lingen (1926), Iimori and Yoshimura (1926), Kerr-Lawson (1927, 1928), Wiman (1930), Henderson and Bateson (1934), Henderson and Turnbull (1934), Henderson, Mushkat and Crawford (1934), Henderson and Sparks (1939), Gentry (1968, 1970, 1971), Snelling and Armitage (2003), and Snelling (2005). U, Th, and other specific halo types in most of these studies have been observed mainly in Precambrian rocks, so much remains to be learned about their occurrence in rocks from the other geological systems of the rock record. However, some studies have shown that they do exist in rocks stretching from the Precambrian to the Tertiary (Paleogene and Neogene) (Holmes 1931; Snelling 2000, 2005, 2023b; Snelling and Armitage 2003; Stark 1936; Wise 1989). Unfortunately, in some of those published studies the radiohalo types were not specifically identified.
Some unusual radiohalo types that appear to be distinct from those formed by 238U and/or 232Th α-decay have been observed (Gentry 1970, 1971, 1973, 1984, 1986; Gentry et al. 1973, 1976, 1978; Snelling 2000). Of these, only the Po (polonium) radiohalos can presently be identified with known α-radioactivity (Gentry 1967, 1968, 1973, 1974; Gentry et al. 1973, 1974). There are three Po isotopes in the 238U-decay chain. In sequence they are 218Po (half-life of 3.1 minutes), 214Po (half-life of 164 microseconds), and 210Po (half-life of 138 days). Po radiohalos contain only three rings, two rings or the one ring produced by these three Po α-emitters respectively (fig. 5). They are designated by the first (or only) Po α-emitter in the portion of the decay sequence that is represented. The presence in Po radiohalos of only the rings of the three Po α-emitters implies that the radiocenters which produced these Po radiohalos initially contained only either the respective Po radioisotopes that then parented the subsequent α-decays, or a non-α-emitting parent (Gentry 1971; Gentry et al. 1973). These three Po radiohalo types occur in biotite from granitic rocks (Gentry 1968, 1971, 1973, 1974, 1984, 1986, 1988; Gentry et al. 1973, 1974; Wise 1989; Snelling and Armitage 2003; Snelling 2005). Some typical radiohalos in biotite are shown in fig. 6.
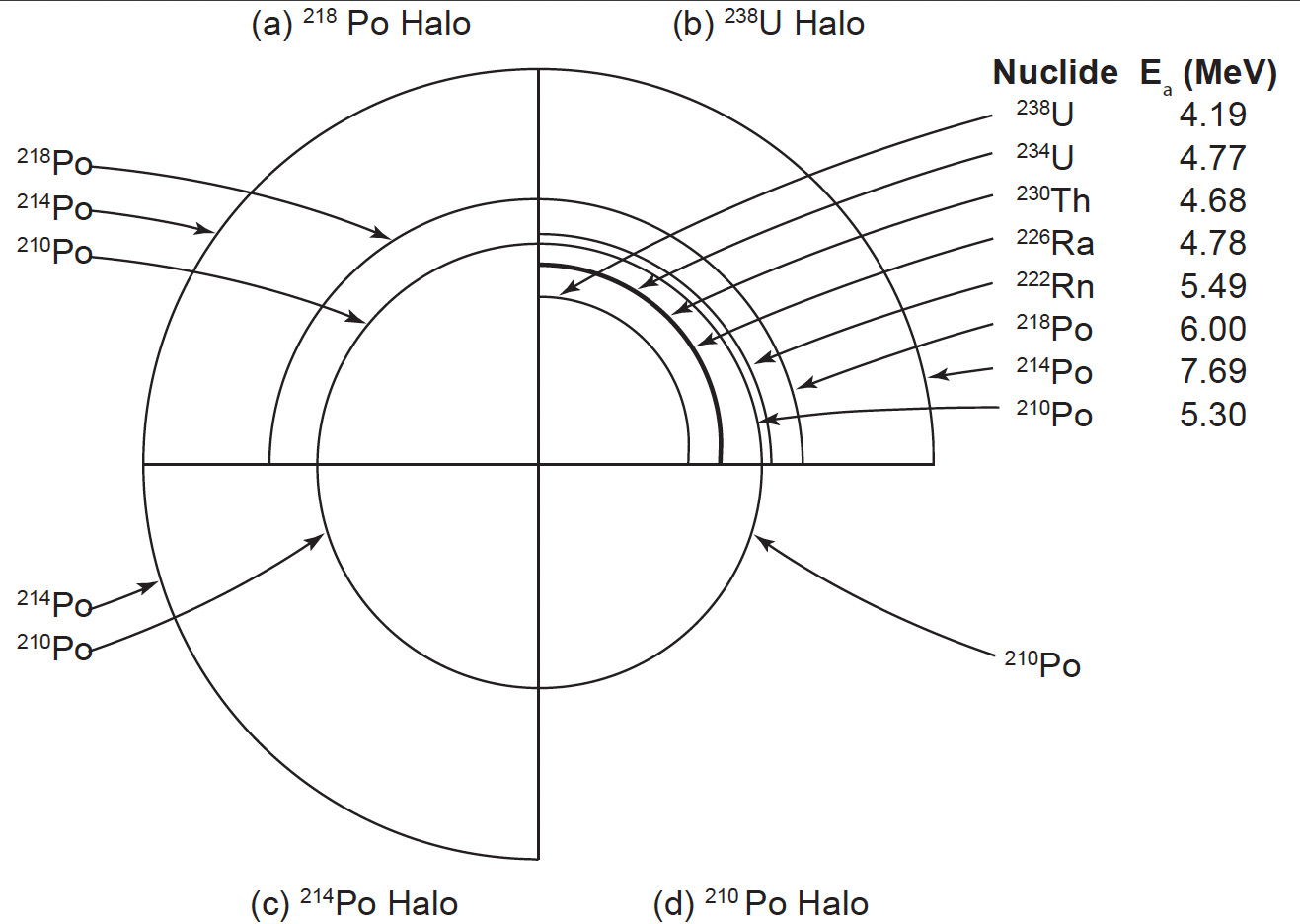
Fig. 5. Composite schematic drawing of (a) a 218Po radiohalo, (b) a 238U radiohalo, (c) a 214Po radiohalo, and (d) a 210Po radiohalo, with radii proportional to the ranges of α-particles in air. The nuclides responsible for the α-particles and their energies are listed for the different halo rings (after Gentry 1973).
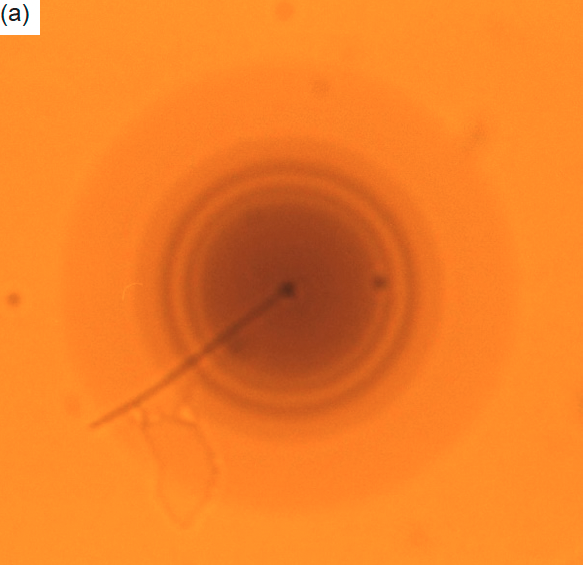
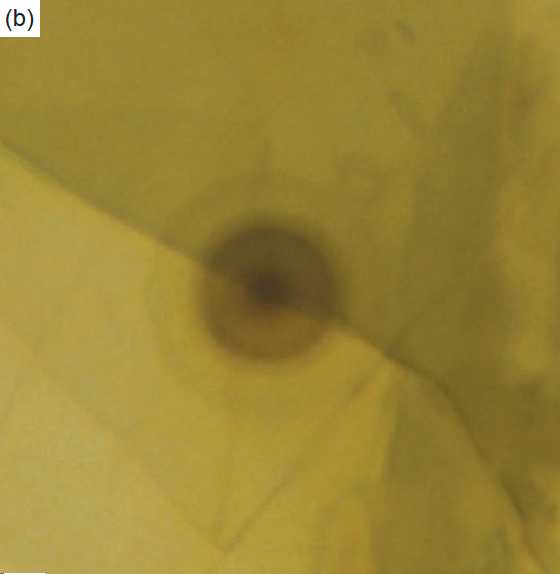
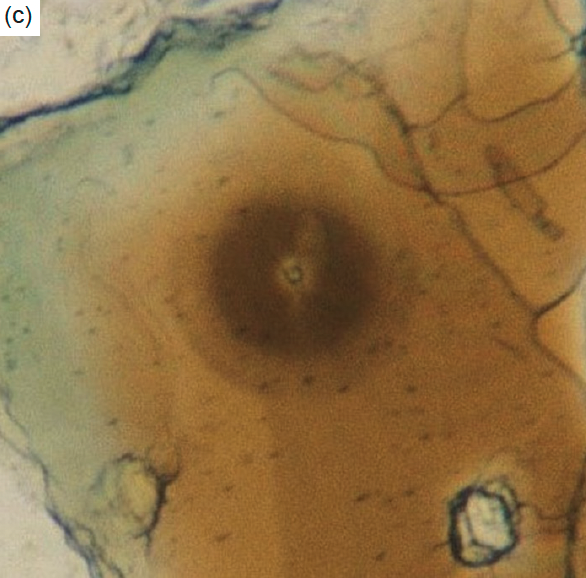
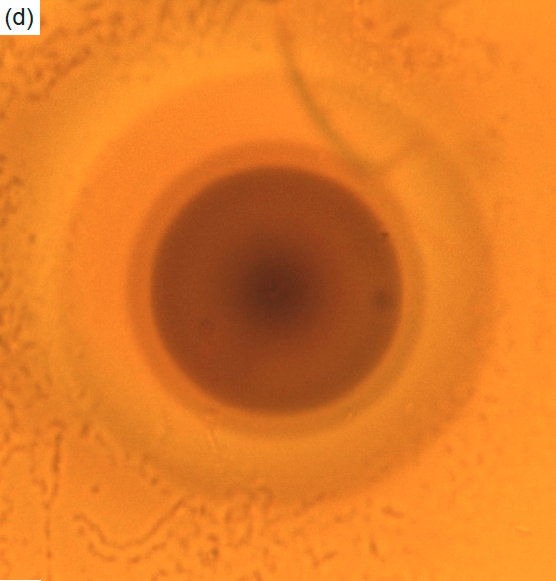
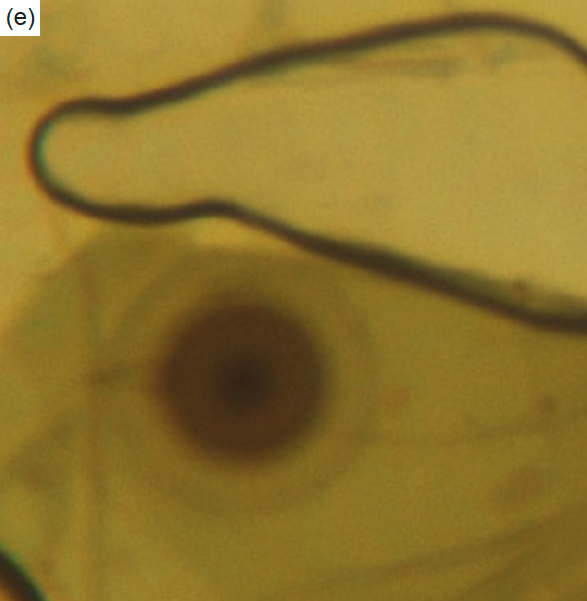
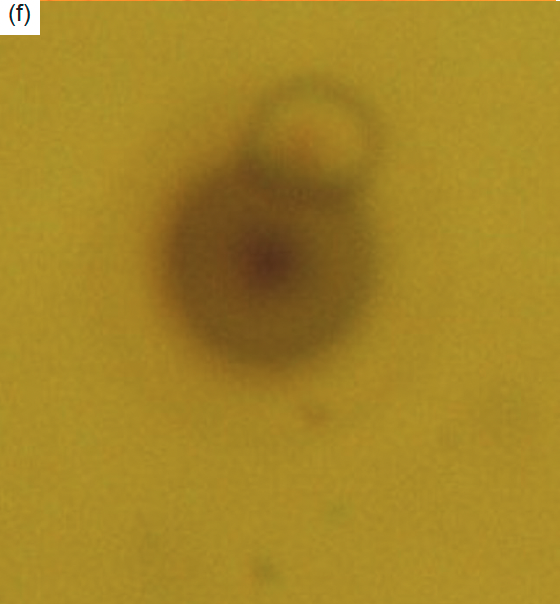
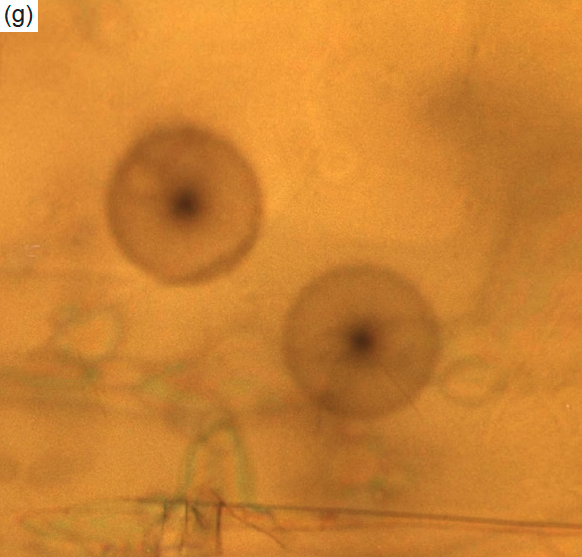
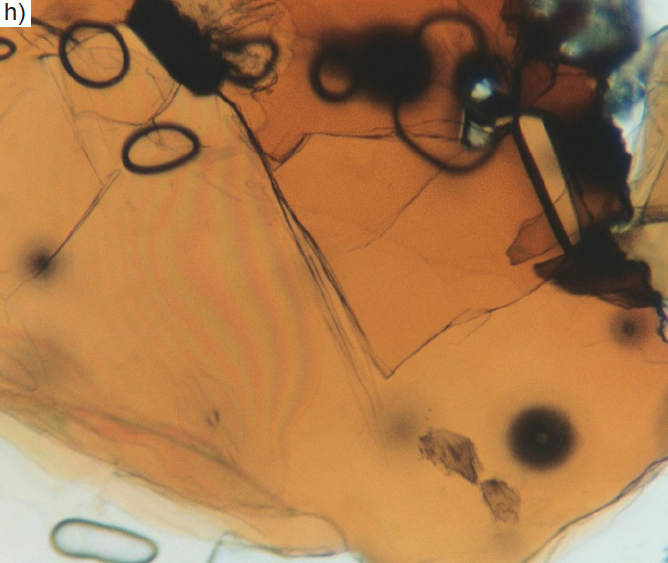
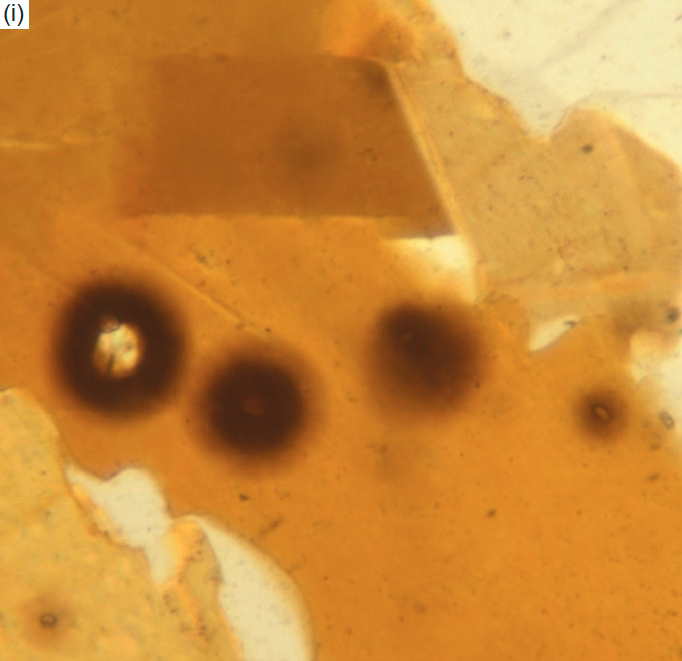
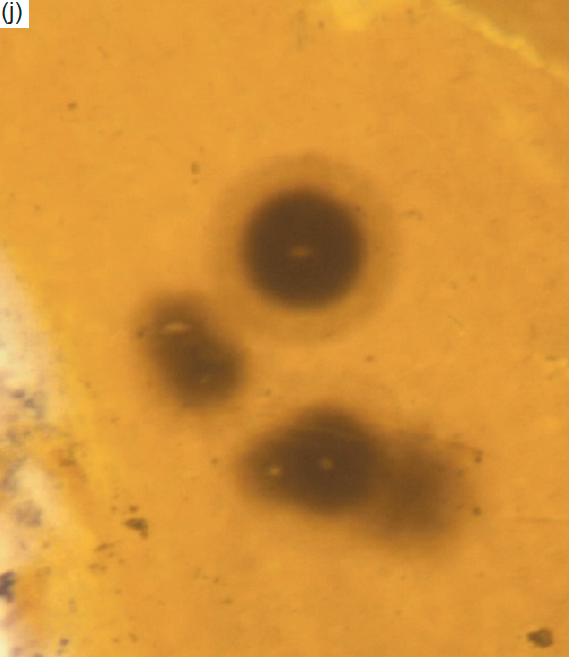
Fig. 6. Some typical examples of the different radiohalos found in granites and metamorphic rocks. All images are in focus, but where the radiohalos appear fuzzy it is due to faintness of the radiation damage or to the intense radiation damage broadening the outlines and the rings: (a) a 238U radiohalo with well-defined rings; (b) a 238U radiohalo; (c) an overexposed 238U radiohalo; (d) a 218Po radiohalo with well-defined rings; (e) a 218Po radiohalo; (f) a 214Po radiohalo; (g) two well-defined 210Po radiohalos; (h) an overexposed 238U radiohalo (bottom right), a 214Po radiohalo (center left), and a 210Po radiohalo (center right); (i) an extremely overexposed 238U radiohalo due to a large zircon crystal as its radiocenter (left), another overexposed 238U radiohalo (left of center), and a 214Po radiohalo (right); and (j) two 238U radiohalos with overexposed inner rings and three overexposed 210Po radiohalos. Scales: 238U radiohalo diameter ≈ 70 μm, 218Po radiohalo diameter ≈ 70 μm, 214Po radiohalo diameter ≈ 68 μm, and 210Po radiohalo diameter ≈ 39 μm.
Joly (1917b, 1924) was probably the first to investigate 210Po radiohalos and was clearly baffled by them. Because Schilling (1926) saw Po radiohalos located only along cleavages and cracks in fluorite from Wölsendorf in Germany, he suggested that they originated from preferential deposition from secondary fluid transport of Po in U-bearing solutions. Henderson (1939) and Henderson and Sparks (1939) invoked a similar but more quantitative hypothesis to explain Po radiohalos along conduits in biotite. However, those Po radiohalos found occurring along much more restricted cleavage planes, similar to those found by Gentry (1973, 1974), have been more difficult to account for. The reason given for these attempts to account for the origin and formation of the Po radiohalos by some secondary process is simple—the half-lives of the respective Po isotopes are far too short to be reconciled with the Po having been primary, that is, originally in the granitic magmas which are usually claimed to have slowly cooled to form the granitic rocks that now contain the Po-radiohalo-bearing biotite grains. The half-life of 218Po, for example, is 3.1 minutes. However, this is not the only formidable obstacle for any secondary process that transported the Po into the biotite as, or after, the granitic rocks cooled. First, there is the need for the isotopic separation of the Po isotopes, or their α-decay precursors, from their parent 238U having occurred naturally (Gentry et al. 1973). Second, the radiocenters of very dark 218Po radiohalos, for example, may need to have contained as much as 5 × 109 atoms (a concentration of greater than 50%) of 218Po (Gentry 1974), yet the host minerals contain only ppm abundances of 238U, which apparently means only a negligible supply of 218Po daughter atoms is available for capture in a radiocenter at any given time. But these 218Po atoms must also migrate or diffuse from their source at very low diffusion rates through surrounding mineral grains to be captured by the radiocenters before the 218Po decays (Fremlin 1975; Gentry 1968, 1975).
Therefore, there are strict time limits for the formation of the Po radiohalos by primary or secondary processes in granites. Studies of some Po radiohalo centers in biotite (and fluorite) have shown little or no U in conjunction with anomalously high 206Pb/207Pb and/or Pb/U ratios, which would be expected from the decay of Po without the U precursor that normally occurs in U radiohalo centers (Gentry 1974; Gentry et al. 1974). Indeed, many 206Pb/207Pb ratios were greater than 21.8 reflecting a seemingly abnormal mixture of Pb isotopes derived from Po decay independent of the normal U-decay chains (Gentry 1971; Gentry et al. 1973). Thus, based on these data, Gentry advanced the hypothesis that the three different types of Po radiohalos in biotite represent the decay of primordial Po (that is, original Po not derived by 238U-decay), and that the rocks hosting these radiohalos must be primordial rocks produced by fiat creation, given that the half-life of 214Po is only 164 microseconds (Gentry 1979, 1980, 1982, 1983, 1984, 1986, 1988, 1989). He thus perceived that all granites must be Precambrian, and part of the earth’s crust created during the Creation Week.
As a consequence of Gentry’s creation hypothesis, the origin of the Po radiohalos has remained controversial and thus apparently unresolved. Of the 22 locations then known where the rocks contained Po radiohalos, Wise (1989) determined that six of the locations hosted Phanerozoic granitic rocks that intruded fossiliferous (and thus Flood-deposited) sedimentary strata, a large enough proportion to severely question Gentry’s hypothesis of primordial Po in fiat created granitic rocks. Many of these Po radiohalo occurrences are also in proximity to higher than normal U concentrations in nearby rocks and/or minerals, suggesting ideal sources for fluid separation and transport of the Po. Subsequently, Snelling (2000) reviewed the literature on radiohalos. He thoroughly discussed the many arguments and evidences used in the debate that had ensued over the previous two decades and concluded that there were insufficient data on the geological occurrence and distribution of the Po radiohalos for the debate to be decisively resolved. He then recognized that the spatial association of Po radiohalos to 238U radiohalos meant that the Po which parented the adjacent Po radiohalos may have been derived from the 238U decay products in the radiocenters of the 238U radiohalos. He also observed that many radiohalo-hosting biotite flakes had been hydrothermally altered. Furthermore, many of the host Phanerozoic granites had intruded into fossil-bearing sedimentary layers that therefore were deposited during the Flood. Thus, those and other granites intruded into fossil-bearing, Flood-deposited sedimentary layers had to form during the Flood subsequent to the deposition of those sedimentary layers and could not be creation rocks as postulated by Gentry (1988). Of course, this does not preclude many Precambrian granites having been created during the Creation Week.
Furthermore, Snelling (2000) found that there are now significant reports of 210Po as a detectable species in volcanic gases, in volcanic/hydrothermal fluids associated with subaerial volcanoes and fumaroles and associated with mid-ocean ridge hydrothermal vents and chimney deposits (Hussain et al. 1995; LeCloarec et al. 1994; Rubin 1997), as well as in ground waters (Harada et al. 1989; Larock et al. 1996). The distances involved in this fluid transport of the Po are several kilometers (and more), so there is increasing evidence of the potential viability of the secondary transport of Po by hydrothermal fluids during pluton emplacement, perhaps in the waning stages of the crystallization and cooling of granitic magmas (Snelling 2000, 2008a; Snelling and Woodmorappe 1998).
Consequently, Snelling and Armitage (2003) investigated the Po radiohalo occurrences in three Phanerozoic granitic plutons and logically argued for a model of Po radiohalo formation involving secondary transport of Po by hydrothermal fluids during crystallization and cooling of the granitic magmas. Their data and details of this hydrothermal fluid transport model were initially published by Snelling, Baumgardner, and Vardiman (2003), but full details encompassing these results were elaborated upon in Snelling (2005). He proposed that hydrothermal fluids infiltrating along the cleavage planes within biotite flakes dissolved 226Ra, 222Rn, and the Po isotopes emanating from 238U decay within the zircon radiocenters of the 238U radiohalos. At conducive sites downflow within the same biotite flakes the Po isotopes were deposited and concentrated in what became the radiocenters for 218Po, 214Po, and 210Po radiohalos as the Po isotopes decayed. Hydrothermal fluids are typically Cl-rich and are known to be capable of dissolving 226Ra, 222Rn, and the Po isotopes, the latter particularly bonding with Cl (Bagnall 1957). Hydrothermal fluids also carry S, and because Po behaves geochemically the same as Pb it also bonds with S (Bagnall 1957). Furthermore, the mica sheets making up the biotite structure are weakly bonded by K, OH, and F ions, so S and Cl ions can occasionally substitute at point loci within the cleavage planes. It was thus postulated by Snelling (2005) that as the hydrothermal fluids carrying 222Rn and the Po along the cleavage planes between the biotite sheets, Po atoms were attracted to those point loci where they decayed, only to be replaced by more Po atoms attracted to the same S or Cl point loci. The Po radiocenters were thus formed surrounded by Po radiohalos.
Whereas Po radiohalos would appear to indicate extremely rapid geological processes were responsible for their production (because of the extremely short half-lives of the Po isotopes responsible), 238U and 232Th radiohalos appear to be evidence of long periods of radioactive decay, assuming decay rates have been constant at today’s rates throughout earth history. Indeed, it has been estimated that dark, fully-formed U and Th radiohalos require around 100 million years’ worth of radioactive decay at today’s rates to form (Gentry 1973, 1974; Humphreys 2000; Snelling 2000). Thus, the presence of mature U and Th radiohalos in granitic rocks globally throughout the geological record would indicate that at least 100 million years’ worth of radioactive decay at today’s rates had occurred. Therefore, the requirement of grossly accelerated 238U decay is essential to this hydrothermal fluid model for the transport of the Po isotopes from the decay of 238U in the radiocenters of 238U radiohalos (Snelling 2005). Vardiman, Snelling, and Chaffin (2005) found that the greater the half-life of a radioisotope the greater the decay rate acceleration. Thus, whereas 238U decay would have been grossly accelerated during some past geologically catastrophic event such as the Flood, the very short half-life Po radioisotopes would not have been appreciably affected. Several lines of evidence suggest that during the Flood when much of the fossil-bearing sedimentary rock record was accumulating, and when biotite-bearing granites were intruded into those sedimentary rocks, the decay rate of 238U was grossly accelerated (Vardiman, Snelling, and Chaffin 2005). These include systematically different radioisotope ages for the same rock units dated by multiple methods, helium diffusion in zircons, the quantities of fission tracks matching conventional Phanerozoic stratigraphic ages in tuff beds deposited during the Flood year, and radiocarbon in Phanerozoic coal beds and other organic materials, as elaborated in detail by Vardiman, Snelling, and Chaffin (2005). Thus, whereas today’s very slow 238U decay rate produces only a few Ra, Rn, and Po atoms very slowly, that grossly accelerated decay rate would have produced huge numbers of Ra, Rn, and Po atoms very rapidly, which were then easily transported the short distances within the host biotite flakes to precipitate in the adjacent Po radiocenters and produce the Po radiohalos.
Constrained by the biblical timescale, Humphreys (2000) and Vardiman, Snelling, and Chaffin (2005) proposed that these observable data require radioisotope decay to have been accelerated, but just by how much needs to be determined. If, for example, mature U and Th radiohalos were found in granitic rocks that were demonstrated to have formed during the Flood year, then that would imply at least 100 million years’ worth of radioisotope decay at today’s rates had occurred at an accelerated rate during the Flood year (Baumgardner 2000; Snelling 2000, 2005). Vardiman, Snelling, and Chaffin (2005) postulated the 238U decay rate was accelerated by five orders of magnitude, so it could then be supposed the Po isotopes’ decay rates were similarly accelerated, which could make their existence so fleeting there wouldn’t be sufficient time for hydrothermal transport to form radiocenters. However, as already noted, Vardiman, Snelling, and Chaffin (2005) found that the amount of acceleration was related to the present half-lives of the parent radioisotopes, the slower the present decay rate (or the longer the current half-life) resulting in the most acceleration. Thus, with such fast decay rates (short half-lives) today, the Po isotopes would scarcely have been accelerated at all. Furthermore, the accelerated decay rates would not have resulted in much larger radii for 238U radiohalos, as ring radii are not affected by the decay rates but are related to the energies of the emitted α-particles (Gentry 1973, 1974).
In this hydrothermal model, the Po accumulated in the radiocenters by time integration, as Po atoms were progressively deposited from the passing hydrothermal fluids (Snelling 2005; Snelling and Armitage 2003). So, instead of the Po radiohalos forming virtually instantaneously as proposed by Gentry (1988), the Po radiohalos formed over hours and days. However, whereas granitic magmas are intruded at 650–730°C, the radiohalos cannot form until the magma has crystallized and the temperature has dropped below 150°C, because above that temperature the α-tracks that produce the radiohalos are thermally annealed (Laney and Laughlin 1981).Yet hydrothermal fluids probably began transporting Rn and the Po isotopes immediately after they were expelled from the crystallized granite at temperatures below 400°C. This has drastic time implications for the formation of granites (Snelling 2008a). Whereas Gentry (1988) concluded that granites were created instantaneously by divine fiat, Snelling (2005, 2008a, 2014a) postulated that granite magmas crystallized and cooled within 6–10 days, which is still very radical compared to the uniformitarian timescale.
Snelling, Baumgardner, and Vardiman (2003) and Snelling (2005) have summarized this model for hydrothermal fluid transport of U-decay products (Rn, Po) in a six-step diagram. The final step concludes with the comment:
With further passing of time and more α-decays both the 238U and 210Po radiohalos are fully formed, the granite cools completely and hydrothermal fluid flow ceases. Note that both radiohalos have to form concurrently below 150°C. The rate at which these processes occur must therefore be governed by the 138 day half-life of 210Po. To get 218Po and 214Po radiohalos these processes would have to have occurred even faster. (Snelling 2005)
Furthermore, if Po radiohalos were alongside U radiohalos in the same granitic rocks, then that would have implications as to the rate of formation and age of these granitic rocks formed during the Flood year within the biblical timescale. If the U and Po radiohalos both mostly formed during the 6–10 days while the granite plutons cooled during the Flood, then this implies 100 million years’ worth of accelerated 238U decay occurred in a time frame of days to a few weeks. Thus the U-Pb isotopic systematics within the zircons in these granite plutons are definitely not providing absolute “ages” as conventionally interpreted.
Subsequently, case studies were undertaken to test this hydrothermal fluid transport model for the formation of Po radiohalos. Most remarkable was the fulfilled prediction of many more Po radiohalos at the staurolite isograd in regionally metamorphosed sandstones in the Great Smoky Mountains, Tennessee-North Carolina, where the metamorphic reaction would have released a lot of water (224 water molecules for every 54 units of muscovite reacting with chlorite; Snelling 2008b). Then, in the Cooma regional metamorphic complex of southeastern Australia the numbers of Po radiohalos increased where water was released in the high-grade zone and in the central granodiorite but decreased sharply in the zone of partial melting where water was dissolved into the melt, just as expected in the model (Snelling 2008c).
In granites, increased numbers of Po radiohalos were also found where they were predicted to be based on the release of hydrothermal fluids during granite crystallization and cooling (Snelling 2008a). In the Shap Granite of northern England, prolific Po radiohalos matched the higher volume of hydrothermal fluids associated with that granite’s large K-feldspar phenocrysts (Glazner and Johnson 2013; Snelling 2008d). The nested plutons of the Tuolumne Intrusive Suite, Yosemite, California (Glazner et al. 2022) contain increasing numbers of Po radiohalos proportional to the increased volumes of active hydrothermal fluids within the sequentially emplaced intrusions (Snelling and Gates 2009). High numbers of Po radiohalos and active hydrothermal fluids coincide with the large K-feldspar phenocrysts in the second to last pluton and the connection to explosive volcanism of the last pluton (Bateman and Chappell 1979; Glazner and Johnson 2013; Glazner et al. 2022; Snelling and Gates 2009). The Bathurst Batholith west of Sydney, Australia, consists of an enormous pluton of 1600 km2 (620 sq. mi) (the Bathurst Granite) intruded into fossiliferous sedimentary strata and numerous smaller related satellite plutons and dikes, which field and textural data have established were sequentially intruded while still hot (Snelling 2014a). The presence of Po radiohalos in all three sequentially-intruded granite phases is evidence that all this intrusive activity, and the cooling of all three granite phases, must have occurred within a week or two so that these Po radiohalos in them formed subsequently within days to weeks. And Snelling (2018) successfully tested the use of Po radiohalos as an exploration guide to locate hydrothermal ore deposits associated with granites, higher numbers of Po radiohalos being spatially associated with hydrothermal ore veins within granites in the New England region of northern New South Wales, Australia.
Finally, Snelling (2023b) tabulated the radiohalos data he had amassed from two decades of radiohalos research on samples of numerous granites and metamorphic rocks from locations around the globe of various geological ages, both Precambrian and Phanerozoic. His data clearly showed the peak occurrence of radiohalos was in Flood-related rocks when hydrothermal fluids would be prevalent during catastrophic geological processes. Yet Po radiohalos were still present, albeit in small numbers, within some Precambrian (pre-Flood) granites. Given the observation that radiohalos are annealed at 150°C (Laney and Laughlin 1981), Snelling (2023b) thus proposed any earlier pre-Flood radiohalos in those rocks may well have been annealed in the deformation of continental rocks during Creation Day Three when God caused the waters on the earth’s surface to be gathered together into one place so that the dry land was formed (Genesis 1:9) and/or at the onset of and during the Flood. If that were the case, then the radiohalos observed in today’s pre-Flood rocks were then generated subsequently during the Flood.
Field and Laboratory Methods
Field Procedure
During several raft trips spaced over almost two decades samples of granites in the inner gorges of Grand Canyon were systematically collected with research and collecting permits approved by the Grand Canyon National Park. Suitable outcrops were chosen along the Colorado River corridor and samples were collected from many granite plutons and granitic pegmatite complexes approximately every half to one mile. Samples were collected using a steel chisel and heavy-duty hammer. Some typical outcrops are shown in fig. 7. Location details for each sample were recorded with a hand-held Garmin GPS unit as well as being plotted on maps of the Colorado River corridor. Their locations are listed in table 2, measured in river miles downstream from Lees Ferry.
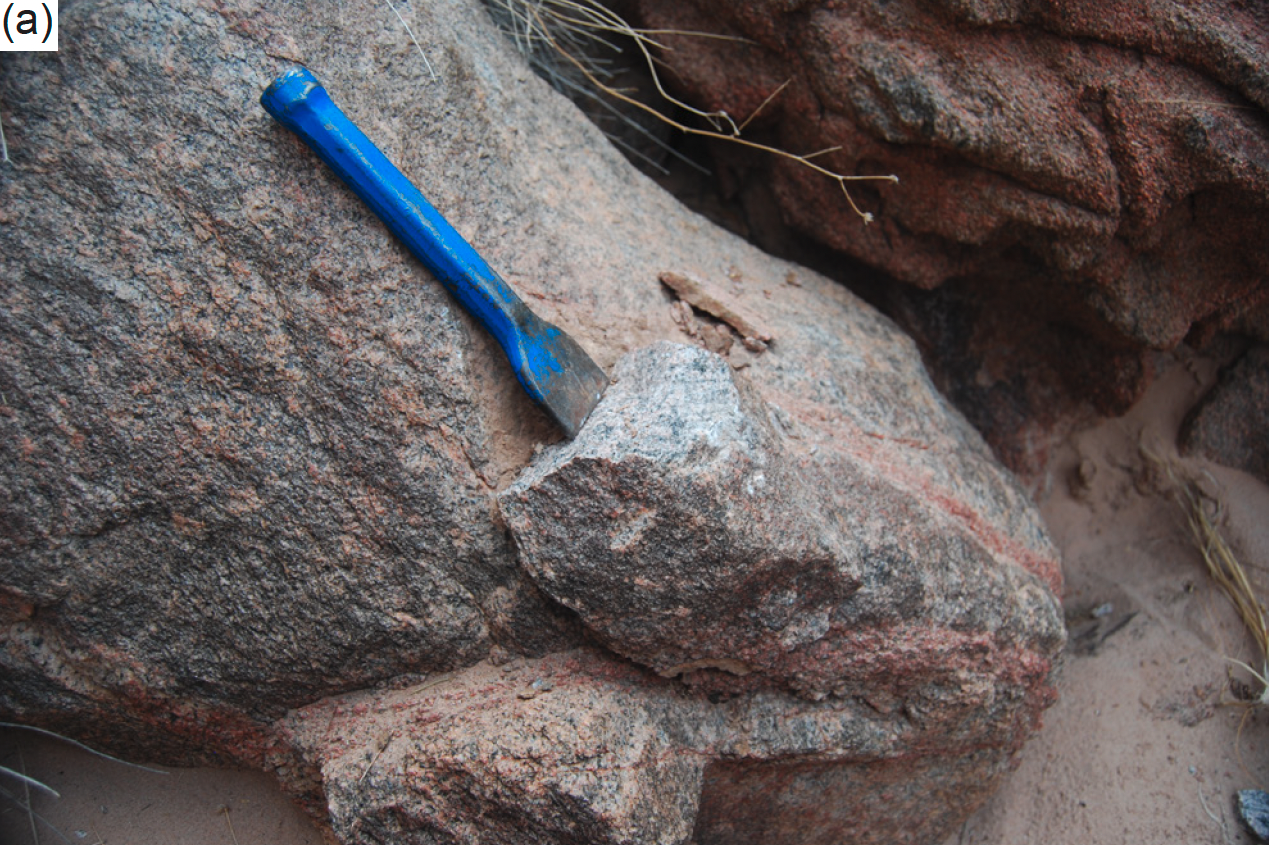
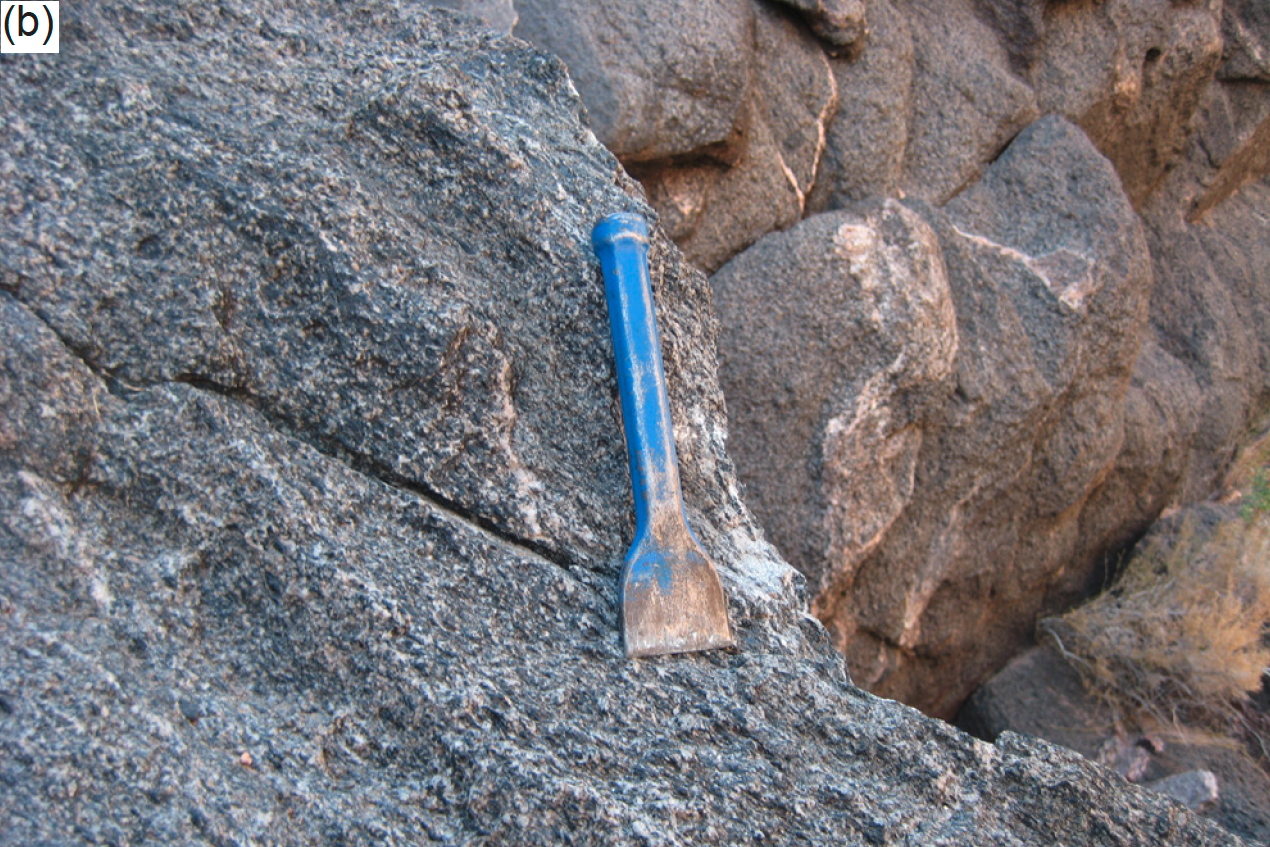
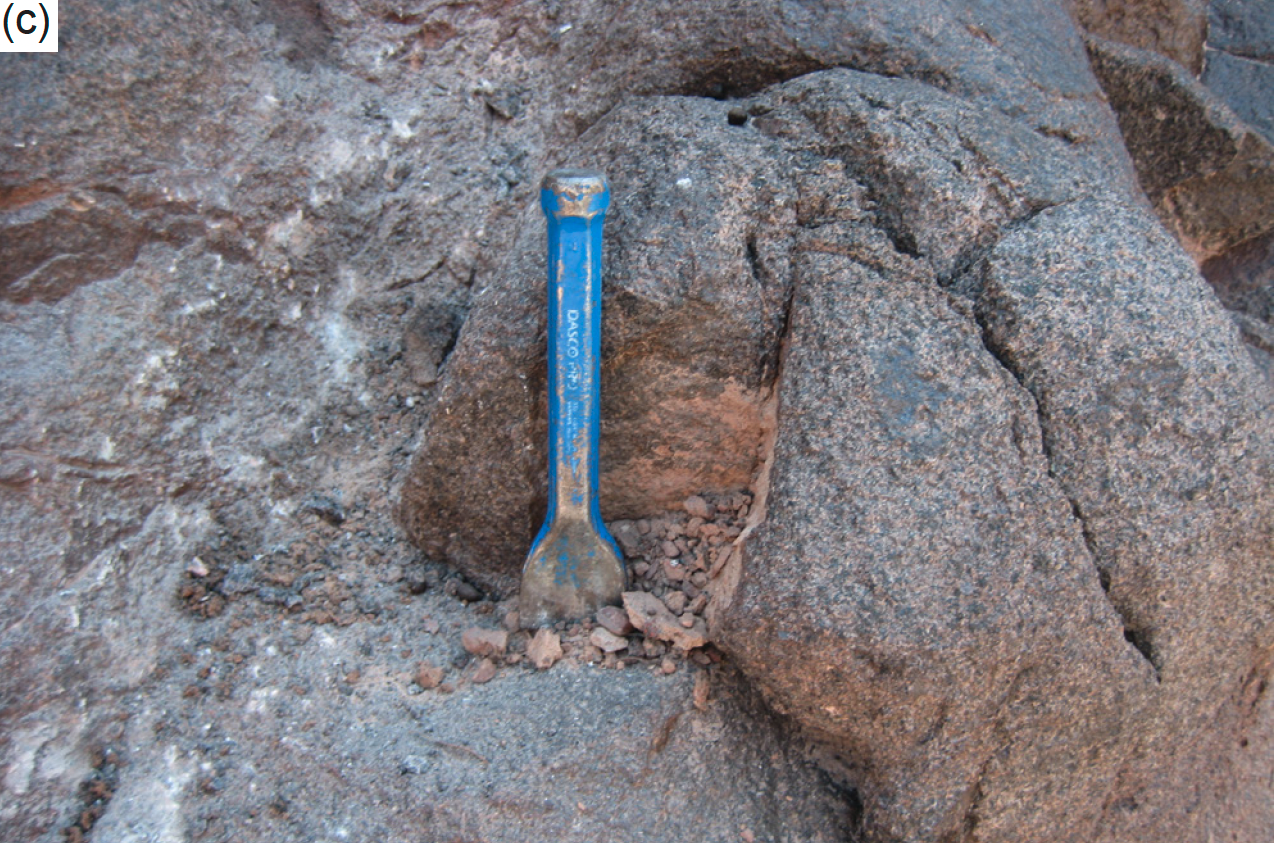
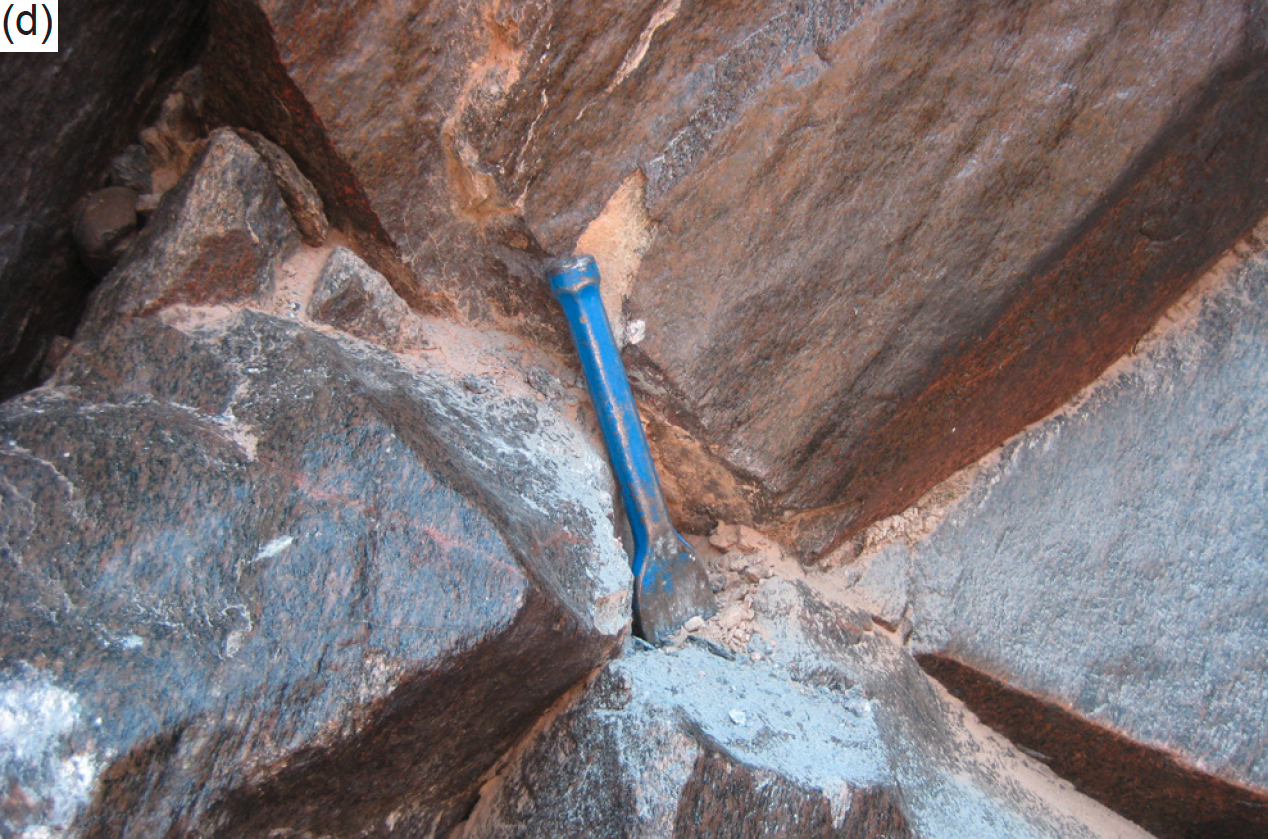
Fig. 7. Some typical outcrops from which samples were collected: (a) Horn Creek pluton, sample GCG-37, river mile 89.3; (b) Ruby pluton, sample GCG-41, river mile 103.5; (c) Ruby pluton, sample GCG-45, river mile 106.5; and (d) Elves Chasm pluton, sample GCG-46, river mile 113.0. The steel chisel which is ~9 in (~23 cm) long provides the scale.
Table 2. Radiohalos counted in each sample from the Precambrian granite plutons and pegmatite complexes in the Upper, Middle and Lower Granite Gorges.
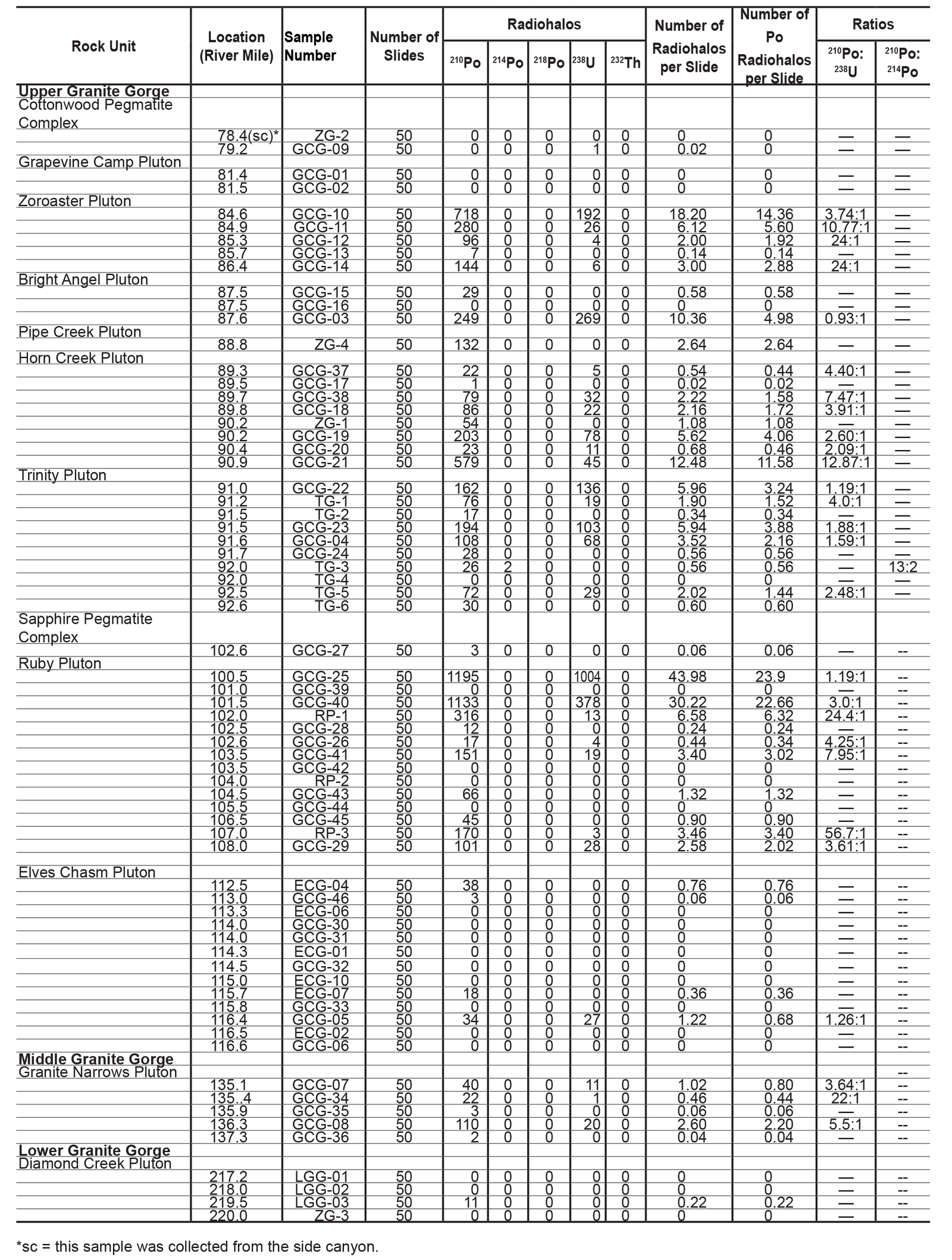
Laboratory Procedure
Snelling and Armitage (2003) and Snelling (2005) devised a method of counting radiohalos for each sample investigated from a designated number of thin sections (usually 50) with approximately 20 biotite flakes per thin section. This allowed for statistical comparisons between samples and rock types. Thus, for this study it was necessary to follow that same procedure to tabulate the needed radiohalos data for these rock samples, which also enables the data to be compared with samples from other rock units from the larger geologic record.
A standard petrographic thin section was obtained for each sample. In the laboratory, a scalpel and tweezers were used to pry flakes of large, primary biotite loose from the sample surfaces, or where necessary portions of the samples were crushed to liberate the constituent mineral grains. Biotite flakes were then handpicked with tweezers from each crushed sample and placed on a piece of Scotch tape™ fixed to the flat surface of a laminated board on a laboratory table with its adhesive side up. Once numerous biotite flakes had been mounted on the adhesive side of this piece of tape, a fresh piece of Scotch tape™ was placed over them and firmly pressed along its length so as to ensure that the two pieces were stuck together with the biotite flakes firmly wedged between them. The upper piece of tape was then peeled back in order to pull apart the sheets composing the biotite flakes, and this piece of tape with thin biotite sheets adhering to it was then placed over a standard glass microscope slide, adhesive side down, so that the thin mica flakes were held rigidly between the slide and the tape. This procedure was repeated with another piece of Scotch tape™ placed over the original tape and biotite flakes affixed to the board, the adhering biotite flakes being progressively pulled apart and transferred to microscope sides. As necessary, further handpicked biotite flakes were added to replace those fully pulled apart. In this way tens of microscope slides were prepared for each sample, each with many (at least 20 to 30) thin biotite flakes mounted on it. This is similar to the method pioneered by Gentry (1988). A minimum of 50 microscope slides was prepared for each sample (at least 1,000 biotite flakes) to ensure good representative sampling statistics.
Each thin section for each sample was then carefully examined under a petrological microscope in plane polarized light, and all radiohalos present were identified, noting any relationships between the different radiohalo types (238U, 232Th, 218Po, 214Po, and 210Po). The numbers of each type of radiohalo in each slide were counted by progressively moving the slide backwards and forwards across the field of view, and the numbers recorded for each slide were then tallied and tabulated for each sample. Because of the progressive peeling apart of many of the same biotite flakes during the preparation of the microscope slides due to biotite’s perfect basal cleavage, many of the radiohalos appeared on more than one microscope slide. Only radiohalos whose radiocenters were clearly distinguishable were counted. This procedure ensured that each radiohalo was counted only once.
Results
Fig. 8 provides images at bench scale (~25 mm wide by ~65 mm long) of the thin sections of samples of some of the granite plutons and pegmatite complexes. These illustrate the variability in the mineralogy and textures of these granites.
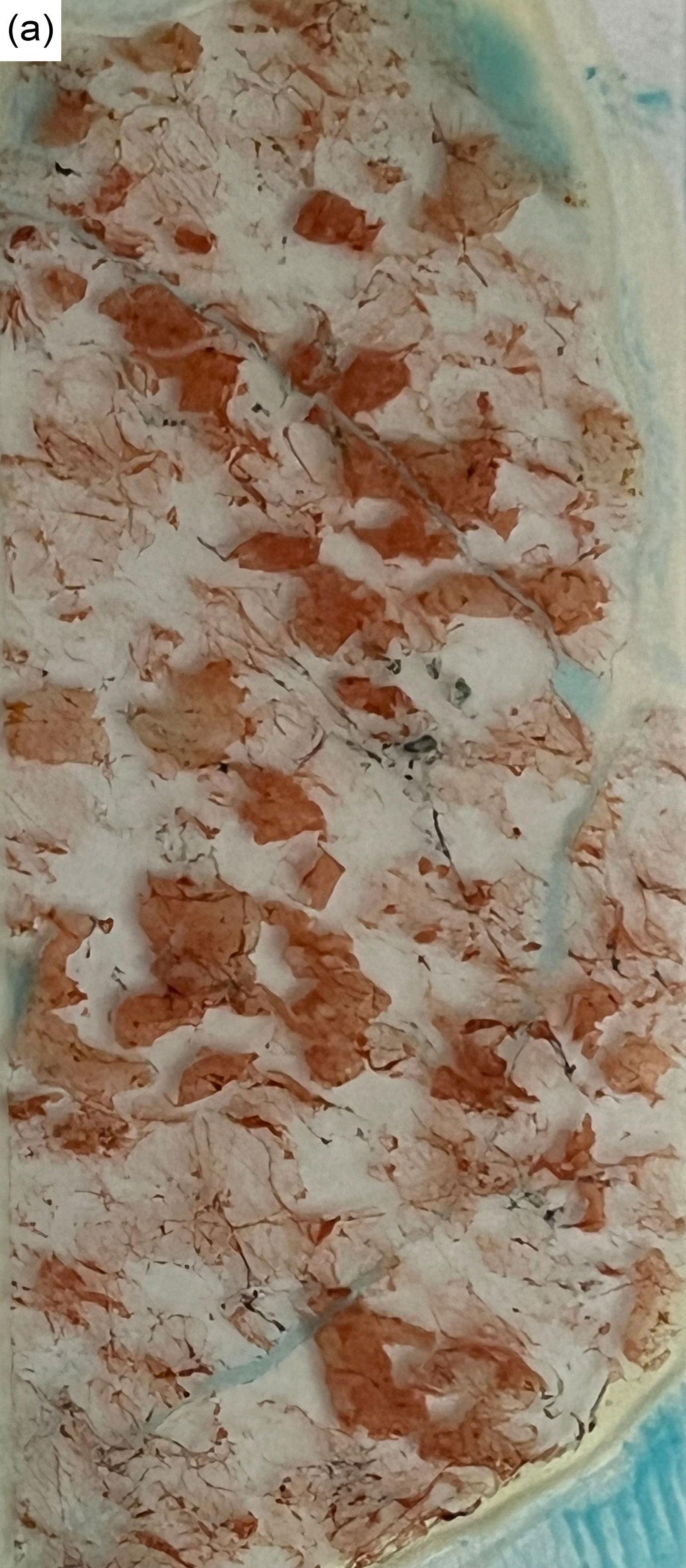
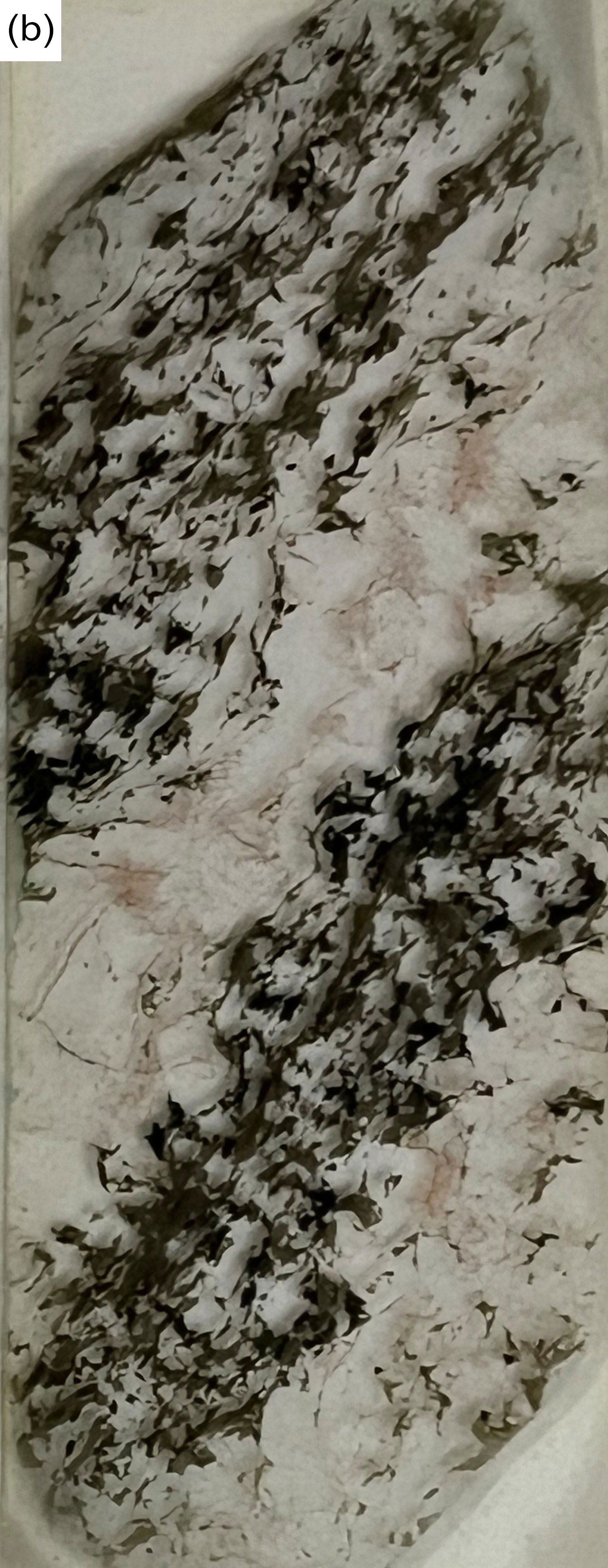
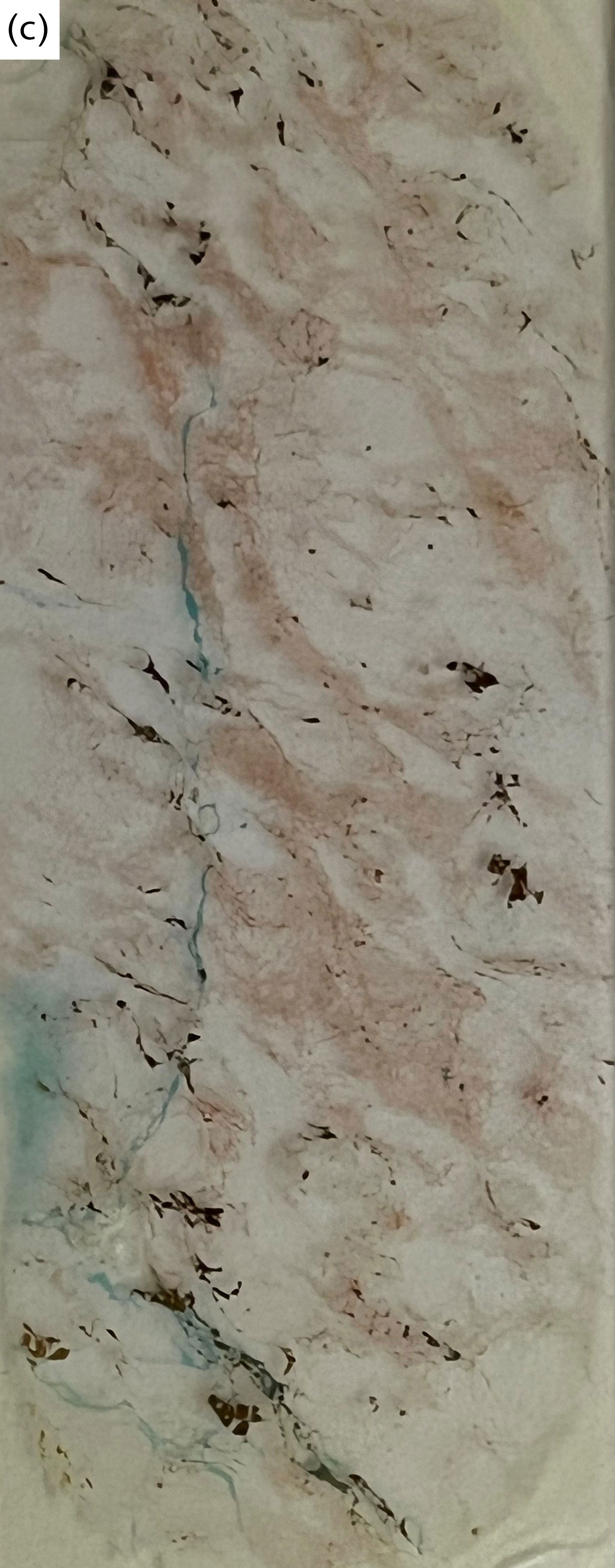
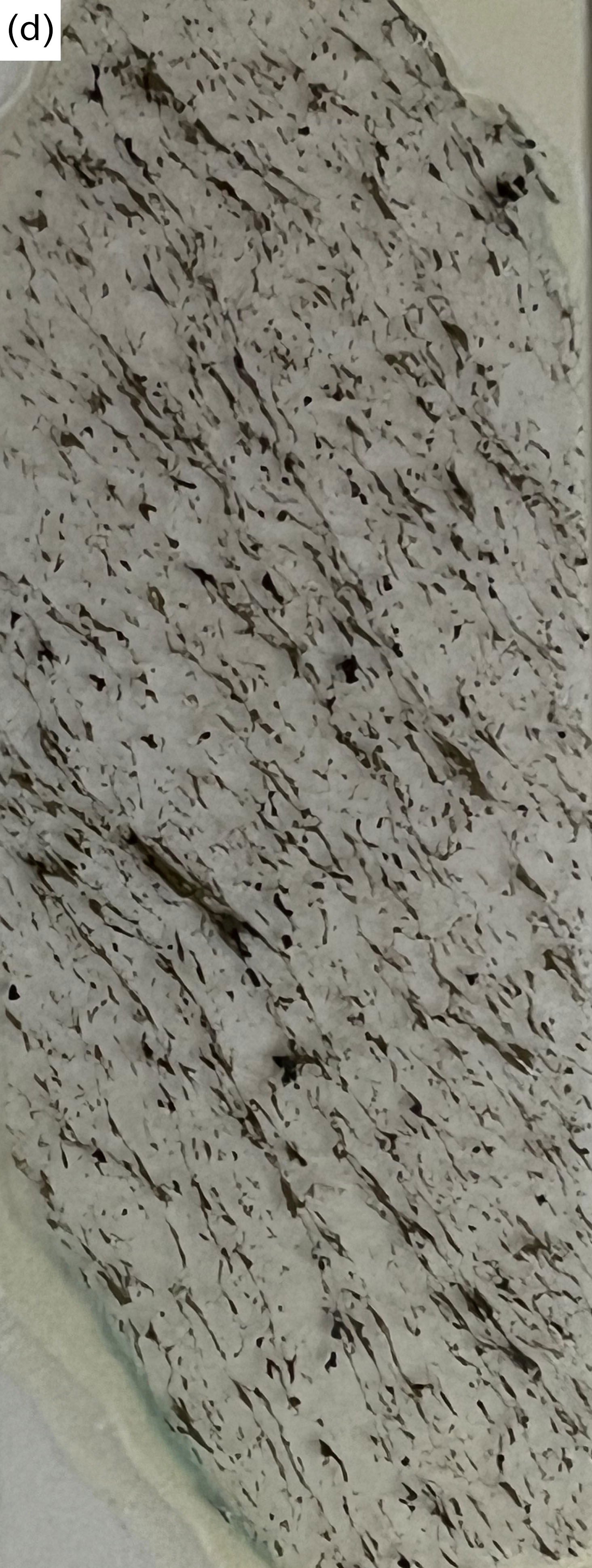
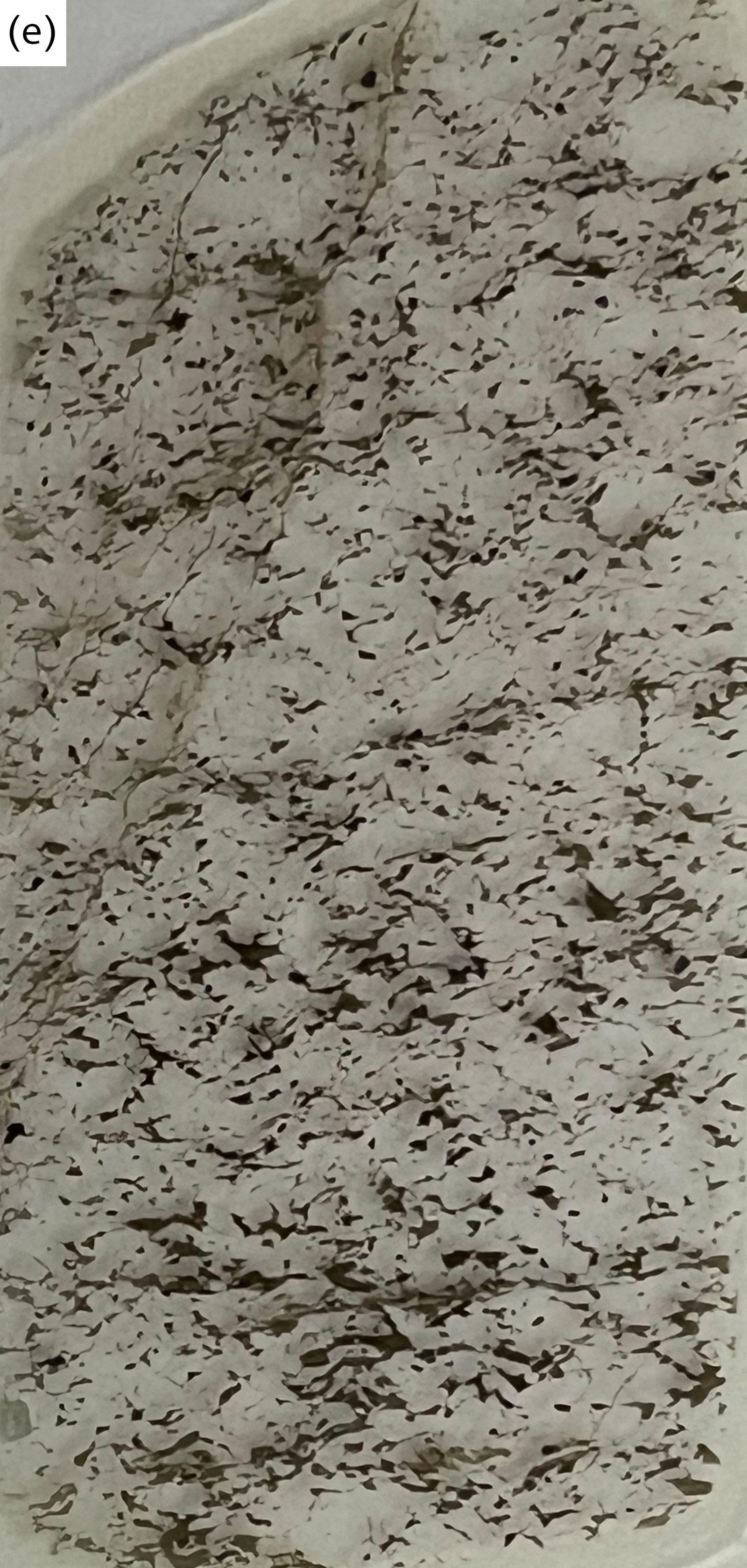
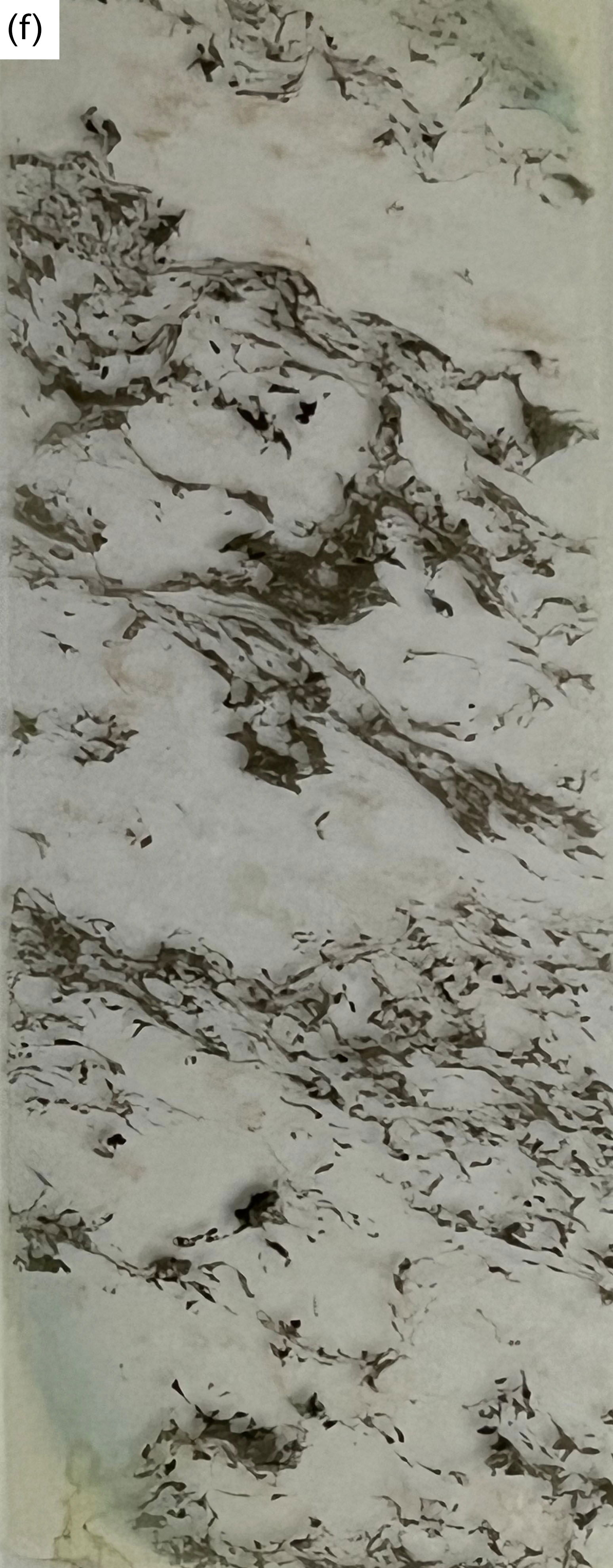
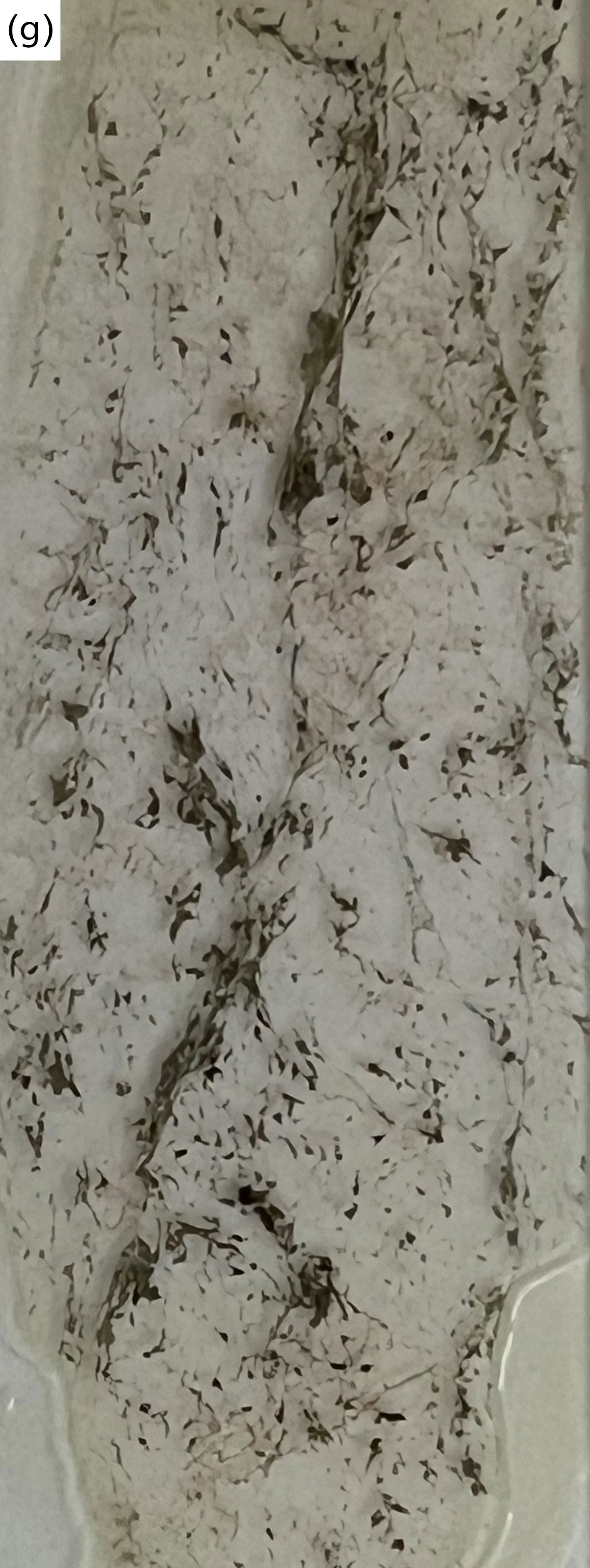
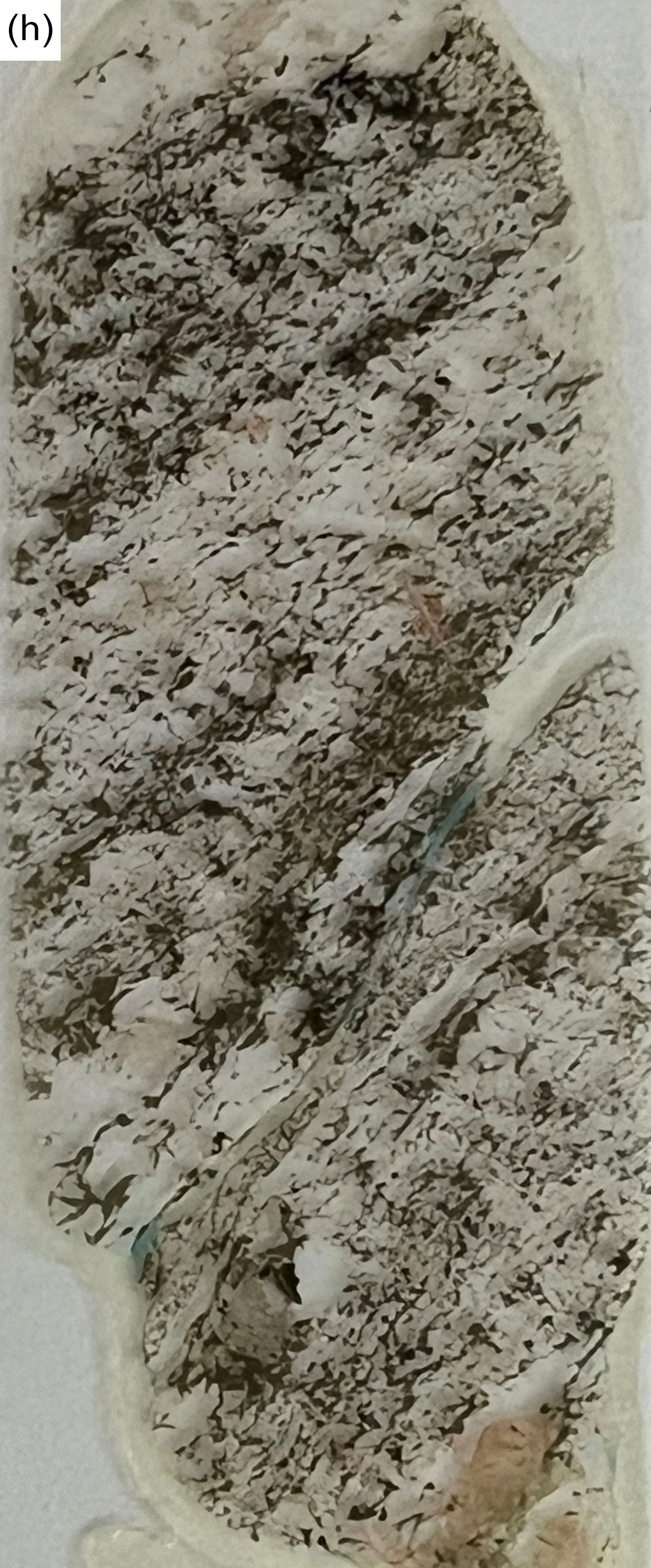
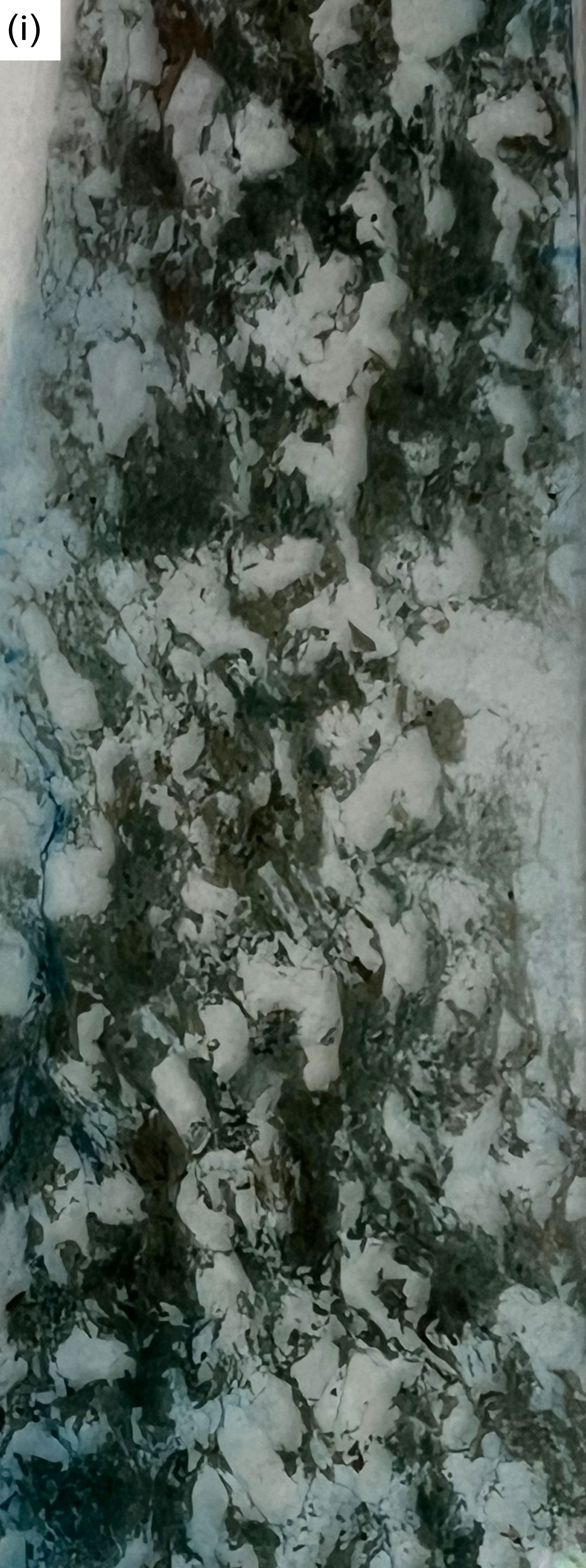
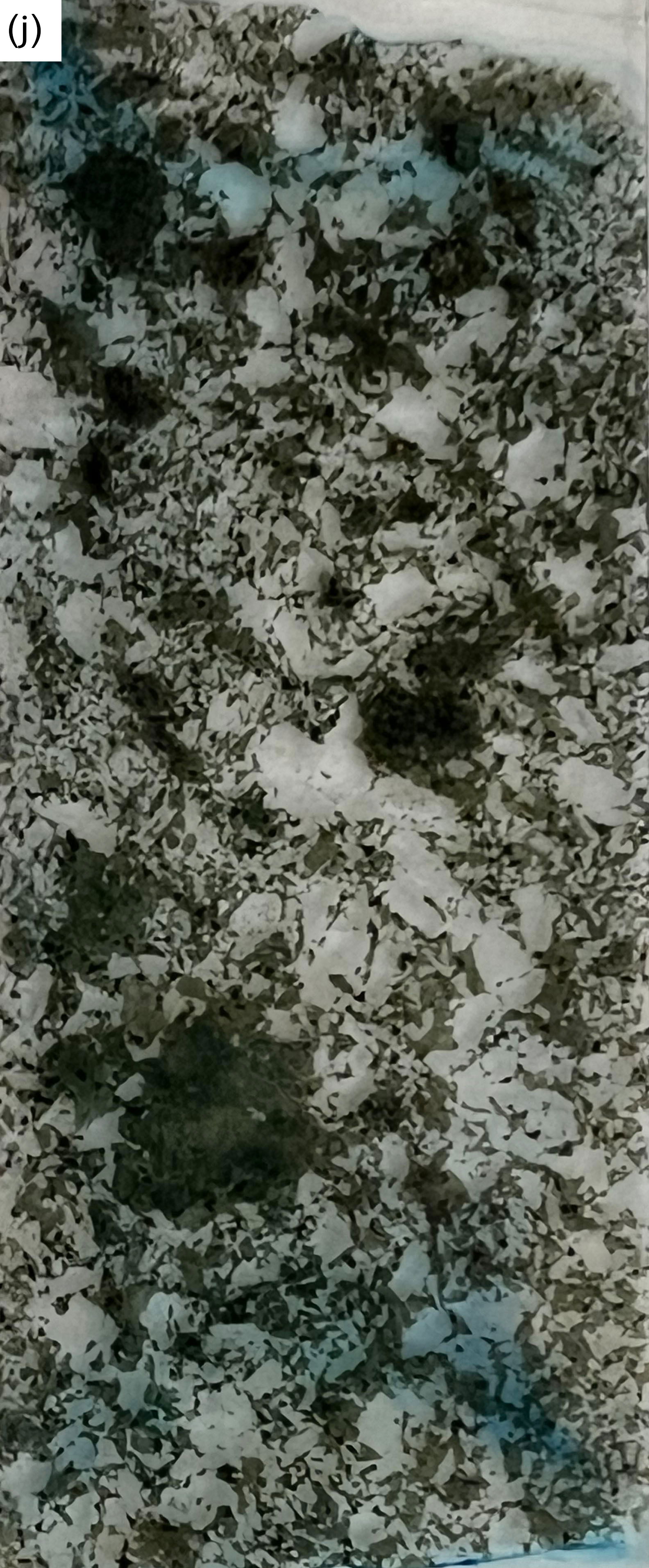
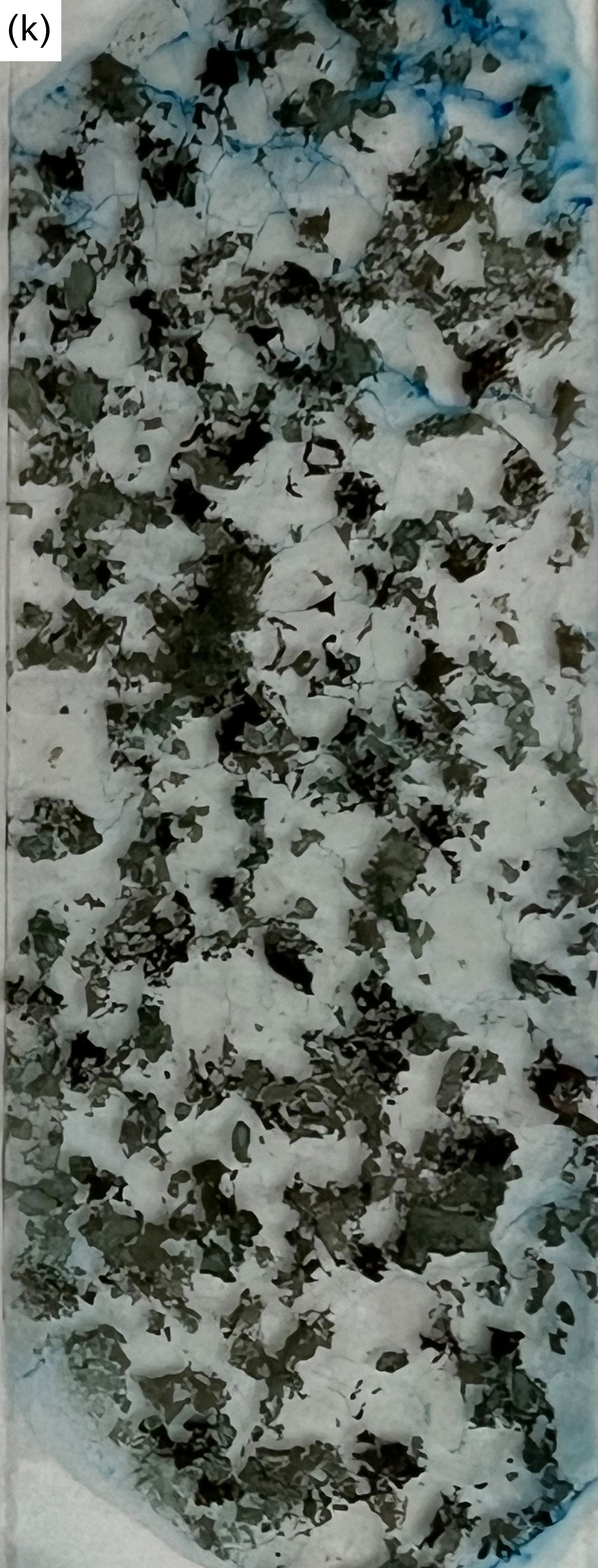
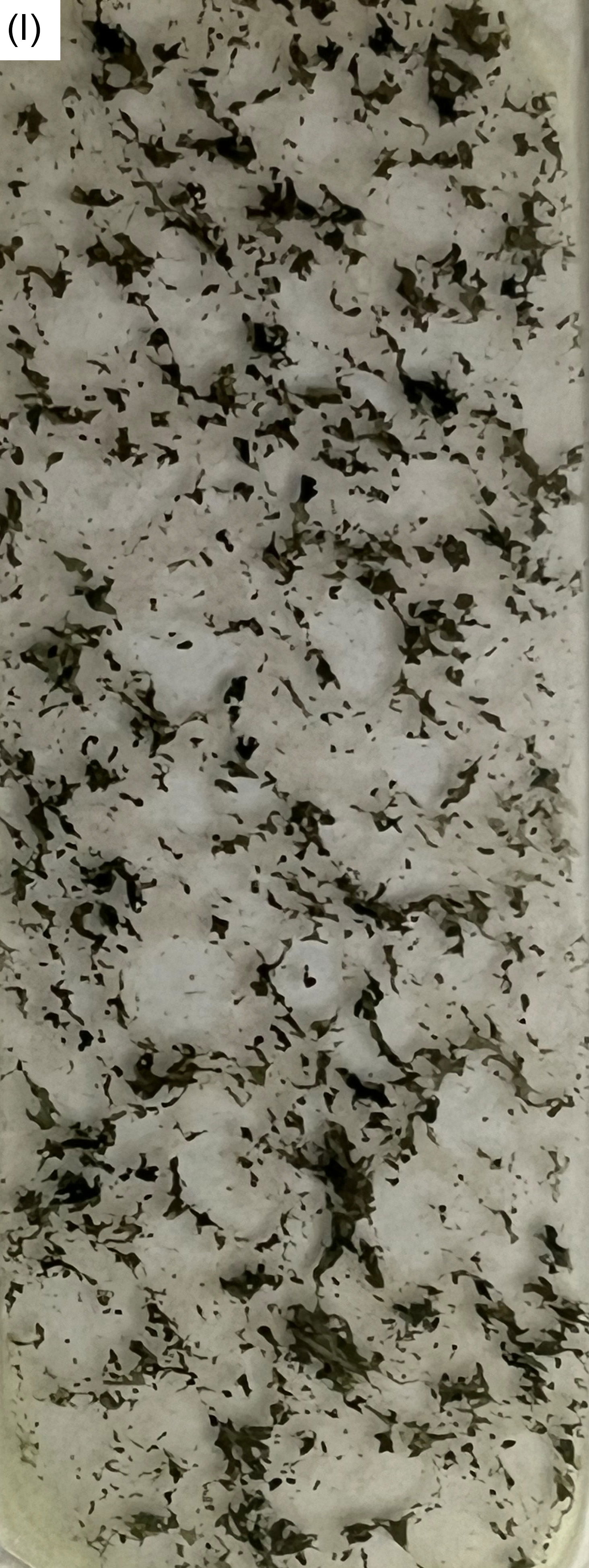
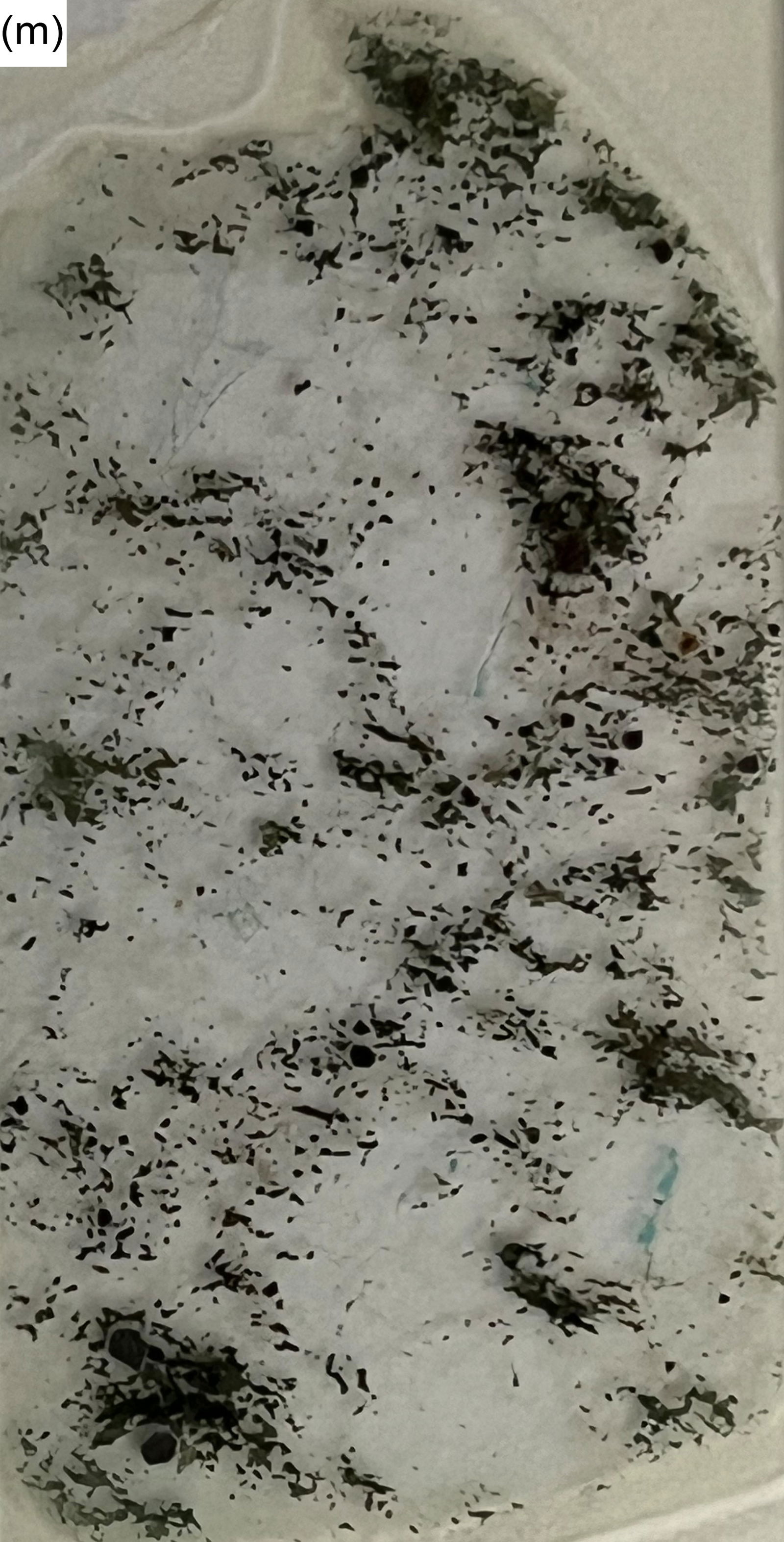
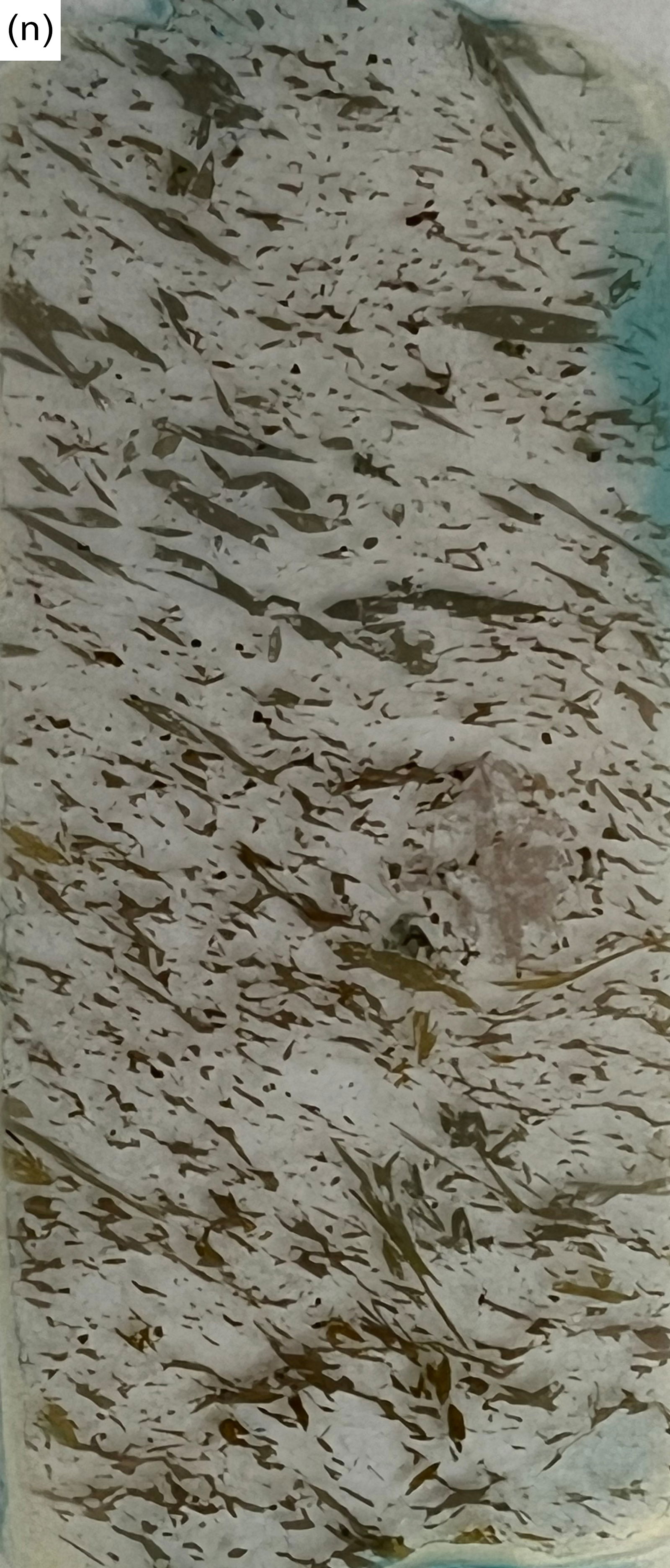
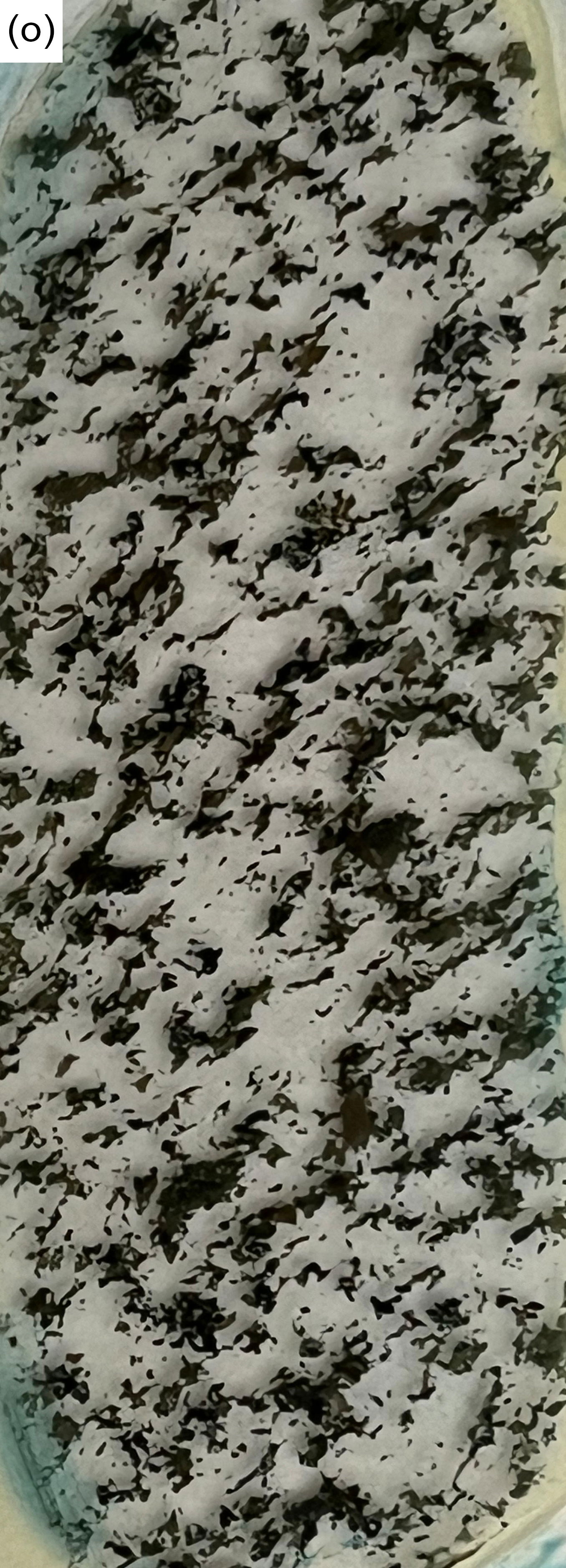
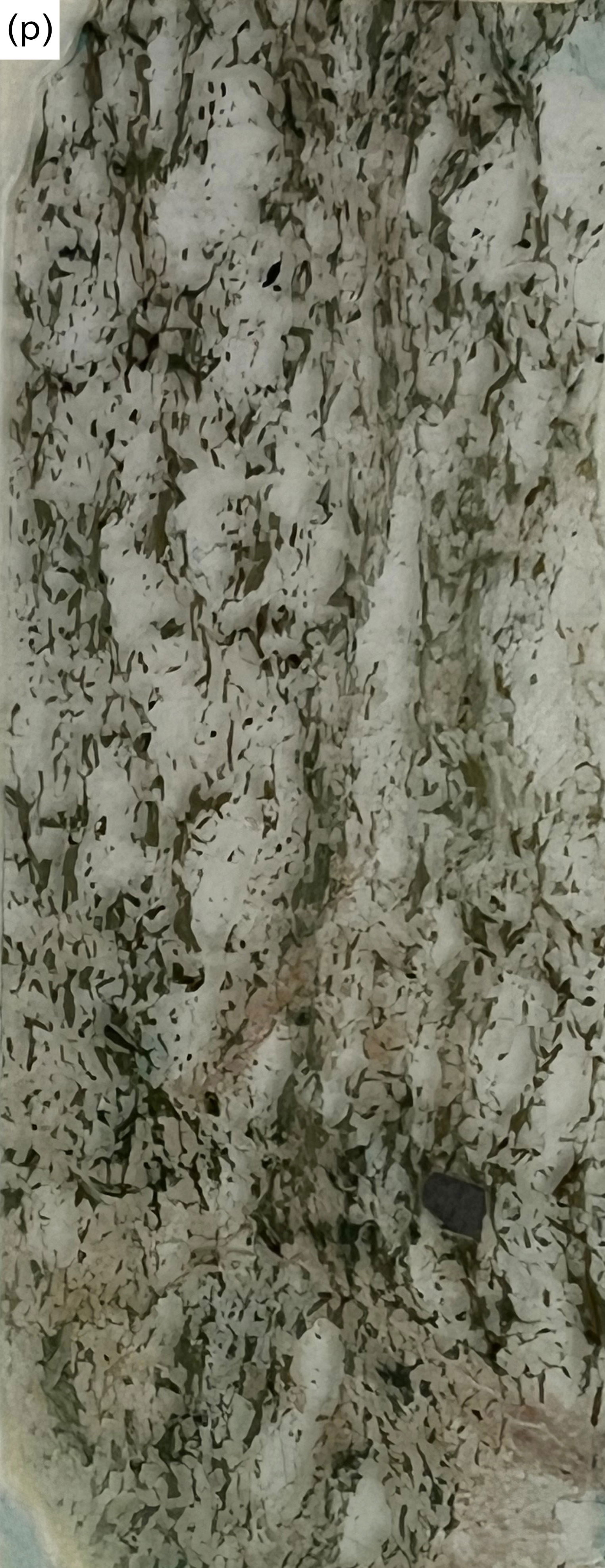
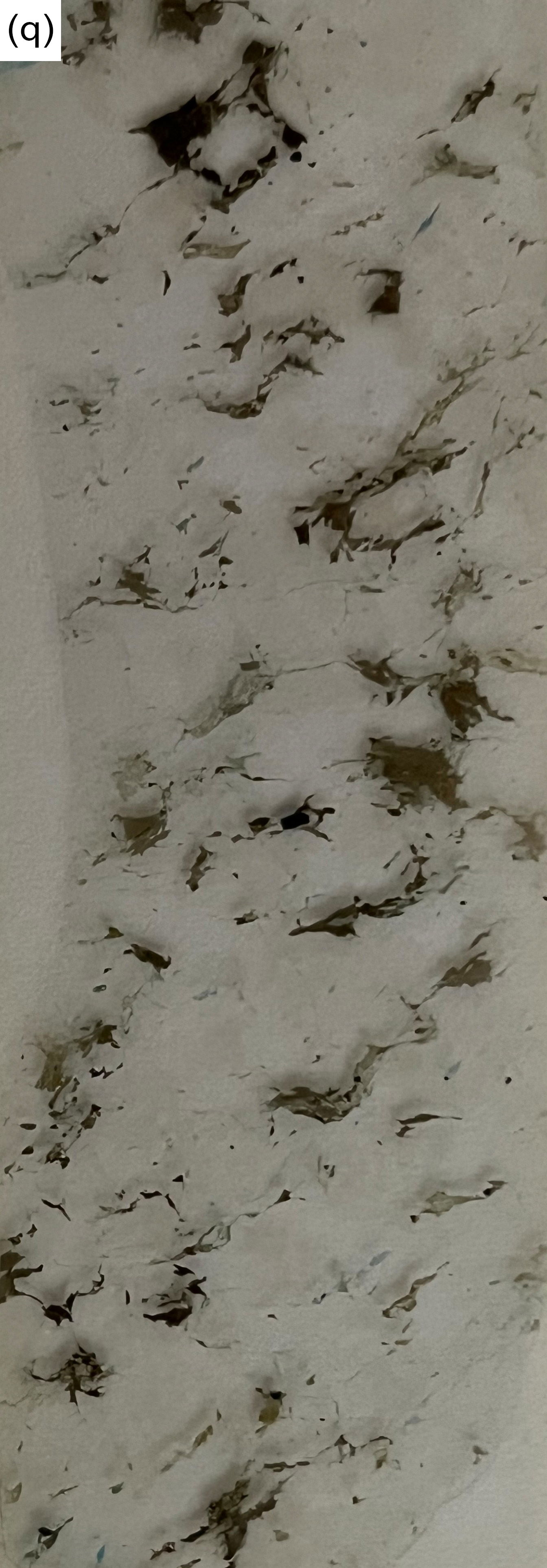
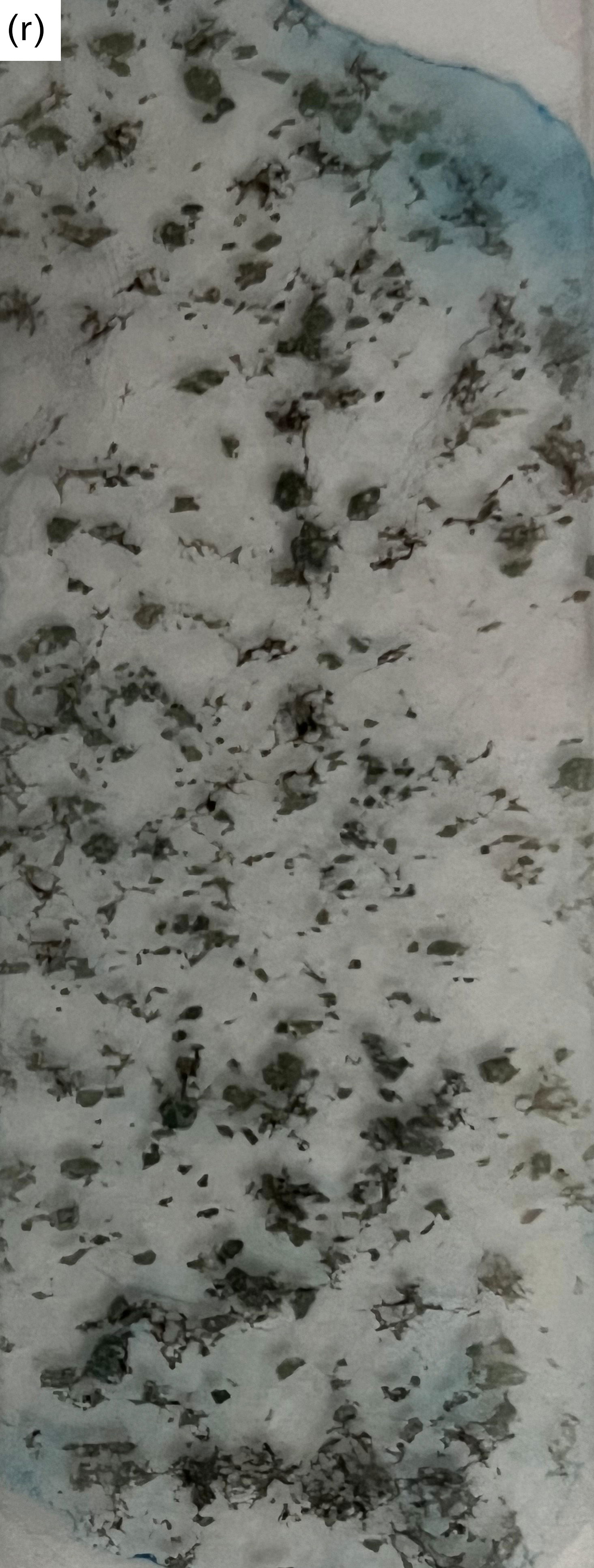
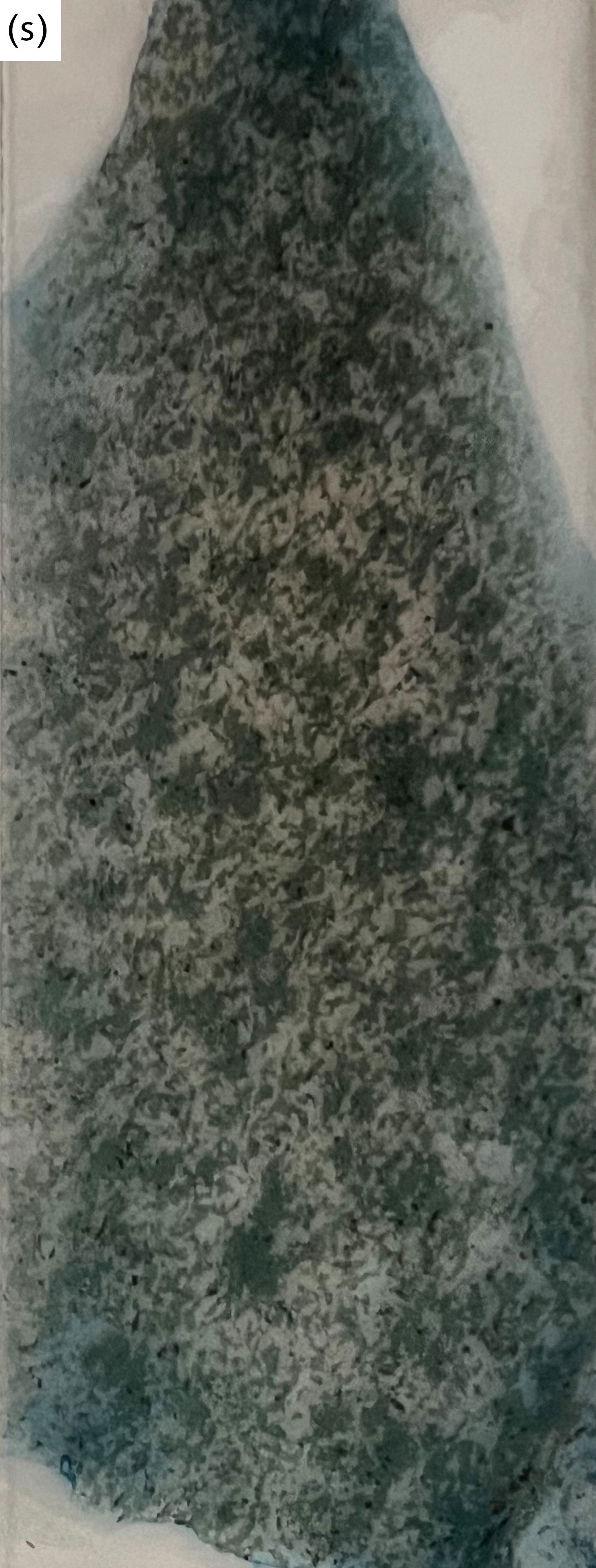
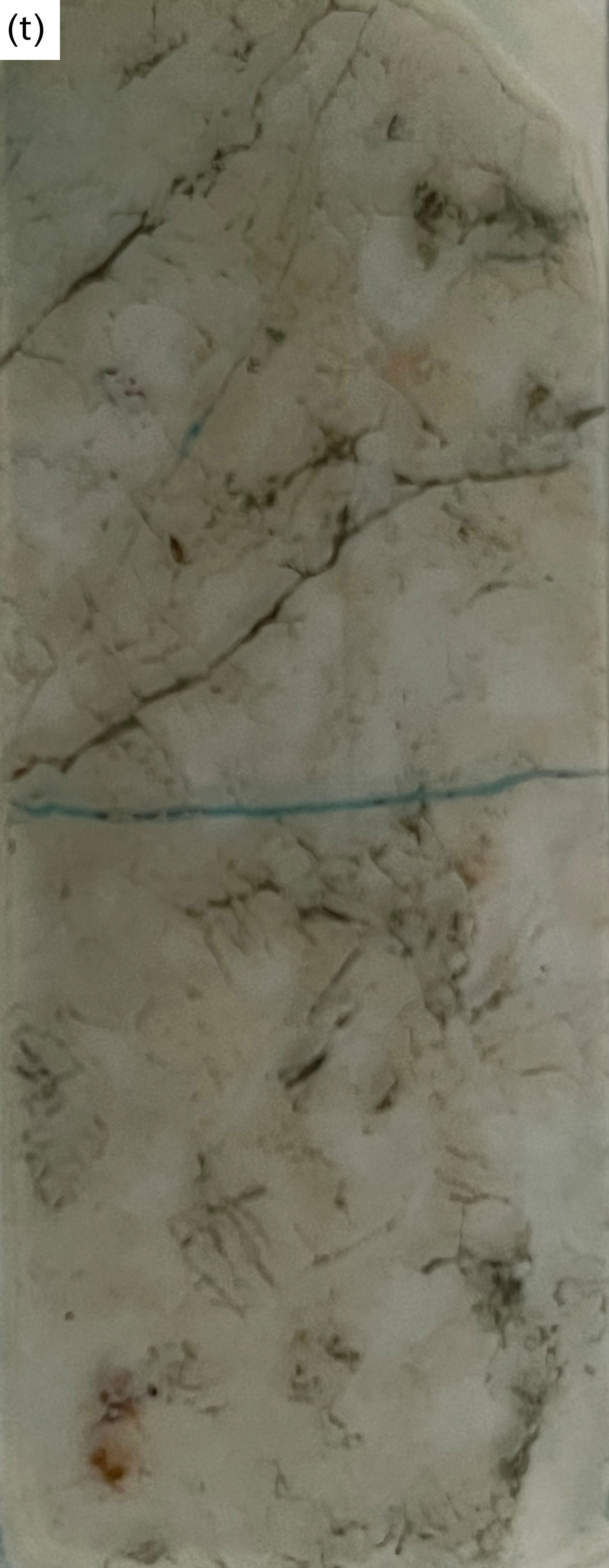
Fig. 8. Selected thin sections all at the same benchtop scale (~25 mm wide by ~65 mm long) for some of the granite plutons and pegmatite complexes, showing the variations in granitic compositions even within the same plutons: (a) Cottonwood Pegmatite complex, sample ZG-2; (b) Pipe Creek pluton, sample ZG-4; (c) Horn Creek pluton, sample ZG-1; (d) Trinity pluton, sample TG-1; (e) Trinity pluton, sample TG-2; (f) Trinity pluton, sample TG-3; (g) Trinity pluton, sample TG-5; (h) Trinity pluton, sample TG-6; (i) Ruby pluton, sample RP-1; (j) Ruby pluton, sample RP-2; (k) Ruby pluton, sample RP-3; (l) Elves Chasm pluton, sample ECG-1; (m) Elves Chasm pluton, sample ECG-2; (n) Elves Chasm pluton, sample ECG-4A; (o) Elves Chasm pluton, sample ECG-7; (p) Elves Chasm pluton, sample ECG-10; (q) Diamond Creek pluton, sample LGG-1; (r) Diamond Creek pluton, sample LGG-2; (s) Diamond Creek pluton, sample LGG-3; and (t) Diamond Creek pluton, sample ZG-3.
For example, the granite pegmatite from the Cottonwood Complex in fig. 8a is distinctive with its large pink K-feldspar crystals and accompanying large quartz crystals. In contrast, the gneissic granite of the Pipe Creek pluton in fig. 8b is foliated and banded with its layers of biotite (black) flakes and comparatively smaller quartz crystals alternating with layers of larger quartz crystals and minor pink K-feldspar and occasional plagioclase crystals.
Similarly, in the sample from the Horn Creek pluton (fig. 8c) pink K-feldspar crystals can be seen interspersed between clear quartz and occasional plagioclase crystals with only scattered black biotite flakes, whereas the six samples from the Trinity pluton (fig. 8d–h) are devoid of pink K-feldspar crystals and are instead often foliated with bands of aligned black biotite flakes alternating with bands of clear quartz and plagioclase crystals. But even then, though their mineralogy is consistent there are variations in the textures in those Trinity pluton samples with some less foliated and some with fewer biotite flakes. In contrast, the three samples from the Ruby pluton (fig. 8i–k) are more uniform in both mineralogy and texture, consisting of black hornblende grains and biotite flakes interspersed between them with clear quartz and plagioclase crystals in a generally non-foliated texture.
The five samples from the Elves Chasm pluton (fig. 8l–p) are also very similar in their mineralogy but their textures vary. Again, black biotite flakes accompanied by minor black hornblende grains are interspersed between clear quartz and grayish plagioclase crystals. The biotite flakes and hornblende grains are generally small, but the quartz crystals particularly vary in size from small to large, which affects the resulting textures. For example, compare fig. 8m and 8o. Some of these samples also display weak foliation (fig. 8n–p). The four samples from the Diamond Creek pluton (fig. 8q–t) are consistent in their mineralogy (small black biotite flakes and hornblende grains interspersed between larger clear quartz and grayish plagioclase crystals) but display textural variability, similar to the five Elves Chasm pluton samples (fig. 8l–p). However, again the quantities of the minerals vary widely, such as much more plagioclase and hardly any biotite in fig. 8q compared with the predominance of biotite and some hornblende in fig. 8s.
Fig. 9 shows what samples from several granite plutons look like under the petrographic microscope. In plane polarized light (fig. 9a–e) the biotite flakes that host the radiohalos are brown, the various shades or intensities of brown being due to their orientation with respect to the polarizing filter and microscope stage angle. Note also that often the biotite flakes are elongated and aligned which has resulted in the overall foliated textures of those samples. In fig. 9d the large greenish grains are hornblende, which primarily occurs clumped with the biotite flakes in those samples in which it is found. Otherwise, the white clear grains are quartz, while the “dirty”-looking white grains are plagioclase, the “dirtiness” being due to the minor alteration to which plagioclase is susceptible. Plagioclase is more abundant in the Ruby pluton sample (fig. 9d) in which the hornblende occurs, so it is a tonalite compared to the other samples which are granodiorite. Fig. 9f is the same view as fig. 9e but under crossed polarized light in which the quartz grains display various shades of yellow and gray depending on the crystal lattice orientations of the grains and their orientations on the microscope stage.
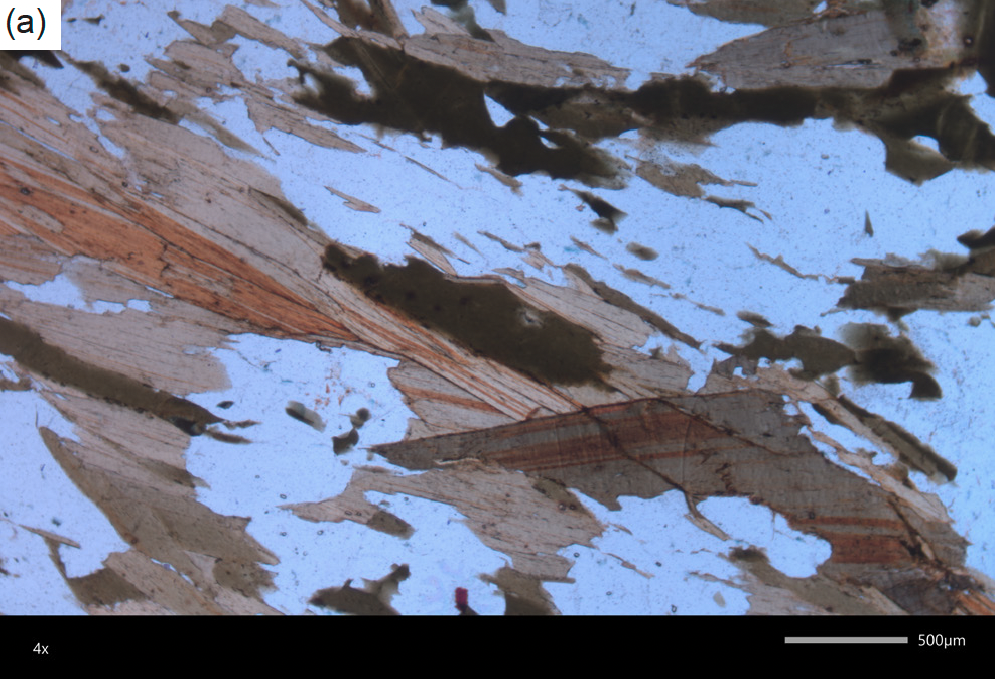
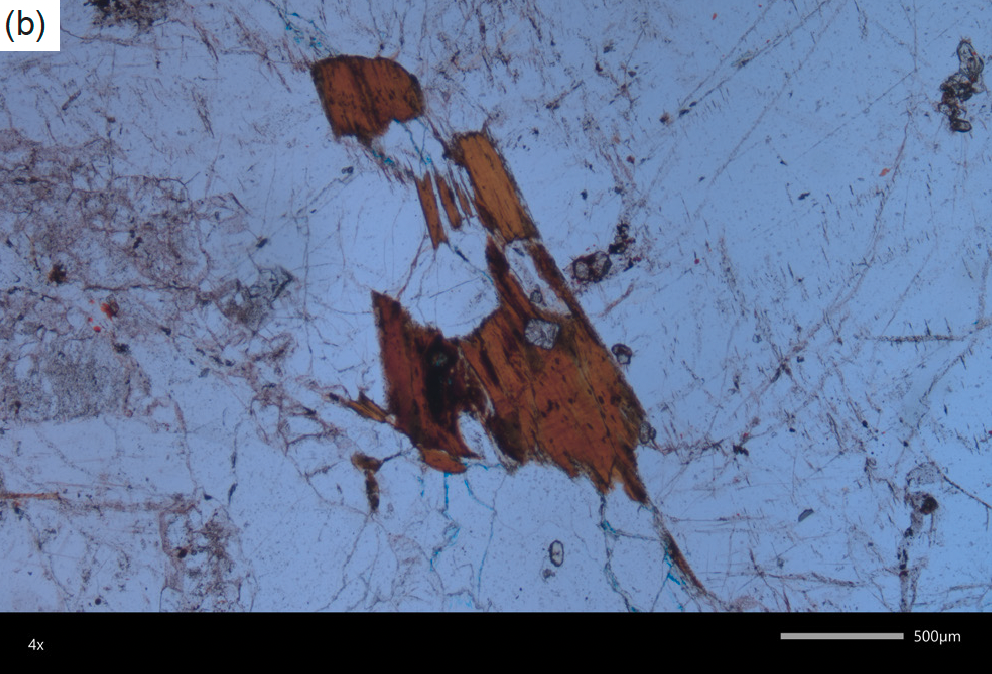
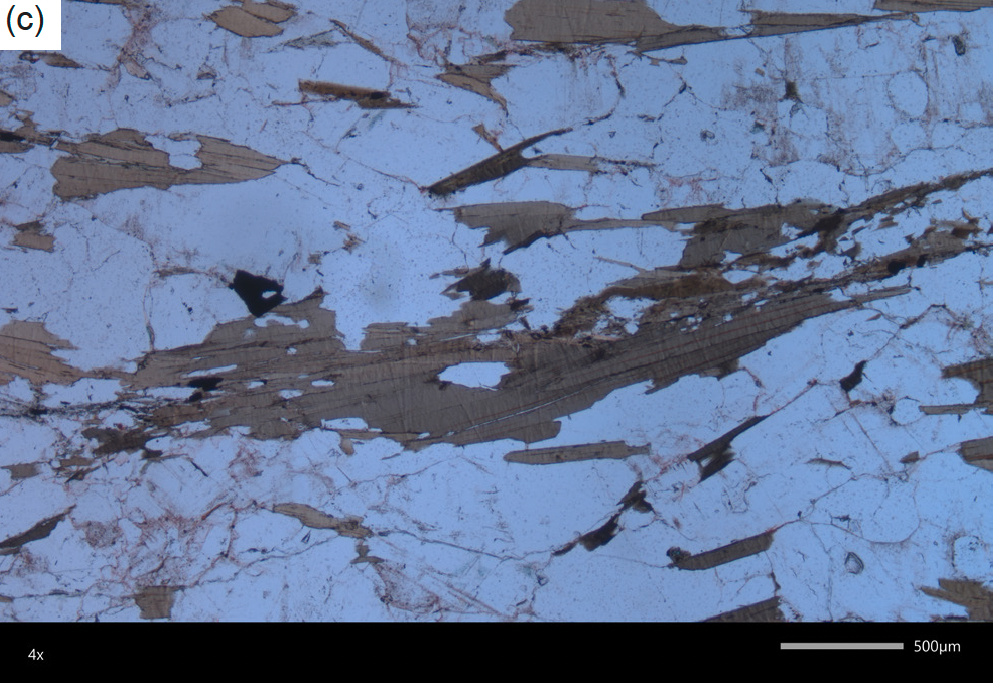
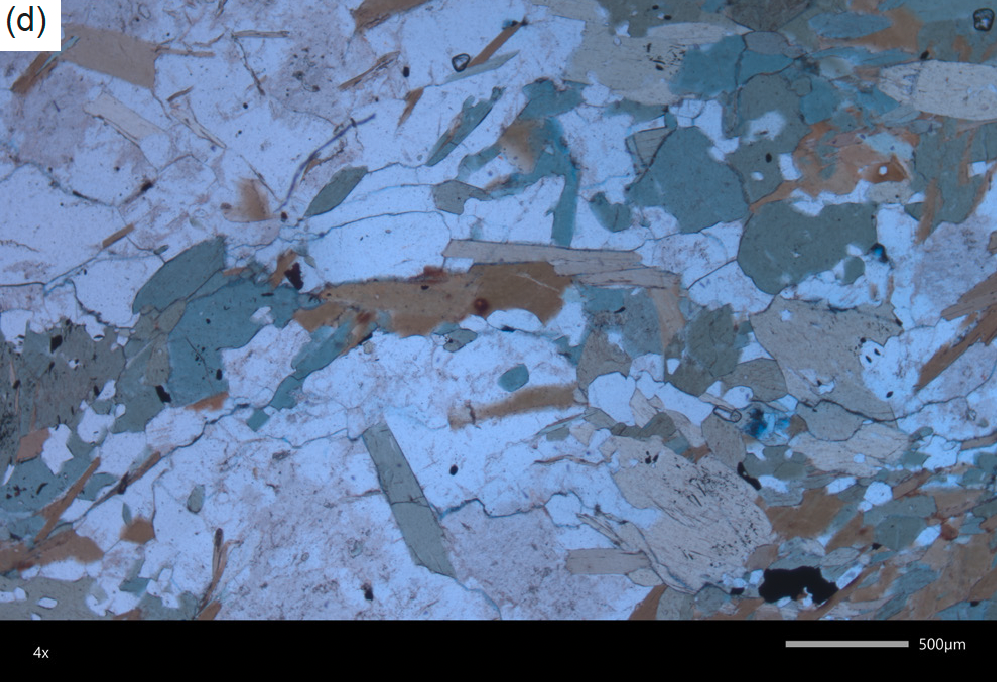
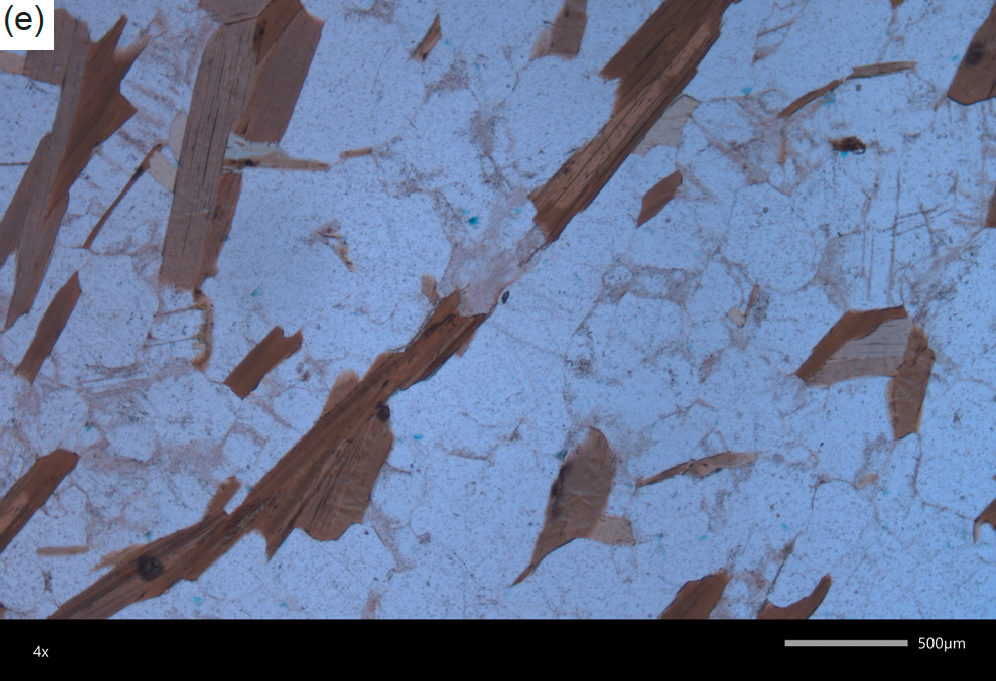
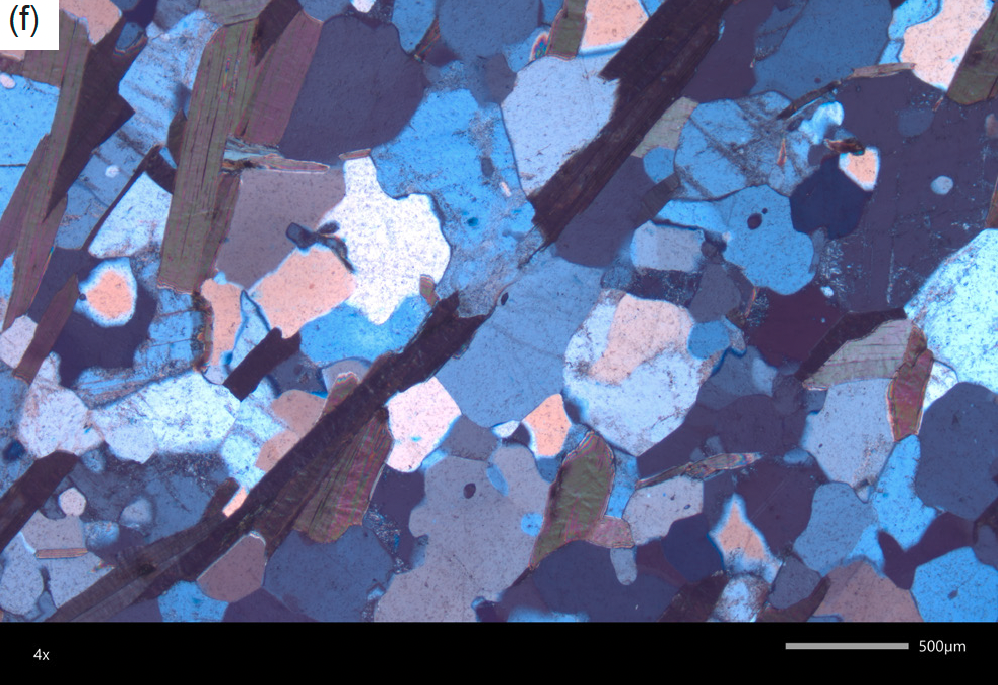
Fig. 9. Some typical samples of the granites viewed under the petrographic microscope in plane polarized light (a–e) and under crossed polars (f). The scale bars = 500 μm. (a) Pipe Creek pluton, sample ZG-4, river mile 88.8. (b) Horn Creek pluton, sample ZG-1, river mile 90.2. (c) Trinity pluton, sample TG-1, river mile 91.2.(d) Ruby pluton, sample RP-1, river mile 102. (e), (f) Elves Chasm pluton, sample ECG-4, river mile 112.5.
Table 2 lists the results of all the radiohalos counted in each of the samples, while table 3 summarizes the total radiohalos numbers in each of the sampled granite plutons and pegmatite complexes. In both tables the numbers of each type of radiohalo observed and counted are listed, the numbers of all radiohalos and Po radiohalos per slide (the total numbers counted divided by the number of microscope slides), and the relevant ratios (primarily the 210Po/238U ratio). The numbers of all radiohalos and Po radiohalos per slide and the 210Po/238U ratios are extremely important for statistical comparisons between samples from the same rock unit and then also between rock units.
Table 3. Summary of the radiohalos counted in all samples of each of the Precambrian granite plutons and pegmatite complexes in the Upper, Middle and Lower Granite Gorges.
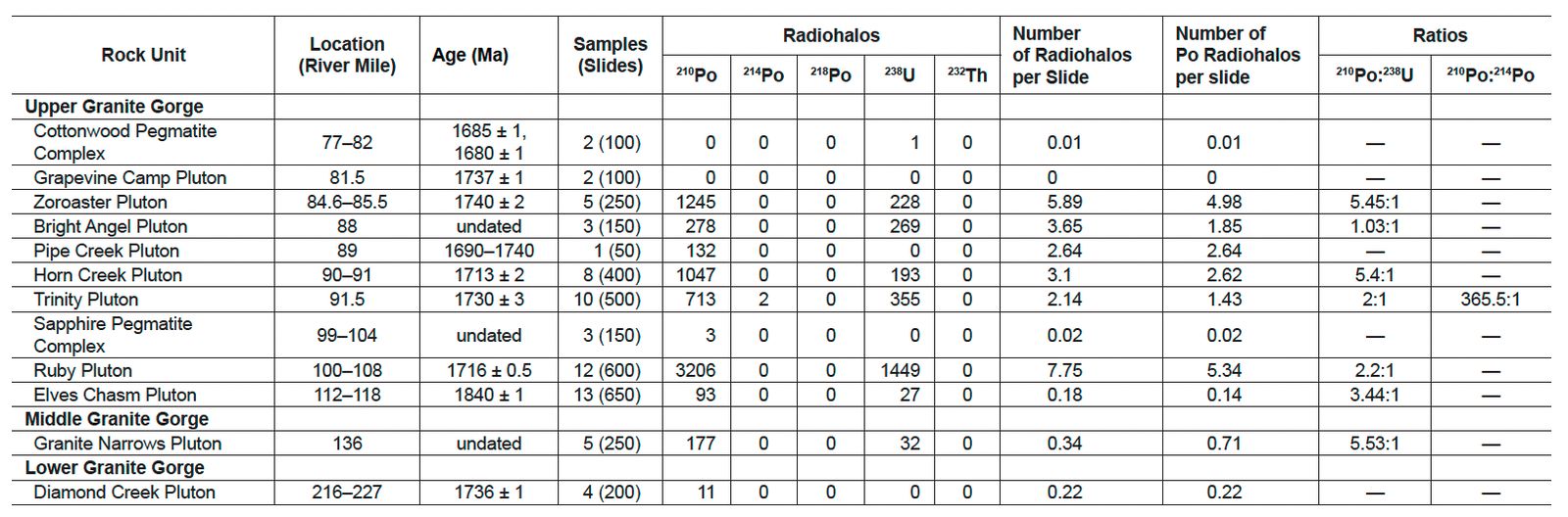
Fig. 10 provides photomicrographs of a few examples of the radiohalos observed in samples from some of the granite plutons. While these are mostly 210Po radiohalos, in some biotite flakes there are large zircon inclusions around which are intense radiation stains due to the damage caused by the α-particles emitted by the high uranium contents of the zircons (fig. 10c–e). Note that there are no radiohalos or even zircon inclusions in the green hornblende grains in fig. 10f and g. Otherwise, the 210Po radiohalos in fig. 10a–c and f–h are all dark, indicating there had been high concentrations of only 210Po atoms in their central radiocenters, most of which are visible and appear to have been tiny bubbles that are now clear.
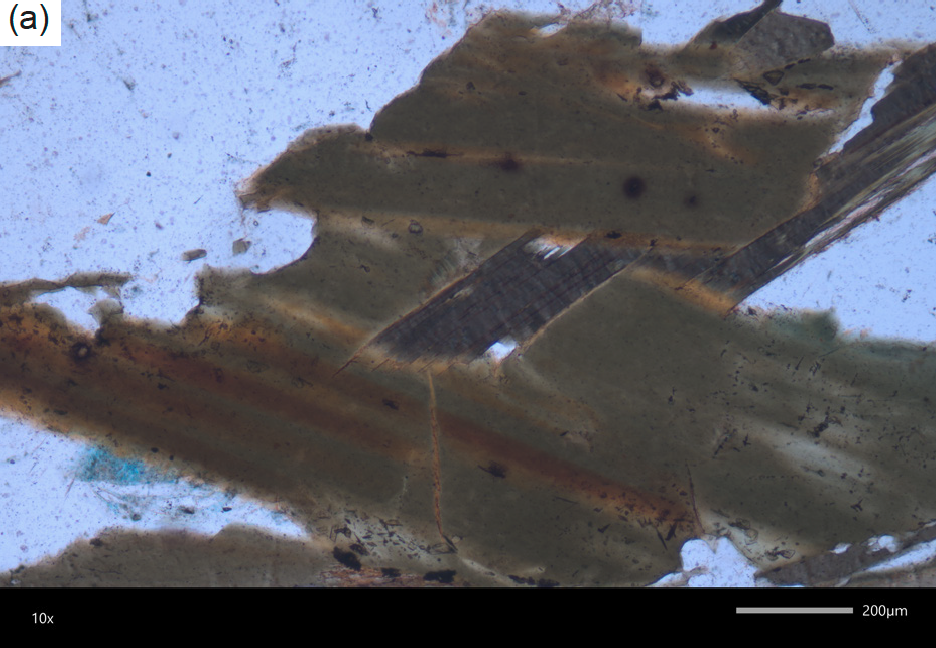
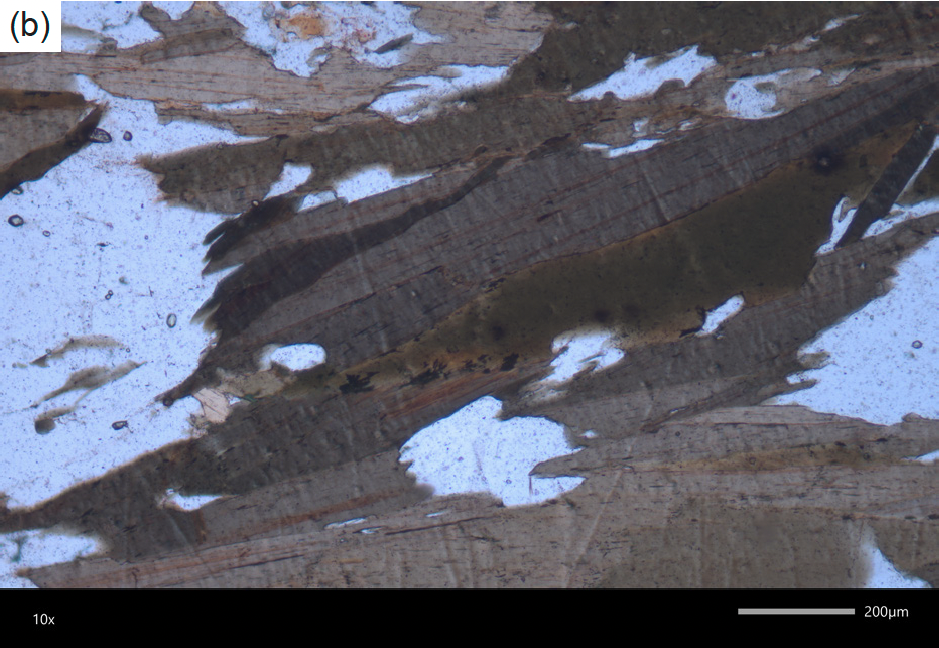
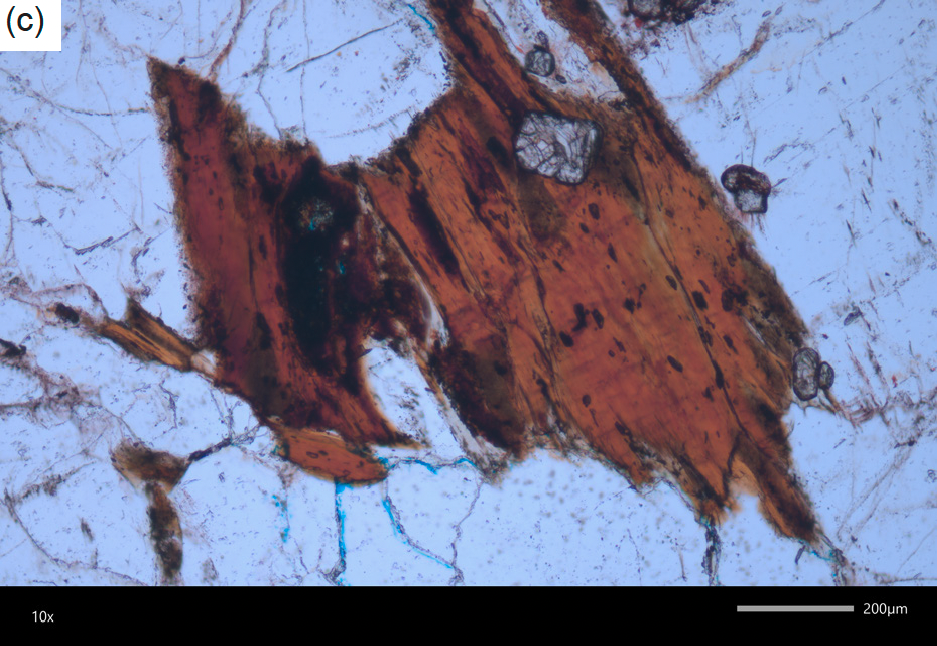
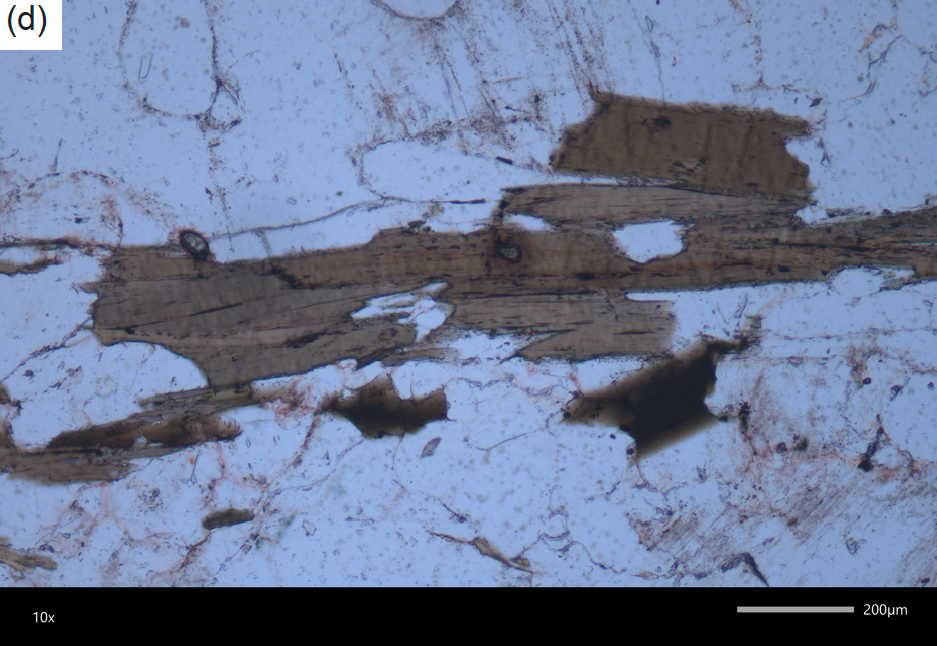
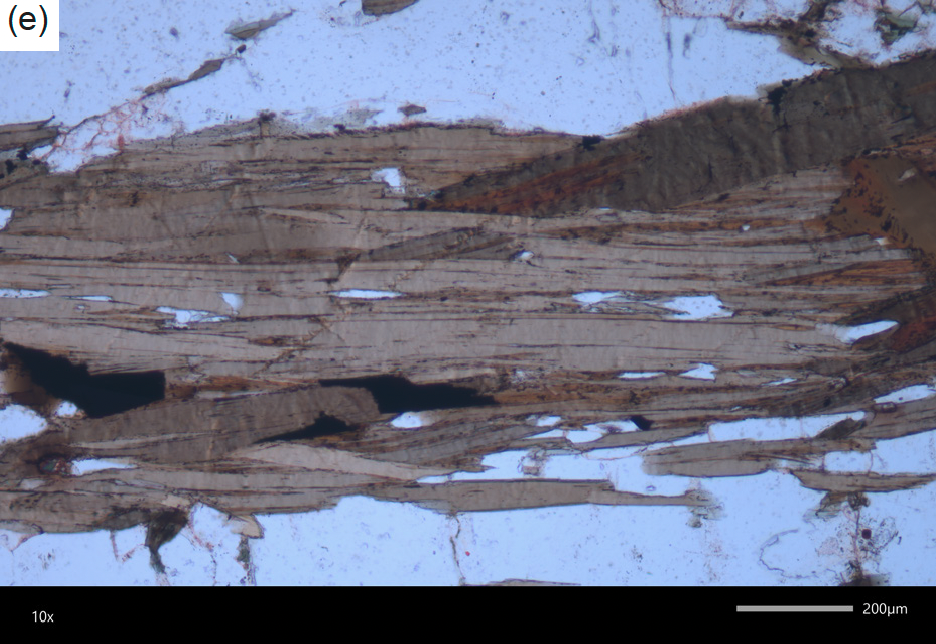
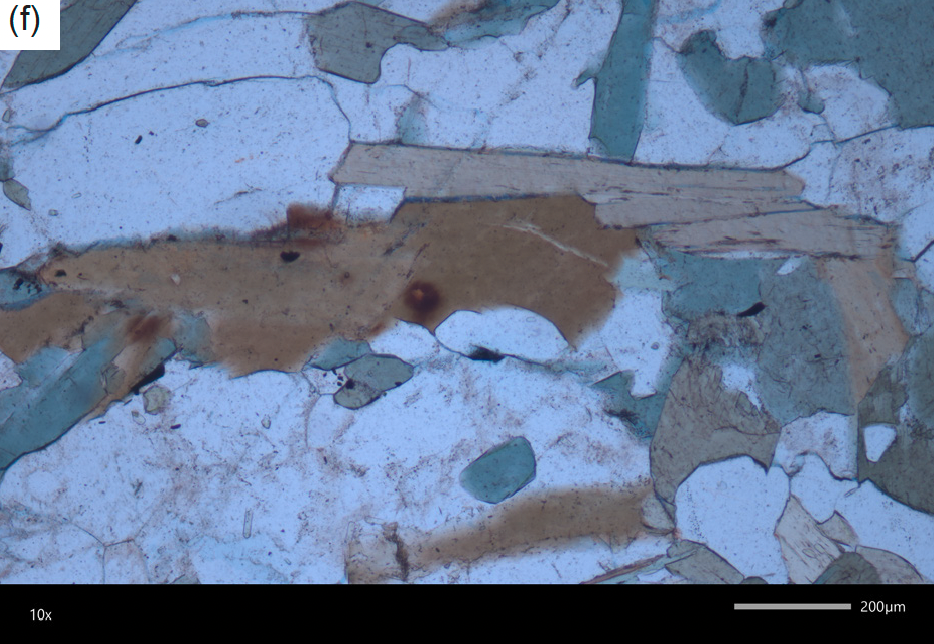
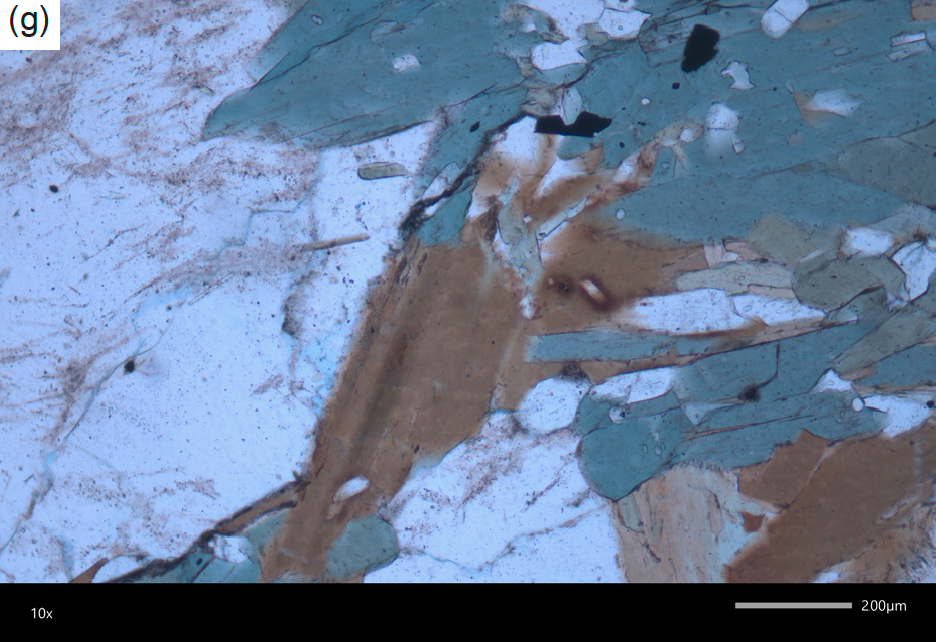
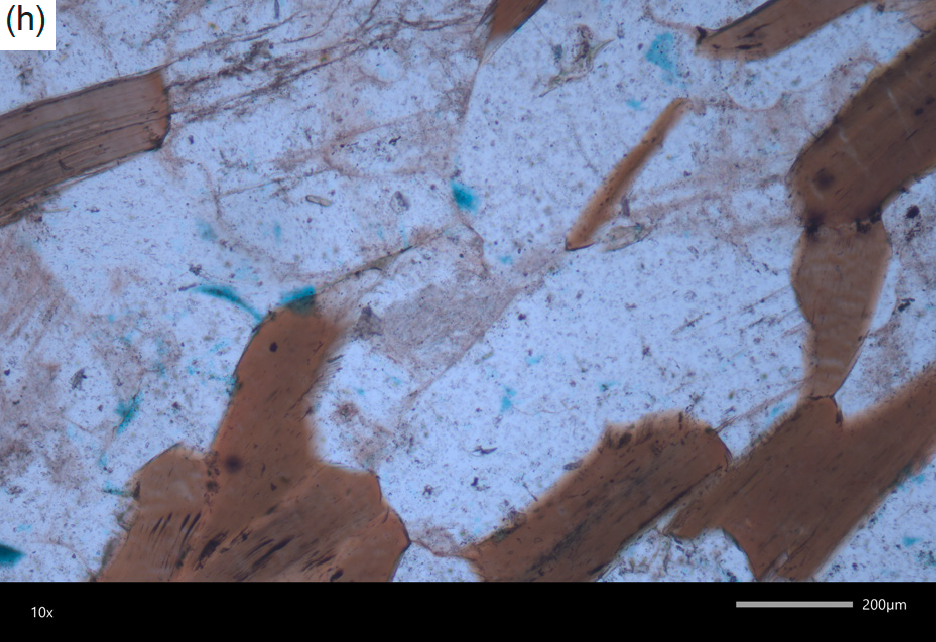
Fig. 10. Typical radiohalos in some samples of the granites. The scale bars = 200 μm. (a) Pipe Creek Pluton, sample ZG-4, river mile 88.8, a dark 210Po radiohalo (upper right). (b) Pipe Creek Pluton, sample ZG-4, river mile 88.8, another dark 210Po radiohalo (upper right). (c) Horn Creek Pluton, sample ZG-1, river mile 90.2, dark radiation damage around a large zircon crystal (center left) and an adjacent dark 210Po radiohalo (center). (d) Trinity Pluton, sample TG-1, river mile 91.2, light radiation damage around a large zircon crystal (center). (e) Trinity Pluton, sample TG-1, river mile 91.2, darker radiation damage around another large zircon crystal (far left). (f) Ruby Pluton, sample RP-1, river mile, a dark 210Po radiohalo (center). (g) Ruby Pluton, sample RP-1, river mile , another dark 210Po radiohalo (center). (h) Elves Chasm Pluton, sample ECG-4, river mile 112.5, two dark 210Po radiohalos (bottom left and top right).
Discussion
Are These Flood Granites?
Some creation geologists argue these granites in the Precambrian crystalline basement of Grand Canyon are not Creation Week rocks created supernaturally, as argued by many other creation geologists (Austin 1994; Snelling 2009, 2022b), but instead were generated and intruded into the schists during the Flood. However, a number of critical observations do not support that contention. So, before proceeding to interpret the radiohalos occurring in these granites it is necessary to settle exactly when these granites were formed, here argued as being created by God during Creation Days 1–2.
First, these Precambrian (Paleoproterozoic) granites, along with the schists, were deformed then eroded before the Precambrian Grand Canyon Supergroup sediment layers were deposited uncomformably on them, starting with the ~1254–1100 Ma Unkar Group (Timmons et al. 2005, 2012). The best exposure of this unconformity is at river level from miles 74 to 78 downstream from Lees Ferry. At the base of the Bass Formation, the lowermost and first-deposited unit, is the Hotauta Conglomerate Member which consists of >80% clasts of granite and quartzite (Timmons et al. 2012). Furthermore, detrital zircon grains recovered from several Unkar Group sedimentary layers include grains eroded from nearby Grand Canyon granites, as indicated by the geochemical “fingerprint” of their U-Pb “ages” (Timmons et al. 2005, 2012). So, even if some creation geologists interpret the Unkar Group sedimentary layers as deposited at the outset of the Flood, these underlying basement granites had already been intruded into the basement schists.
Second, these Precambrian (Paleoproterozoic) granites, along with the schists and the Precambrian (Mesoproterozoic and Neoproterozoic) Grand Canyon Supergroup sediment layers were deformed then eroded before the Phanerozoic sedimentary layers were uncomformably deposited on them (Karlstrom and Timmons 2012). There was a major tectonic upheaval before this massive erosion occurred to produce the Great Unconformity in which faulting with huge displacements of thousands of meters downthrust blocks of Grand Canyon Supergroup rocks so that they were preserved in place when the subsequent massive erosion completely beveled the crystalline basement and the surviving blocks of Grand Canyon Supergroup rocks (Karlstrom and Timmons 2012). Thus, in many places through the inner gorges of Grand Canyon the 3600-meter-thick Grand Canyon Supergroup (Timmons et al. 2012) has been eroded away at the Great Unconformity, as well as an unknown thickness of the underlying granites and schists. Many creation geologists regard the Great Unconformity as marking the onset of the Flood (Austin 1994; Austin and Wise 1994; Snelling 2009, 2022b; Wise and Snelling 2005), not least because it is almost a global erosion surface (Peters and Gaines 2012). That the Great Unconformity sits directly on the Precambrian crystalline basement’s granite plutons can be seen in many places above the river corridor within the inner gorges of Grand Canyon. Furthermore, the first of the Phanerozoic sediment layers deposited on the Great Unconformity by the floodwaters was the Tapeats Sandstone and it contains many K-feldspar and zircon grains, and some muscovite flakes, that are demonstrably derived from erosion of the nearby underlying granites (Snelling 2021a). Indeed, the overlying Bright Angel and Muav Formations also contain similar grains and flakes that are demonstrably derived from erosion of the nearby underlying granites (Snelling 2021b, 2022a).
And finally, there are the interrelationships between these granite plutons and the schists. It is well-recognized and now established that the 1.84 Ga Elves Chasm pluton (Hawkins et al. 1996) is likely the basement for the turbidites that are now the Vishnu Schist (Karlstrom et al. 2003). The contact zone between the Elves Chasm pluton and the overlying Granite Gorge Metamorphic Suite is exposed in several places and is gradational over several meters. The composition of the contact zone is unusual, which suggested to Babcock (1990) that alteration took place during weathering before the overlying sediments were deposited that are now the Vishnu Schist. Furthermore, the regional metamorphism that formed the schists was accompanied by deformation and magmatism when the granite plutons were apparently intruded into the schists (Karlstrom et al. 2012). However, these intrusions generally did not bake and metamorphose the schists adjacent to them, although the pegmatite dike swarms intruded into some of the lithotectonic blocks (metamorphic domains separated by shear zones) appear to have created the hottest metamorphic rocks in the crystalline basement as determined by the mineral assemblages in the schists (Karlstrom et al. 2012). Thus, the whole package of schists and granites combined to form the crystalline basement was generated almost simultaneously, albeit slowly within the uniformitarian timescale.
Combining all these observations and evidences it is reasonably well-established that both the schists and the granites in Grand Canyon’s crystalline basement are pre-Flood rocks, the granites not being generated and intruded during the Flood. On the contrary, these granites are likely Creation Week rocks created by God on Creation Days 1–2 as contended here and by many creation geologists.
Were These Granites Created by Fiat?
A further contention that needs to be settled before proceeding to interpret the radiohalos occurring in these granites is to discuss how these granites were created, as it has implications for when and how the radiohalos formed. Specifically, were these granites formed by time progressive processes as precursor sedimentary and volcanic rocks underwent metamorphism, melting, and plutonism before the creation of plant life partway through Creation Day 3, or were these schists and granites created by fiat, that is, in a single step as fully-formed rocks?
Humphreys (2005) found 1.5 billion years’ worth of radiogenic Pb and almost that much helium (α-particles in the form of retained radiogenic helium) in the Precambrian Fenton Hill granodiorite of New Mexico. While the year-long Flood event would account for about 600 million years’ worth of accelerated radioisotope decay, it could be contended that it still leaves 900 million years’ worth of accelerated nuclear decay which thus may have occurred before the creation of plant life on Creation Day 3 as the rocks of Creation Days 1–2 underwent metamorphism, melting, and plutonism. However, the 1,500 million years’ worth of decay products in the Fenton Hill granodiorite is based on assuming those decay products all came from accelerated radioisotope decay. Could not God have created by fiat the granites and schists with some Pb isotopes and helium already in them? After all, when He created the element Pb, why would He not create all the Pb isotopes? In other words, He would have created Pb isotopes that today we interpret as derived by radioisotope decay, when in fact no radioisotope decay had yet occurred. Would there not have been a starting isotopic composition of the first created rocks? And besides, the radiohalos in the Fenton Hill granodiorite only record physical evidence of 100 million years’ worth or so of radioisotope decay as measured at today’s decay rate.
Space precludes detailed discussion and development of this issue of the initial composition of Pb and other isotopes in the earth’s first created crustal rocks. However, it could be argued that the reason the earth yields the same Pb-Pb “age” as most of the meteorites (for example, Snelling 2014b, c, d, 2015a, b) is that the parent asteroids were created on Creation Day 4 of the same initial materials God used to create the earth and its initial crustal rocks on Creation Days 1–2. Similarly, God made man’s body from the same dust of the earth He used to create the animals. Many studies of the Pb isotopic compositions of ocean island basalts found they define a series of linear arrays corresponding to Pb-Pb isochrons with “ages” of between 1 Ga and 1.5 Ga for what are only recently-erupted lava flows (Sun 1980). The significance of these false Pb-Pb isochron data arrays remains elusive, because they have more radiogenic Pb in them than they should have if the earth’s age is 4.57 Ga (Dickin 2005). This problem has been called the “lead paradox” and still remains unsolved. What is clear is that Pb-Pb dating of these recent basalts produces anomalous old ages that represent the inheritance of the Pb isotopic compositions of the magmas’ mantle sources, perhaps ultimately derived from their primordial composition.
It can also be argued that it is not necessary to postulate grossly accelerated radioactive decay during Creation Days 1–3. That was a time frame in which the Creator’s fiat supernatural activity occurred, before today’s post-Creation-Week operational and thermodynamics rules were instigated to sustain the created order. It is somewhat presumptuous to suggest that God needed to use accelerated radioisotope decay to accompany His process of fiat creation of these rocks. When Jesus the Creator (John 1:1–3; Colossians 1:15–17) turned water into wine (John 2:1–11), His fiat supernatural activity did not necessarily follow today’s thermodynamic rules. We do not explain Jesus’ miracle of His creation of more bread and fish from the five loaves and two fish (Matthew 14: 15–21) by suggesting the bread came from accelerated growth of wheat whose grains were crushed and then baked as bread or fish that hatched and grew at an accelerated speed. So why would we suggest these created schists and granites came into being via accelerated metamorphism and magmatism of precursor rocks? We would then have to explain where those precursor sedimentary and volcanic rocks came from. Eventually at some point in the chain of reasoning we would have to admit God created the first rocks by fiat just as the rocks were. So why not simply accept these schists and granites were created by fiat much as they are, though as seen today likely affected by subsequent events after they were created?
In doing what He did God did not deceive us, no more than Jesus deceived His onlookers. Indeed, God has told us what He did and when He did it, and God does not lie (Titus 1:2). Thus, God tells us when He used progressive processes, such as when He first made man’s body from the dust of the ground and then breathed into his nostrils the breath of life (Genesis 2:7). And God certainly tells us He first formed the earth, the seas, and the heavens before filling them, which constituted a progressive process. However, we do not need to postulate grossly accelerated metamorphic and magmatic processes to transform precursor sediments and volcanics into schists and granites any more than postulating Jesus used grossly accelerated processes to produce more bread and fish. The resultant schists and granites are not testimony to illusionary metamorphism and magmatism. God simply tells us He created the earth and its rocks and then on Creation Day 3 He raised rocks to form the dry land. We are the ones who postulate those rocks were formed by metamorphic and magmatic processes based on our experience and observations today, when God is sustaining the earth and universe (Colossians 1:17), by projecting today’s processes back into the Creation Week and suggesting they must have occurred at a grossly accelerated rate.
So were these granites and schists created by fiat? Or did God use processes of grossly accelerated metamorphism and magmatism? Ultimately we cannot be dogmatic either way as God’s Word is silent, except that God does tell us when He used progressive processes. Jesus used a progressive process when He first put mud on the eyes of a blind man and then sent him to wash the mud off so his sight was healed (John 9:1–7), but for other blind men He simply restored their sight in one supernatural act. At least we can agree that God created supernaturally during the Creation Week, so ultimately these schists and granites were created supernaturally.
The Distribution of Radiohalos
Now that we have discussed the above fundamental issues we can discuss the distribution of radiohalos in these granites. From table 3 it is evident that while the 1840 ± 1 Ma Elves Chasm pluton, the oldest rock unit in Grand Canyon (Ilg et al. 1996; Karlstrom et al. 2003), contains very few radiohalos (0.18 per slide), the highest radiohalos numbers are found in the 1716 ± 0.5 Ma Ruby pluton (7.75 per slide) and the 1740 ± 2 Ma Zoroaster pluton (5.89 per slide). Among the other 1740–1710 granite plutons sampled, the Pipe Creek, Horn Creek, and Trinity plutons have between 2.14 and 3.10 radiohalos per slide, whereas the Diamond Creek pluton has only 0.22 radiohalos per slide and the Grapevine Camp pluton has no radiohalos at all. In contrast, the 1680–1685 Ma Cottonwood Pegmatite complex and the undated Sapphire Pegmatite complex have virtually no radiohalos in them (0.01 and 0.02 radiohalos per slide respectively). These pegmatite complexes would belong to the 1710–1660 Ma group of plutons generated by partial melting of the lower crust of Babcock (1990), Ilg et al. (1996) and Karlstrom et al. (2003). Given that the undated Bright Angel pluton contains 3.65 radiohalos per slide it likely represents another 1740–1710 granite pluton since this group usually contains between 2.14 and 7.75 radiohalos per slide, and geographically it fits in that group because it crops out between the Zoroaster and Horn Creek plutons with 5.89 and 3.10 radiohalos per slide respectively. It is not possible to characterize which group the undated Granite Narrows pluton belongs to but at 0.34 radiohalos per slide it is similar to the 1736 ± 1 Ma Diamond Creek pluton (0.22 radiohalos per slide).
Thus, in this dataset, the supposed age and grouping of a granite pluton is no gauge as to the number of radiohalos it contains. However, a granite pluton’s geographical location potentially is a guide. In table 3 the sampled granite plutons and pegmatite complexes are listed in geographical order, that is, in the order they are encountered rafting downstream along the Colorado River. It is then evident that the plutons of the Upper Granite Gorge from the Zoroaster pluton to the Ruby pluton (river miles 84.6 to 108) generally contain far more radiohalos per slide (2.14–7.75), the Sapphire Pegmatite complex (river miles 99 to 104) being the exception. Otherwise, since there was only one pluton each sampled in the Middle and Lower Granite Gorges, this geographical factor is not statistically relevant.
Next, from table 2 it is evident that even within a pluton the numbers of radiohalos can vary significantly between samples. For example, in the Zoroaster pluton the radiohalo numbers per slide vary in the samples between 0.14 and 18.20. Indeed, wherever there are multiple samples from a pluton similar varying numbers of radiohalos per slide are found—in the Bright Angel pluton (0.0–10.36), Horn Creek pluton (0.02–12.48), Trinity pluton (0.0–5.96), Ruby pluton (0.0–43.98), Elves Chasm pluton (0.0–1.02), Granite Narrows pluton (0.04–2.20), and Diamond Creek pluton (0.0–0.22).
Perhaps an obvious factor is the biotite content of each sample, because the biotite flakes host the radiohalos. Thus, the samples with more biotite flakes would be expected to contain more radiohalos. However, there is no such consistent pattern when table 2 and fig. 8 are compared. For example, comparing the first three samples in fig. 8, (b) ZG-4 from the Pipe Creek pluton obviously contains a lot more biotite than (a) ZG-2 from the Cottonwood Pegmatite complex and (c) ZG-1 from the Horn Creek pluton, which seem to have about the same biotite content. However, from table 2, even though ZG-4 from the Pipe Creek pluton contains 2.64 radiohalos per slide, ZG-1 from the Horn Creek pluton with hardly any biotite in it contains 1.08 radiohalos per slide, while ZG-2 from the Cottonwood Pegmatite complex with also hardly any biotite in it contains 0 radiohalos per slide. Among the five Trinity pluton samples TG-1, 2, 3, 5, and 6 in fig. 8d–h respectively, sample TG-6 (fig. 8h) appears to contain the most biotite, yet it has only 0.60 radiohalos per slide (table 2) compared to sample TG-5 which has 2.02 radiohalos per slide and contains much less biotite. Given that the Ruby pluton samples also contain hornblende (which is also black), the three samples in fig. 8i–k appear to contain similar amounts of biotite flakes, yet (i) sample RP-1 has 6.58 radiohalos per slide and (k) sample RP-3 has 3.46 radiohalos per slide compared to (j) sample RP-2 that has 0 radiohalos per slide. Then while the five Elves Chasm pluton samples in fig. 8l–p would seem to have similar biotite contents, sample ECG-4 (fig. 8n) contains the most radiohalos per slide at 0.76 yet it appears to contain the least biotite, whereas sample ECG-7 (fig. 8o) which clearly contains the most biotite only has 0.36 radiohalos per slide. And finally, while Diamond Creek pluton sample LGG-03 (fig. 8s) obviously contains much more biotite than the other three samples (fig. 8q, r, t), with 0.22 radiohalos per slide it only has marginally more radiohalos than the other three samples which each have 0 radiohalos per slide.
Thus, the amount of biotite in a sample is no guide to radiohalos numbers. However, the generation of radiohalos depends on the 238U decay within zircon crystals that need to be included within the biotite flakes. Sometimes they are not within the biotite flakes, for example, the zircon crystal to the left of center in fig. 10d. Furthermore, the included zircon crystals need to be tiny enough so that all the α-particles from each step in the decay chain escape the zircon crystals and systematically damage the surrounding biotite. However, if the zircon crystals are too large, then only the more energetic α-particles escape from the zircon crystals and just produce a darkened area around them, as in fig. 10c–e. So, the prevalence of zircon crystals included in the biotite flakes and tiny enough to be 238U radiocenters correlates approximately with the resultant radiohalo numbers. Again, there may not be enough radiohalos to be statistically significant, so we can only make inferences.
It can be seen in table 2 that not all samples have 238U radiohalos in them. Indeed, of the 68 samples listed, only 27 (~40%) have 238U radiohalos in them, compared to 46 (~68%) which contain Po radiohalos. Furthermore, of the 27 samples that contain 238U radiohalos, only one sample (Cottonwood Pegmatite complex sample GCG-09) has no accompanying Po radiohalos, and of the remaining 26 samples only one sample (Bright Angel pluton sample GCG-03) has more 238U radiohalos in it than Po radiohalos. Once again, the numbers of 238U radiohalos in samples from the same pluton varies considerably. For example, in the five Zoroaster pluton samples the 238U radiohalo numbers vary from 0 to 192, although this is skewed by the one sample GCG-10 with 192, because the other four samples contain 0, 4, 6, and 26. Similarly, the three Bright Angel pluton samples contain 0, 0, and 269 238U radiohalos, and the ten Trinity pluton samples range from 0 to 29 plus three outliers with 68, 103 and 136 238U radiohalos. Furthermore, seven of the 14 Ruby pluton samples (50%) contain 0 238U radiohalos, while the other seven range from 3 to 28 plus two outliers with 378 and 1004. In contrast, the eight Horn Creek pluton samples contain a narrower spread of 0–78 238U radiohalos (two with 0), as does the five Granite Narrows pluton samples with 0–28 238U radiohalos (two with 0). Only one of the 13 Elves Chasm pluton samples contains 238U radiohalos (27), while the samples from the Grapevine Camp and Diamond Creek plutons do not contain any 238U radiohalos.
Obviously, these variations in 238U radiohalo numbers within multiple samples in these plutons is due to two factors. First is the random spread of suitable tiny zircon crystals included within the varying numbers of biotite flakes per sample with elevated 238U contents sufficient to generate 238U radiohalos. Second is the randomness of the sample collection. There was no guarantee that the locations where the suitably selected samples were collected would contain some or more 238U radiohalos. That is why the multiple samples collected from each pluton provide better statistical assessments of the “fertility” of each granite to have generated 238U radiohalos, and also better comparisons between granites. Thus, by these measures the Ruby pluton is the most “fertile” granite but only has two of its 14 samples with 378 and 1,004 238U radiohalos, a one in seven probability. This compares with the better odds of one “fertile” sample in three from the Bright Angel pluton, but only with 269 238U radiohalos. While the outcomes seem random, so much clearly depends on each granite’s composition, that is, the minerals within it and their proportions.
The Prevalence and Formation of the Po Radiohalos
Next to consider are the numbers of Po radiohalos compared to the numbers of 238U radiohalos and how they vary between samples and granites. Only the Trinity pluton contains any 214Po radiohalos (table 3), and those two 214Po radiohalos are just in one of the ten samples collected (TG-2 in table 2). None of the samples contain any 218Po radiohalos. Otherwise, 46 of the 68 samples in this study (~68%) contain 210Po radiohalos. It is obviously significant that more samples contain 210Po radiohalos than those with 238U radiohalos, and that the samples with both overwhelmingly contain more 210Po radiohalos.
According Gentry (1986, 1988) the Po radiohalos are “God’s fingerprints” of fiat creation, especially the 218Po and 214Po radiohalos because the parent 218Po and 214Po atoms have half-lives of only 3.1 minutes and 164 microseconds. With such fleeting existences the 218Po and 214Po radiohalos that each required 500 million to a billion 218Po or 214Po atoms to α-decay had to form virtually instantly. To Gentry that implied the host biotite flakes and thus the granites had to form virtually instantly, the expected hallmark of fiat creation by God. However, all these granites in Grand Canyon’s Precambrian crystalline basement which many but not all creation geologists believe are created rocks (Austin 1994; Snelling 2009, 2022b), if in the form in which they were created, should according to Gentry’s hypothesis contain many 218Po and 214Po radiohalos. But they do not, which implies that Gentry’s hypothesis is not correct, and/or something has happened to these granites since their creation that has annealed, that is, wiped away, the postulated original 218Po and 214Po radiohalos.
The alternative hydrothermal fluid transport model for the formation of the Po radiohalos (Snelling and Armitage 2003; Snelling 2005) postulates that hydrothermal fluids released from the granite magmas as they crystallized and cooled transported Po atoms from the tiny zircon crystals within biotite flakes in the granites as 238U in them underwent grossly accelerated α-decay to adjacent sites within the biotite flakes where the Po atoms were chemically concentrated into radiocenters that generated the Po radiohalos. While a slower process, Snelling (2008a) argued that the host granites still had to form within 6–10 days while grossly accelerated α-decay was occurring to rapidly generate the billions of Po atoms needed to form the Po radiohalos. And the Po radiohalos had to also form below the 150°C annealing temperature of radiohalos (Laney and Laughlin 1981) and before the hydrothermal fluids had cooled and thus become ineffective in transporting further Po atoms. The predictions and implications of this model have subsequently been tested and verified (Snelling 2008b, c, d, 2014a, 2018; Snelling and Gates 2009).
The prediction of the hydrothermal fluid transport model for Po radiohalo formation would be that ubiquitous 210Po radiohalos would form, with very few or no accompanying 218Po and 214Po radiohalos. Because the hydrothermal fluid process is relatively slower than fiat creation, there would not routinely be sufficient time for enough 218Po and 214Po atoms with half-lives of only 3.1 minutes and 164 microseconds respectively to be transported, nucleate and then α-decay to generate 218Po and 214Po radiohalos. On the other hand, the half-life of 210Po is 138 days. Thus, much more time is available for 210Po atoms to be transported, nucleate and then α-decay to generate 210Po radiohalos, even after the 6–10 days for the host granites to form, as the released hydrothermal fluids would have continued to circulate and cool below 150°C for several more weeks.
The fulfilment of this prediction of the hydrothermal fluids transport model for Po radiohalos formation can be seen in these Grand Canyon Precambrian granite plutons. Of the ten granite plutons sampled in this study, all but one (the Grapevine Camp pluton) contain 210Po radiohalos (table 3), numbers ranging from 11 to 3206. However, due to those numbers being based on different numbers of samples from each pluton, a statistically more relevant abundance measure is the number of 210Po radiohalos per slide. In those nine plutons the numbers of 210Po radiohalos per slide varies from 0.14 in the Elves Chasm pluton to 5.34 in the Ruby pluton (table 3). However, two of these nine granite plutons, the Pipe Creek and Diamond Creek plutons, contain 210Po radiohalos without any accompanying 238U radiohalos. On the other hand, in the seven plutons that contain both 210Po and 238U radiohalos a better statistical comparison between plutons is the ratio of 210Po radiohalos to 238U radiohalos, which ranges from 1.03 to 1 in the Bright Angel pluton to 5.53 to 1 in the Granite Narrows pluton, both plutons only containing moderate numbers of 210Po radiohalos per slide, 1.85 and 0.71 respectively (table 3). In contrast, the two plutons with the highest numbers of abundant 210Po radiohalos per slide, the Ruby pluton (5.34) and Zoroaster pluton (4.98) have widely different numbers of 238U radiohalos and thus different 210Po radiohalos to 238U radiohalos ratios of 2.2 to 1 and 5.45 to 1 respectively (table 3).
As already highlighted, 46 of the 68 samples in this study (~68%) contain 210Po radiohalos, numbers in them ranging from 1 in Horn Creek pluton sample GCG-17 to 1195 in Ruby pluton sample GCG-25 (table 2). However, similarly to the 238U radiohalos, the numbers of 210Po radiohalos in the individual samples vary considerably. For example, the numbers of 210Po radiohalos in the fourteen Ruby pluton samples vary from 0 to 1195, with four samples containing 0, two containing 1195 and 1133, and the other eight between 12 and 316 (table 2). Similarly, the numbers of 210Po radiohalos in the five Zoroaster pluton samples vary from 7 to 728, in the eight Horn Creek pluton samples from 1 to 579, in the ten Trinity pluton samples from 0 to 194, and in the five Granite Narrows pluton samples from 0 to 110 (table 2). In contrast, the numbers of 210Po radiohalos in the thirteen Elves Chasm pluton samples vary from 0 to only 38, with eight samples containing 0, while only one of the four Diamond Creek pluton samples contains 11 210Po radiohalos and the rest none (table 2).
What is also significant is that whereas ten of the fourteen Ruby pluton samples contain 12–1195 210Po radiohalos, only seven of the samples contain 3–1004 238U radiohalos, and whereas four samples contain no radiohalos, three samples only contain 12–66 210Po radiohalos (table 2). Similarly, of the eight Horn Creek pluton samples two contain 210Po radiohalos but no 238U radiohalos, and of the ten Trinity pluton samples four contain 210Po radiohalos but no 238U radiohalos (table 2). Furthermore, of the four Elves Chasm pluton samples that contain 210Po radiohalos only one also contains 238U radiohalos, and only one sample each from the Pipe Creek and Diamond Creek plutons contains only 210Po radiohalos, whereas of the five Zoroaster pluton and five Granite Narrows pluton samples which all contain 210Po radiohalos one and two respectively of those samples contain no 238U radiohalos (table 2).
This detailed elaboration, therefore, clearly shows that many samples from these granite plutons only contain 210Po radiohalos with no 238U radiohalos to accompany them, while other samples with abundant 210Po radiohalos have far fewer 238U radiohalos accompanying them. And only one of the 68 samples contains two 214Po radiohalos, a sample (Trinity pluton sample TG-03) that otherwise only contains 26 210Po radiohalos and no 238U radiohalos (table 2). All these statistics support the claim that the predicted outcome of the hydrothermal fluid transport model for Po radiohalo formation is fulfilled in these granite pluton samples from Grand Canyon’s Precambrian crystalline basement.
When Did These Po Radiohalos Form?
Having established that the prevalence of 210Po radiohalos in samples from these granite plutons is consistent only with the hydrothermal fluid transport model for their formation, the final question to answer is when did these Po radiohalos form? Many, but not all, creation geologists would interpret these Grand Canyon granite plutons in the continent’s crystalline basement foundation rocks as rocks created likely on Days 1 or 2 of the Creation Week before being uplifted and beveled to form the dry land on day 3 (Austin 1994; Snelling 2009, 2022b). In Gentry’s fiat creation model these granites were created by fiat fully-formed with the fully-developed 238U, 218Po, 214Po, and 210Po radiohalos already in them by the end of Creation Day 2 at the latest. So, why then do samples from two of these nine created granite plutons and many samples in the other seven granite plutons now contain many 210Po radiohalos without any accompanying 238U radiohalos? The answer is that we cannot be sure these granite plutons are still today in their original created condition, as subsequent events on Creation Day 3 and then later during the Flood probably changed them. The third possibility that these plutons were generated during the opening moments of the Flood has already been discounted above.
From his tabulated radiohalos data for numerous granites and metamorphic rocks from locations around the globe of various geological ages, both Precambrian and Phanerozoic, Snelling (2023b) clearly showed the peak occurrence of radiohalos was in Flood-related rocks when hydrothermal fluids would be prevalent during catastrophic geological processes. Yet polonium radiohalos were still present, albeit in small numbers, within some Precambrian (pre-Flood) granites. Given that radiohalos are annealed at 150°C (Laney and Laughlin 1981), he thus proposed that any earlier pre-Flood radiohalos in those rocks may have been annealed during the tectonic upheavals of the Flood so that the radiohalos observed in them today were then generated subsequently during the Flood. Even so, any earlier radiohalos could have instead been annealed during the tectonic upheaval of the Creation Day 3 uplift of the initial crustal rocks to form the dry land. Can we determine which scenario is the more likely?
Snelling (2023a) discussed the studies which have been done to determine the temperature of burial of the Cambrian Tapeats Sandstone in Grand Canyon. Because that sandstone layer sits on top of the Great Unconformity and in places rests directly on some of the Precambrian granite plutons sampled in this study, the temperature of burial of the Tapeats would be a guide to the temperature the granite plutons were potentially subjected to during the Flood. Snelling (2023a) detailed the various burial temperature estimation methods used on a tuff bed found within the Tapeats and on the Proterozoic basement rocks, which included zircon and apatite fission-track ages (Dumitru, Duddy, and Green 1994; Kelley, Chapin, and Karlstrom 2001; Kelley and Karlstrom 2012; Naeser et al. 1989, 2001), apatite (U-Th)/He and 4He/3He thermochronology (Flowers et al. 2007, 2009; Flowers, Wernicke, and Farley 2008; Flowers and Farley 2012), and smectite/illite ratios (Essene and Peacor 1995; Hillier et al. 1995; Hower 1981; Huang, Longo, and Pevear 1993; Pollastro 1993; Pytte and Reynolds 1989; Renac and Meunier 1995; Smart and Clayton 1985; Velde and Espitalié 1989; Velde and Lanson 1993). He found that the consensus from all these estimation methods to be that the Tapeats Sandstone, prior to the Laramide deformation responsible for uplifting the Colorado Plateau, was subjected to a burial temperature of 110–130°C, which is below the 150°C annealing temperature of radiohalos (Laney and Laughlin 1981).
However, Peak et al. (2021) and Thurston et al. (2022) used zircon (U-Th)/He thermochronology data obtained from the Precambrian crystalline basement rocks themselves in eastern Grand Canyon to constrain their thermal history. They found that their data and models were highly sensitive to late-stage reheating due to burial beneath ~3–4 km of Phanerozoic strata prior to ca. 60 Ma. Their models that best matched observed date-equivalent uranium trends showed maximum burial temperatures of 140–160°C, which agree with the available apatite (U-Th)/He and apatite fission-track data. While this temperature range straddles the 150°C annealing temperature of radiohalos, it is an estimate of the maximum burial temperature. Thus, coupled with the 110–130°C burial temperature for the overlying Tapeats Sandstone which was also based on some samples from the underlying Proterozoic crystalline basement, the lower temperature of 140°C should be considered the probable maximum burial temperature during the Flood for these Grand Canyon granite plutons. So, any pre-Flood radiohalos in them may not have been annealed during the Flood.
On the other hand, it is possible that the tectonic upheaval on Creation Day 3 responsible for the uplifting of these granites in the original crust created on Creation Days 1–2 would have generated sufficient heat to anneal any radiohalos present in these granites. There was also subsequent burial under the Grand Canyon Supergroup. Thurston et al. (2024) using combined zircon (U-Th)/He and K-feldspar 40Ar/39Ar thermochronometers concluded from their modeling that the Precambrian crystalline basement rocks were at 150–200°C (at 5–8 km depth) until their rapid exhumation to the surface when the Great Unconformity was generated. However, we cannot be certain, because the events of Creation Day 3 also involved supernatural activity that did not necessarily follow today’s thermodynamic rules, just as when Jesus the Creator (John 1:1–3; Colossians 1:15–17) turned water into wine (John 2:1–11). In any case, the lack of 218Po and 214Po radiohalos and the abundance of 210Po radiohalos often without accompanying 238U radiohalos in these Grand Canyon Precambrian granite plutons would seem to rule out Gentry’s fiat creation model for the formation of the Po radiohalos observed today in these rocks.
Instead, we need to examine more closely the applicability of the hydrothermal fluid model for the generation of these radiohalos. Two processes are crucial to the model, namely, grossly accelerated radioactive decay, and generation and circulation of hydrothermal fluids. The grossly accelerated radioactive decay of 238U in zircon inclusions in biotite flakes was needed to generate rapidly the sufficient quantities of the Po isotopes (218Po, 214Po, and 210Po), and the hydrothermal fluids were needed to transport rapidly these Po isotopes to adjacent nucleation sites within biotite flakes which became Po radiocenters that then formed the Po radiohalos around them.
However, the evidence (helium diffusion, radiohalos, fission tracks, discordant isochrons ages by different radioisotope dating methods on the same rock units, and radiocarbon in fossil materials) only points with certainty to accelerated radioactive decay having occurred during the Flood (Vardiman, Snelling, and Chaffin 2005). Indeed, as discussed above, it can be argued that it is not necessary to postulate grossly accelerated radioactive decay during Creation Days 1–3 because that was a time frame in which the Creator’s fiat supernatural activity occurred, before today’s post-Creation-Week operational and thermodynamics rules were instigated to sustain the created order.
And as for the needed hydrothermal fluids to rapidly transport the Po isotopes, those were generated by being expelled from the crystallizing and cooling granite magmas only within plutons that formed during the Flood (Snelling and Woodmorappe 1998; Snelling 2008a). After all, if these Grand Canyon Precambrian granite plutons were created supernaturally by fiat as fully-formed on Creation Days 1–2, then they did not crystallize and cool from granite magmas and thus generate hydrothermal fluids. Furthermore, there would likely have been no generation and intrusion of granite magmas to crystallize and cool as plutons during the geologically quiet and tectonically stable pre-Flood period. Therefore, the hydrothermal fluids needed to transport the Po isotopes to form the Po radiohalos we now observe in these granite plutons had to be generated by other means during the Flood, at the same time as the grossly accelerated radioactive decay was occurring that rapidly produced the needed quantities of Po isotopes.
Two ingredients were readily available during the Flood to produce and circulate hydrothermal fluids, namely, the water that was buried within the sedimentary layers as they were rapidly deposited, and heat generated by the grossly accelerated radioactive decay. Lest the objection be raised that the heat from the grossly accelerated radioactive decay would have been too excessive, the survival of the radiohalos negates that objection as the temperature could not have reached 150°C. Once generated, those hydrothermal fluids could then have circulated down from the sedimentary layers into the underlying Grand Canyon Precambrian crystalline basement. There the hydrothermal fluids would have penetrated into the granite plutons through fractures, around grain boundaries, and then along the cleavage planes within the biotite flakes, where they dissolved the Po isotopes being produced and ejected rapidly from zircon inclusions and rapidly transported them to generate adjacent Po radiohalos.
Ideally, to test this model further granite samples would need to be collected systematically below the Great Unconformity to see if there are more radiohalos in samples closer to the Great Unconformity and along fractures in the granites and then fewer radiohalos further at depth and in the unfractured granites. The depths below the Great Unconformity were not measured for the present set of samples, although some depths can be estimated for some of them. However, that may not provide sufficient data to test this model. A better approach would be to select two or three profiles of granite plutons to progressively sample from the Great Unconformity downwards and along and away from fractures. However, that involves two logistical difficulties, namely, the physical effort to scale down massive cliffs safely, and obtaining the necessary research and sampling permit from the Grand Canyon National Park’s Research Office.
It is admitted that it may seem difficult to envisage water from the overlying sediments permeating down into the crystalline basement rocks which were under hydrostatic pressure. However, water trapped in marine sediments being subducted on top of an underlying oceanic plate in a subduction zone is believed to be expelled at depth into the overlying mantle wedge, which is equally under considerable hydrostatic pressure, but there the water causes partial melting to occur. Thus water must find its way around grains, and along cleavages and fractures, however tight they may be. Such infiltration of metamorphic rocks by hydrothermal fluids has been recognized in granulites in Norway (granular rocks like granites), where earthquakes helped to propel the hydrothermal fluids along shear zones during mountain building (Camacho et al. 2005; Jamtveit, Austrheim, and Malthe-Sorenssen 2000; Jamtveit, Bucher-Nurminen, and Austrheim 1990), early during the Flood and generated 210Po radiohalos in the resultant eclogite (Snelling 2006). Similarly, economic uranium deposits in Canada and Australia found in Precambrian schists beneath unconformities overlain by sandstones at the bases of thick sediment piles have been demonstrated to likely have formed by connate waters in the sandstones becoming hydrothermal fluids with dissolved U in them that permeated downwards across the unconformities and into the underlying schists where they deposited the U in chemically and structurally conductive zones (Marmont 1987). Therefore, if uniformitarians concede descending connate waters can penetrate crystalline rocks, especially when aided by earthquakes, then it would have been clearly achievable during the Flood when geological processes were operating at cataclysmic rates associated with catastrophic plate tectonics (Austin et al. 1994).
This would not have been as effective a process for generating and driving hydrothermal fluid circulation as the expulsion of hydrothermal fluids from crystallizing and cooling granite magmas in plutons, nor would similar large volumes of hydrothermal fluids have been generated. It would also have been a slower process. That conclusion is consistent with the lack of 218Po and 214Po radiohalos, because the fleeting existence of the 218Po and 214Po atoms (half-lives of 3.1 minutes and 164 microseconds respectively) would have decayed before being carried to nucleating 218Po and 214Po radiocenters. It is also consistent with the occurrence of 210Po radiohalos without adjacent 238U radiohalos. This required the hydrothermal fluids to travel further from the sources of the Po isotopes to the distant nucleating 210Po radiocenters, aided by the longer existence of the 210Po atoms due to their 138 day half-life. Indeed, the process of generating these 210Po radiohalos could have continued for several months during the Flood year, until the hydrothermal fluids started cooling and their circulation ceased, particularly in the waning months of the Flood. This process would also have been inefficient, because of the smaller volumes of hydrothermal fluids. Consistent with this is the fewer 210Po radiohalos in these granite plutons compared with granite plutons formed during the Flood (Snelling 2023b) with their abundant Po radiohalos generated from hydrothermal fluids expelled by crystallizing and cooling granite magmas generated during the Flood. It is also consistent with the wide variations in the numbers of 210Po radiohalos between plutons and between samples in each pluton.
Summary and Conclusions
The granite plutons within Grand Canyon’s Precambrian crystalline basement exposed in the Canyon’s inner gorges were likely created supernaturally on Creation Days 1–2 as the foundation rocks for the continental crust. They were then uplifted and beveled as part of the upheaval on Creation Day 3 when God made the dry land. At the onset of the Flood they were beveled further and exposed before the fossil-bearing Flood sediment layers were deposited unconformably over them. Today we observe 238U and 210Po radiohalos in many of these granites plutons. These are a physical record of radioactive decay that resulted in damage to the host biotite flakes by α-particles produced in the 238U decay chain within tiny zircon inclusions. The 210Po radiohalos are enigmatic due to the very short half-life of the parent 210Po atoms and the need for billions of 210Po atoms separated from their 238U decay source. Two models have been proposed previously to resolve that enigma—creation by fiat of the host granites with the Po radiohalos during Creation Days 1–2, or rapid transport of the Po atoms by hydrothermal fluids expelled from rapidly crystallizing granite magmas while grossly accelerated radioactive decay was occurring during the Flood.
Sixty-eight samples were collected from ten of these granite plutons and two pegmatite complexes, and the radiohalos in them identified and statistically counted in prepared microscope slides. Of the 68 samples only 27 (~40%) have 238U radiohalos in them, compared to 46 (~68%) which contain 210Po radiohalos and 22 (~32%) which contain no radiohalos. Thus, 19 samples (~28%) contain 210Po radiohalos with no adjacent 238U radiohalos. And only one sample contains two 214Po radiohalos. Furthermore, there are much fewer radiohalos in these granite plutons, that have been demonstrated from observational evidence to not be granites generated during the Flood, compared to many granite plutons that formed during the Flood.
Since these are supernaturally created granite plutons any radiohalos created in them were subsequently annealed during the Creation Day 3 upheaval and the onset of the Flood. Thus, these data are consistent only with the hydrothermal fluid transport model for the formation of the Po radiohalos during the Flood. Water trapped in the Flood-deposited sediment layers was likely heated by the burial temperatures and by the residual heat generated by grossly accelerated radioactive decay. These hydrothermal fluids circulated down into the underlying basement granite plutons where they rapidly transported Po atoms produced by grossly accelerated decay of the 238U contained in tiny zircon crystals in biotite flakes in the granites and deposited them in nucleating radiocenters that thus generated the Po radiohalos, many without adjacent 238U radiohalos. This was a slower and less effective process that likely lasted months. The 210Po atoms were longer-lived and thus transported further by hydrothermal fluids of lesser volume and less vigorous circulation than hydrothermal fluids expelled by the granite magmas that were generated, intruded, crystallized, and cooled during the Flood.
Acknowledgements
The several research and collecting permits issued by the Grand Canyon National Park that made this study possible are acknowledged and its Research Office staff are thanked. The field support of Tom Vail of Canyon Ministries and of the various crews of Arizona River Runners was essential and much appreciated. Mark Armitage assisted with the preparation of the 50 slides for each sample and the counting of the radiohalos, which assistance was crucial. The use of the Cedarville University Geology Department’s research petrographic microscope is acknowledged. This research was funded initially by the Institute for Creation Research and then subsequently by Answers in Genesis, and by their generous donors who gave specifically for research.
References
Armitage, Mark H., and Andrew A. Snelling. 2008. “Radiohalos and Diamonds: Are Diamonds Really For Ever?” In Proceedings of the Sixth International Conference on Creationism. Edited by Andrew A. Snelling, 323–334. Pittsburgh, Pennsylvania: Creation Science Fellowship; and Dallas, Texas: Institute for Creation Research.
Austin, Steven A. 1994. Grand Canyon: Monument to Catastrophe. Santee, California: Institute for Creation Research.
Austin, Steven A., and Kurt P. Wise. 1994. “The Pre-Flood/ Flood Boundary: As Defined in Grand Canyon and Eastern Mojave Desert, California.” In Proceedings of the Third International Conference on Creationism. Edited by Robert E. Walsh, 37–47. Pittsburgh, Pennsylvania: Creation Science Fellowship.
Austin, Steven A., John R. Baumgardner, D. Russell Humphreys, Andrew A. Snelling, Larry Vardiman, and Kurt P. Wise. 1994. “Catastrophic Plate Tectonics: A Global Flood Model of Earth History.” In Proceedings of the Third International Conference on Creationism. Edited by Robert E. Walsh, 609–621. Pittsburgh, Pennsylvania: Creation Science Fellowship.
Babcock, R. S. 1990. “Precambrian Crystalline Core.” In Grand Canyon Geology. 1st ed. Edited by Stanley S. Beus and Michael Morales, 11–28. New York, New York: Oxford University Press.
Babcock, R. S., E. H. Brown, M. D. Clark, and D. E. Livingston. 1979. “Geology of the Older Precambrian Rocks of the Upper Granite Gorge of the Grand Canyon. Part II. The Zoroaster Plutonic Complex and Related Rocks.” Precambrian Research 8, nos. 3–4 (April): 243–275.
Bagnall, K. W. 1957. Chemistry of the Rare Radioelements: Polonium-Actinium. London, United Kingdom: Butterworths.
Bateman, Paul C., and Bryan W. Chappell. 1979. “Crystallization, Fractionation, and Solidification of the Tuolumne Intrusive Series, Yosemite National Park, California.” Geological Society of America Bulletin 90, no. 5 (May 1): 465–482.
Baumgardner, John R. 2000. “Distribution of Radioactive Isotopes in the Earth.” In Radioisotopes and the Age of the Earth: A Young-Earth Creationist Research Initiative. Edited by Larry Vardiman, Andrew A. Snelling, and Eugene F. Chaffin, 49–94. El Cajon, California: Institute for Creation Research; and St Joseph, Missouri: Creation Research Society.
Bower, William R., Richard A. D. Pattrick, Carolyn I. Pearce, Giles T. R. Droop, and Sarah J. Haigh. 2016a. “Radiation Damage Haloes in Biotite Investigated Using High-Resolution Transmission Electron Microscopy.” American Mineralogist 101, no. 1 (January 1): 105–110.
Bower, William R., Carolyn I. Pearce, Andrew D. Smith, Simon M. Plimblott, J. Frederick W. Mosselmans, Sarah J. Haigh, James P. McKinley, and Richard A. D. Pattrick. 2016b. “Radiation Damage in Biotite Mica by Accelerated α-Particles: A Synchrotron Microfocus X-Ray Diffraction and X-Ray Absorption Spectroscopy Study.” American Mineralogist 101, no. 4 (April 1): 928–942.
Brown, E. H., R. S. Babcock, M. D. Clark, and D. E. Livingston. 1979. “Geology of the Older Precambrian Rocks of the Grand Canyon. Part I. Petrology and Structure of the Vishnu Complex.” Precambrian Research 8, nos. 3–4 (April): 219–241.
Camacho, Alfredo, James K. W. Lee, Bastiaan J. Hensen, and Jean Braun. 2005. “Short-Lived Orogenic Cycles and the Eclogitization of Cold Crust by Spasmodic Hot Fluids.” Nature 435, no. 7046 (30 June): 1191–1196.
Campbell, I., and J. H. Maxson. 1938. “Geological Studies of the Archean Rocks at the Grand Canyon.” Carnegie Institution of Washington Year Book 37: 359–364.
Clarey, Timothy L. 2020. Carved in Stone: Geological Evidence of the Worldwide Flood. Dallas, Texas: Institute for Creation Research.
Clarey, Timothy L., and Davis J. Werner. 2023. “A Progressive Global Flood Model Confirmed by Rock Data Across Five Continents.” In Proceedings of the Ninth International Conference on Creationism. Edited by John H. Whitmore, 412–445. Cedarville, Ohio: Cedarville University International Conference on Creationism.
Dickin, Alan P. 2005. Radiogenic Isotope Geology. 2nd ed. Cambridge, England: Cambridge University Press.
Dumitru, Trevor A., Ian R. Duddy, and Paul F. Green. 1994. “Mesozoic–Cenozoic Burial, Uplift, and Erosion History of the West-Central Colorado Plateau.” Geology 22, no. 6 (June 1): 499–502.
Essene, E. J., and P. R. Peacor. 1995. “Clay Mineral Thermometry—A Critical Perspective.” Clays and Clay Minerals 43, no. 5 (1 October): 540–553.
Flowers, Rebecca M., and Ken A. Farley. 2012. “Apatite 4He/3He and (U-Th)/He Evidence for an Ancient Grand Canyon.” Science 338, no. 6114 (December 21): 1616–1619.
Flowers, Rebecca M., D. L. Shuster, B. P. Wernicke, and Ken A. Farley. 2007. “Radiation Damage Control on Apatite (U-Th)/He Dates from the Grand Canyon Region, Colorado Plateau.” Geology 35, no. 5 (May 1): 447–450.
Flowers, Rebecca M., Richard A. Ketcham, David L. Shuster, and Kenneth A. Farley. 2009. “Apatite (U–Th)/He Thermochronometry Using a Radiation Damage Accumulation and Annealing Model.” Geochimica et Cosmochimica Acta 73, no. 8 (15 April): 2347–2365.
Flowers, Rebecca M., B. P. Wernicke, and Ken A. Farley. 2008. “Unroofing, Incision and Uplift History of the Southwestern Colorado Plateau from Apatite (U-Th)/He Thermochronometry.” Geological Society of America Bulletin 120, nos. 5–5 (May): 571–587.
Fremlin, J. H. 1975. “Spectacle Haloes.” Nature 258, no. 5532 (November): 269.
Gentry, Robert V. 1967. “Extinct Radioactivity and the Discovery of a New Pleochroic Halo.” Nature 213, no. 5075 (4 February): 487–489.
Gentry, Robert V. 1968. “Fossil Alpha-Recoil Analysis of Certain Variant Radioactive Halos.” Science 160, no. 3833 (14 June): 1228–1230.
Gentry, Robert V. 1970. “Giant Radioactive Halos: Indicators of Unknown Radioactivity.” Science 169, no. 3946 (14 August): 670–673.
Gentry, Robert V. 1971. “Radiohalos: Some Unique Lead Isotopic Ratios and Unknown Alpha Radioactivity.” Science 173, no. 3998 (20 August): 727–731.
Gentry, Robert V. 1973. “Radioactive Halos.” Annual Review of Nuclear Science 23, no. 1 (December): 347–362.
Gentry, Robert V. 1974. “Radiohalos in a Radiochronological and Cosmological Perspective.” Science 184, no. 4132 (April 5): 62–66.
Gentry, Robert V. 1975. “Spectacle Haloes (Reply).” Nature 258 (20 November): 269–270.
Gentry, Robert V. 1979. “Time: Measured Responses.” EOS, Transactions of the American Geophysical Union 60, no. 22: 474.
Gentry, Robert V. 1980. “Polonium Halos.” EOS, Transactions of the American Geophysical Union 61, no. 27 (1 July): 514.
Gentry, Robert V. 1982. “Creationism Again.” Physics Today 35, no. 10 (October): 13.
Gentry, Robert V. 1983. “Creationism Still Again.” Physics Today 36, no. 4 (April): 13–-15.
Gentry, Robert V. 1984. “Radioactive Halos in a Radiochronological and Cosmological Perspective.” In Proceedings of the 63rd Annual Meeting of the Pacific Division, American Association for the Advancement of Science 1, no. 3: 38–65.
Gentry, Robert V. 1986. “Radioactive Halos: Implications for Creation.” In Proceedings of the First International Conference on Creationism. Vol. 2. Edited by Robert E. Walsh, Christopher L. Brooks, and R. S. Crowell, 89–100. Pittsburgh, Pennsylvania: Creation Science Fellowship.
Gentry, Robert V. 1988. Creation’s Tiny Mystery. Knoxville, Tennessee: Earth Science Associates.
Gentry, Robert V. 1989. “Response to Wise.” Creation Research Society Quarterly 25, no. 4 (March): 176–180.
Gentry, Robert V. 1998. “Radiohalos in Diamonds.” Creation Ex Nihilo Technical Journal 12, no. 3 (December): 287–290.
Gentry, Robert V., T. A. Cahill, N. R. Fletcher, H. C. Kaufmann, L. R. Medsker, J. W. Nelson, and R. G. Flocchini. 1976. “Evidence for Primordial Superheavy Elements.” Physical Review Letters 37, no. 11 (5 July): 11–15.
Gentry, Robert V., W. H. Christie, D. H. Smith, J. W. Boyle, S. S. Cristy, and J. F. McLaughlin. 1978. “Implications on Unknown Radioactivity of Giant and Dwarf Haloes in Scandinavian Rocks.” Nature 274, no. 5670 (3 August): 457–459.
Gentry, Robert V., S. S. Cristy, J. F. McLaughlin, and J. A. McHugh. 1973. “Ion Microprobe Confirmation of Pb Isotope Ratios and Search for Isomer Precursors in Polonium Radiohaloes.” Nature 244, no. 5414 (3 August): 282–283.
Gentry, Robert V., L. D. Hulett, S. S. Cristy, J. F. McLaughlin, J. A. McHugh, and Michael Bayard. 1974. “‘Spectacle’ Array of 210Po Halo Radiocentres in Biotite: A Nuclear Geophysical Enigma.” Nature 252, no. 5484 (13 December): 564–566.
Glazner, Allen F., and Breck R. Johnson. 2013. “Late Crystallization of K-Feldspar and the Paradox of Megacrystic Granites.” Contributions to Mineralogy and Petrology 166, no. 3 (September): 777–779.
Glazner, Allen F., Victor R. Baker, John M. Bartley, Kevin M. Bohacs, and Drew R. Coleman. 2022. “The Rocks Don’t Lie, But They can be Misunderstood.” Geology Today 32, no. 10 (October): 4–10.
Harada, Koh, William C. Burnett, Paul A. LaRock, and James B. Cowart. 1989. “Polonium in Florida Groundwater and its Possible Relationship to the Sulfur Cycle and Bacteria.” Geochimica et Cosmochimica Acta 53, no. 1 (January): 143–150.
Hawkins, David P., Samuel A. Bowring, Bradley R. Ilg, Karl E. Karlstrom, and Michael L. Williams. 1996. “U-Pb Geochronological Constraints on Paleoproterozoic Crustal Evolution of the Upper Granite Gorge, Grand Canyon, Arizona.” Geological Society of America Bulletin 108, no. 9 (September 1): 1167–1181.
Henderson, George Hugh. 1939. “A Quantitative Study of Pleochroic Haloes—V. The Genesis of Haloes.” Proceedings of the Royal Society of London, Series A 173, no. 953 (28 November): 250–264.
Henderson, George Hugh, and S. Bateson. 1934. “A Quantitative Study of Pleochroic Haloes—I.” Proceedings of the Royal Society of London, Series A 145, no. 855 (2 July): 563–581.
Henderson, George Hugh, C. M. Mushkat, and D. P. Crawford. 1934. “A Quantitative Study of Pleochroic Haloes—III Thorium.” Proceedings of the Royal Society of London, Series A 158, no. 893 (1 January): 199–211.
Henderson, George Hugh, and F. W. Sparks. 1939. “A Quantitative Study of Pleochroic Haloes—IV. New Types of Haloes.” Proceedings of the Royal Society of London, Series A 173, no. 953 (28 November): 238–249.
Henderson, G. H., and L. G. Turnbull. 1934. “A Quantitative Study of Pleochroic Haloes—II.” Proceedings of the Royal Society of London, Series A 145, no. 855 (2 July): 582–598.
Hillier, S., J. Mátyás, A. Matter, and G. Vasseur. 1995. “Illite/Smectite Diagenesis and Its Variable Correlation with Vitrinite Reflectance in the Pannonian Basin.” Clays and Clay Minerals 43, no. 2 (1 April): 174–183.
Holmes, Arthur. 1931. “Radioactivity and Geological Time.” In Physics of the Earth—IV. The Age of the Earth. Bulletin of the National Research Council 80: 124–460.
Hower, J. 1981. “Shale Diagenesis.” In Clays and the Resource Geologist. Edited by F. J. Longstaffe, 60–80. Quebec City, Canada: Mineralogical Association of Canada, Short Course Handbook 7.
Huang, Wuu-Liang, John M. Longo, and David R. Pevear. 1993. “An Experimentally Derived Kinetic Model for Smectite-to-Illite Conversion and Its Use as a Geothermometer.” Clays and Clay Minerals 41, no. 2 (1 April): 162–177.
Humphreys, D. Russell. 2000. “Accelerated Nuclear Decay: A Viable Hypothesis?” In Radioisotopes and the Age of the Earth: A Young-Earth Creationist Research Initiative. Edited by Larry Vardiman, Andrew A. Snelling, and Eugene F. Chaffin, 333–379. El Cajon, California: Institute for Creation Research; and St Joseph, Missouri: Creation Research Society.
Humphreys, D. Russell. 2005. “Young Helium Diffusion Age of Zircons Supports Accelerated Nuclear Decay.” In Radioisotopes and the Age of the Earth: Results of a Young-Earth Creationist Research Initiative. Edited by Larry Vardiman, Andrew A. Snelling, and Eugene F. Chaffin, 25–100. El Cajon, California: Institute for Creation Research, and Chino Valley, Arizona: Creation Research Society.
Hussain, N., T. M. Church, G. W. Luther III, and W. S. Moore. 1995. “210Po and 210Pb Disequilibrium in the Hydrothermal Vent Fluids in Chimney Deposits from Juan de Fuca Ridge.” Geophysical Research Letters 22, no. 23 (1 December): 3175–3178.
Iimori, S., and J. Yoshimura. 1926. “Pleochroic Halos in Biotite: Probable Existence of the Independent Origin of the Actinium Series.” Scientific Papers of the Institute of Physical and Chemical Research 5, no. 66: 11–24.
Ilg, Bradley R., Karl E. Karlstrom, David P. Hawkins, and Michael L. Williams. 1996. “Tectonic Evolution of Paleoproterozoic Rocks in the Grand Canyon: Insights into Middle-Crustal Processes.” Geological Society of America Bulletin 108, no. 9 (September 1): 1149–1166.
Jamtveit, Bjørn, Håkon Austrheim, and Anders Malthe-Sørenssen. 2000. “Accelerated Hydration of the Earth’s Deep Crust Induced by Stress Perturbations.” Nature 408, no. 6808 (2 November): 75–78.
Jamtveit, Bjørn, Kurt Bucher-Nurminen, and Håkon Austrheim. 1990. “Fluid Controlled Eclogitization of Granuliites in Deep Crustal Shear Zones, Bergen Arcs, Western Norway.” Contributions to Mineralogy and Petrology 104, no. 2 (February): 184–193.
Joly, J. 1907. “Pleochroic Halos.” Philosophical Magazine 13, no. 75: 381–383.
Joly, J. 1917a. “Radio-Active Halos.” Philosophical Transactions of the Royal Society of London, Series A 217: 51–79.
Joly, J. 1917b. “Radio-Active Halos.” Nature 99, no. 2492 (2 August): 456–458, 476–478.
Joly, J. 1923. “Pleochroic Haloes of Various Geological Ages.” Proceedings of the Royal Society of London, Series A 102, no. 719 (March 1): 682–705.
Joly, J. 1924. “The Radioactivity of the Rocks.” Nature 114, no. 2857 (2 August): 160–164.
Karlstrom Karl E., Bradley R. Ilg, Michael L. Williams, David P. Hawkins, Samuel A. Bowring, and S. J. Seaman. 2003. “Paleoproterozoic Rocks of the Granite Gorges.” In Grand Canyon Geology. 2nd ed. Edited by Stanley S. Beus, and Michael Morales, 9–38. New York, New York: Oxford University Press.
Karlstrom Karl E., Bradley R. Ilg, David P. Hawkins, Michael L. Williams, Gregory Dumond, Kevin Mahan, and Samuel A. Bowring. 2012. “Vishnu Basement Rocks of the Upper Granite Gorge: Continent Formation 1.84 to 1.66 Billion Years Ago.” In Grand Canyon Geology: Two Billion Years of Earth’s History. Edited by J. Michael Timmons, and Karl E. Karlstrom, 7–24. Boulder, Colorado: Geological Society of America, Special Paper 489.
Karlstrom, Karl E., and J. Michael Timmons. 2012. “Many Unconformities Make One ‘Great Unconformity’.” In Grand Canyon Geology: Two Billion Years of Earth’s History. Edited by J. Michael Timmons, and Karl E. Karlstrom, 73–79. Boulder, Colorado: Geological Society of America, Special Paper 489.
Kelley, Shari A., Charles E. Chapin, and Karl E. Karlstrom. 2001. “Laramide Cooling Histories of Grand Canyon, Arizona, and the Front Range, Colorado, Determined from Apatite Fission-Track Thermochronology.” In Colorado River: Origin and Evolution. Edited by Richard A. Young, and Earle E. Spamer, 37–42. Grand Canyon, Arizona: Grand Canyon Association, Monograph 12.
Kelley, Shari A., and Karl E. Karlstrom. 2012. “The Laramide and Post-Laramide Uplift and Erosional History of the Eastern Grand Canyon: Evidence from Apatite Fission-Track Thermochronology.” In Grand Canyon Geology: Two Billion Years of Earth’s History. Edited by J. Michael Timmons, and Karl E. Karlstrom, 109–117. Boulder, Colorado: Geological Society of America, Special Paper 489.
Kerr-Lawson, D. E. 1927. “Pleochroic Haloes in Biotite from near Murray Bay.” University of Toronto Studies in Geology Series 24: 54–71.
Kerr-Lawson, D. E. 1928. “Pleochroic Haloes in Biotite.” University of Toronto Studies in Geology Series 27: 15–27.
Laney, Randy, and A. William Laughlin. 1981. “Natural Annealing of Pleochroic Haloes in Biotite Samples from Deep Drill Holes, Fenton Hill, New Mexico.” Geophysical Research Letters 8, no. 5 (May): 501–504.
Larock, P. A., J.-H. Hyun, S. Boutelle, W. C. Burnett, and C. D. Hull. 1996. “Bacterial Mobilization of Polonium.” Geochimica et Cosmochimica Acta 60, no. 22 (November): 4321–4328.
LeCloarec, M. F., M. Pennisi, E. Corazza, and G. Lambert. 1994. “Origin of Fumarolic fluids Emitted from a Nonerupting Volcano: Radionuclide Constraints at Vulcano (Aeolian Islands, Italy).” Geochimica et Cosmochimica Acta 58, no. 20 (October): 4401–4410.
Lingen, J.S., van der. 1926. “Ueber Pleochroitische Höfe.” Zentralel. Mineralogie und Geologie, Abteilung A 177–183.
Marmont, Soussan. 1987. “Ore Deposit Models #13. Unconformity-Type Uranium Deposits.” Geoscience Canada 14, no. 4 (December 12): 219–229.
Mügge, O. 1907. “Radioaktivität als Ursuche der Pleochroitischen Höfe des Cordierite.” Zentralel. Mineralogie und Geologie: 397–399.
Naeser, Chris W., Ian R. Duddy, Donald P. Elston, Terry A. Dumitru, and Paul F. Green. 1989. “Fission-Track Dating: Ages for Cambrian Strata and Laramide and Post-Middle Eocene Cooling Events from the Grand Canyon, Arizona.” In Geology of Grand Canyon, Northern Arizona (with Colorado River Guides). Edited by Donald P. Elston, George H. Billingsley, and Richard A. Young, 139–144. Washington, DC: American Geophysical Union.
Naeser, Chris W., Ian R. Duddy, Donald P. Elston, Terry A. Dumitru, and Paul F. Green. 2001. “Fission-Track Analysis of Apatite and Zircon from Grand Canyon, Arizona.” In Colorado River: Origin and Evolution. Edited by Richard A. Young, and Earle E. Spamer, 31–36. Grand Canyon, Arizona: Grand Canyon Association, Monograph 12.
Noble, Levi F., and J.F. Hunter. 1916. “A Reconnaissance of the Archean Complex of the Granite Gorge, Grand Canyon, Arizona.” US Geological Survey Professional Paper 98-I: 95–113.
Peak, B. A., Rebecca M. Flowers, F. A. Macdonald, and J. M. Cottle. 2021. “Zircon (U-Th)/He Thermochronology Reveals Pre-Great Unconformity Paleotopography in the Grand Canyon Region, USA.” Geology 49, no. 12 (August 12): 1462–146.
Peters, Shanan E., and Robert R. Gaines. 2012. “Formation of the ‘Great Unconformity’ as a Trigger for the Cambrian Explosion.” Nature 484, no. 7394 (18 April): 363–366
Pollastro, Richard M. 1993. “Considerations and Applications of the Illite/Smectite Geothermometer in Hydrocarbon-Bearing Rocks of Miocene to Mississippian Age.” Clays and Clay Minerals 41, no. 2 (April): 119–133.
Powell, John Wesley. 1876. Exploration of the Colorado River of the West. Washington, D.C.: Smithsonian Institution.
Pytte, A. M., and R. C. Reynolds. 1989. “The Thermal Transformation of Smectite to Illite.” In Thermal History of Sedimentary Basins. Edited by N. D. Naeser, and T. H. McCulloh, 133–140. Berlin, Germany: Springer-Verlag.
Ramdohr, Paul. 1933. “Ueber die Blaue Kuppe bei Eschwege und benach-barte Basaltvorkommen.” Neues Jahrbuch für Mineralogie Beilageband Abteilung A 67: 53–65.
Ramdohr, Paul. 1957. “Neue Beobachtungen über Radioaktive Höfe und über Radioaktive Sprengungen.” Abhandlungen der Deutschen Akademie der Wissenschaften zu Berlin, Klasse für Chemie, Geologie und Biologie 2: 1–17.
Ramdohr, Paul. 1960. “Neue Beobachtungen an Radioacktiven Höfen in Verschiedenen Mineralien mit Kritischen Bemerkungen zur Auswertung der Höfe zur Altersbestimmung.” Geologische Rundschau 49 (November): 253–263.
Renac, C., and A. Meunier. 1995. “Reconstruction of Palaeothermal Conditions in the Passive Margin Using Illite-Smectite Mixed-Layer Series (BA1 Scientific Deep Drill-Hole, Ardeche, France).” Clay Minerals 30, no. 2 (June): 107–118.
Rubin, Ken. 1997. “Degassing of Metals and Metalloids from Erupting Seamount and Mid-Ocean Ridge Volcanoes: Observations and Predictions.” Geochimica et Cosmochimica Acta 61, no. 17 (September): 3525–3542.
Schilling, A. 1926. “Die Radioacktiven Höfe im Flußspat von Wölsendorf.” Neues Jahrbuch für Mineralogie, Geologie und Paläeontology, Abteilung A 53: 241–265.
Schulze, Daniel J., and Lutz Nasdala. 2017. “Unusual Paired Pattern of Radiohaloes on a Diamond Crystal from Guaniamo (Venezuela).” Lithos 265 (15 November): 177–181.
Smart, G., and T. Clayton. 1985. “The Progressive Illitization of Interstratified Illite-Smectite from Carboniferous Sediments of Northern England and its Relationship to Organic Maturity Indicators.” Clay Minerals 30, no. 4 (December): 455–466.
Snelling, Andrew A. 2000. “Radiohalos.” In Radioisotopes and the Age of the Earth: A Young-Earth Creationist Research Initiative. Edited by Larry Vardiman, Andrew A. Snelling, and Eugene F. Chaffin, 381–468. El Cajon, California: Institute for Creation Research, and St. Joseph, Missouri: Creation Research Society.
Snelling, Andrew A. 2005. “Radiohalos in Granites: Evidence for Accelerated Nuclear Decay.” In Radioisotopes and the Age of the Earth: Results of a Young-Earth Creationist Research Initiative. Edited by Larry Vardiman, Andrew A. Snelling, and Eugene F. Chaffin, 101–207. El Cajon, California: Institute for Creation Research, and Chino Valley, Arizona: Creation Research Society.
Snelling, Andrew A. 2006. “Confirmation of Rapid Metamorphism of Rocks.” Acts and Facts 35, no. 2 (February 1): Impact Article #392
Snelling, Andrew A. 2008a “Catastrophic Granite Formation: Rapid Melting of Source Rocks, and Rapid Magma Intrusion and Cooling.” Answers Research Journal 1 (February 6): 11–25. https://answersresearchjournal.org/catastrophic-granite-formation/.
Snelling, Andrew A. 2008b. “Testing the Hydrothermal Fluid Transport Model for Polonium Radiohalo Formation: The Thunderhead Sandstone, Great Smoky Mountains, Tennessee-North Carolina.” Answers Research Journal 1 (March 26): 53–64. https://answersresearchjournal.org/polonium-radiohalo-testing/.
Snelling, Andrew A. 2008c. “Radiohalos in the Cooma Metamorphic Complex, New South Wales, Australia: The Mode and Rate of Regional Metamorphism.” In Proceedings of the Sixth International Conference on Creationism. Edited by Andrew A. Snelling, 371–387. Pittsburgh, Pennsylvania: Creation Science Fellowship, and Dallas, Texas: Institute for Creation Research.
Snelling, Andrew A. 2008d. “Radiohalos in the Shap Granite, Lake District, England: Evidence that Removes Objections to Flood Geology.” In Proceedings of the Sixth International Conference on Creationism. Edited by Andrew A. Snelling, 389–405. Pittsburgh, Pennsylvania: Creation Science Fellowship, and Dallas, Texas: Institute for Creation Research.
Snelling, Andrew A. 2009. Earth’s Catastrophic Past: Geology, Creation and the Flood. Dallas, Texas: Institute for Creation Research.
Snelling, Andrew A. 2014a. “Radiohalos in Multiple, Sequentially Intruded Phases of the Bathurst Batholith, NSW, Australia: Evidence for Rapid Granite Formation during the Flood.” Answers Research Journal 7 (March 5): 49–81. https://answersresearchjournal.org/radiohalos-bathurst-batholith-australia/
Snelling, Andrew A. 2014b. “Radioisotope Dating of Meteorites: I. The Allende CV3 Carbonaceous Chondrite.” Answers Research Journal 7 (April 16): 103–145. https://answersresearchjournal.org/radioisotope-dating-meteorites-1/.
Snelling, Andrew A. 2014c. “Radioisotope Dating of Meteorites II: The Ordinary and Enstatite Chondrites.” Answers Research Journal 7 (August 20): 239–296. https://answersresearchjournal.org/radioisotope-dating-chondrites-2/.
Snelling, Andrew A. 2014d. “Radioisotope Dating of Meteorites: III. The Eucrites (Basaltic Achondrites).” Answers Research Journal 7 (December 31): 533–583. https://answersresearchjournal.org/radioisotope-dating-meteorites-3/.
Snelling, Andrew A. 2015a. “Radioisotope Dating of Meteorites: IV. The Primitive and Other Achondrites.” Answers Research Journal 8 (May 6): 209–251. https://answersresearchjournal.org/radioisotope-dating-meteorites-4/.
Snelling, Andrew A. 2015b. “Radioisotope Dating of Meteorites: V. Isochron Ages of Groups of Meteorites.” Answers Research Journal 8 (December 16): 449–478. https://answersresearchjournal.org/radioisotope-dating-meteorites-5/.
Snelling, Andrew A. 2018. “Radiohalos as an Exploration Pathfinder for Granite-Related Hydrothermal Ore Veins: A Case Study in the New England Batholith, Eastern Australia.” In Proceedings of the Eighth International Conference on Creationism. Edited by John H. Whitmore, 567–580. Pittsburgh, Pennsylvania: Creation Science Fellowship.
Snelling, Andrew A. 2021a. “The Petrology of the Tapeats Sandstone, Tonto Group, Grand Canyon, Arizona.” Answers Research Journal 14 (June 23): 159–254. https://answersresearchjournal.org/petrology-tapeats-sandstone-tonto-group/.
Snelling, Andrew A. 2021b. “The Petrology of the Bright Angel Formation, Tonto Group, Grand Canyon, Arizona.” Answers Research Journal 14 (September 8): 303–415. https://answersresearchjournal.org/petrology-bright-angel-tonto-group/.
Snelling, Andrew A. 2022a. “The Petrology of the Muav Formation, Tonto Group, Grand Canyon, Arizona.” Answers Research Journal 15 (August 10): 139–262. https://answersresearchjournal.org/geology/petrology-muav-formation-tonto-group/.
Snelling, Andrew A. 2022b. The Genesis Flood Revisited. Green Forest, Arkansas: Master Books; and Hebron, Kentucky: Answers in Genesis.
Snelling, Andrew A. 2023a. “The Carbon Canyon Fold, Eastern Grand Canyon, Arizona.” Answers Research Journal 16 (February 22): 1–124. https://answersresearchjournal.org/geology/carbon-canyon-fold-arizona/.
Snelling, Andrew A. 2023b. “Radiohalos Through Earth History—What Clues Can They Provide Us?” In Proceedings of the Ninth International Conference on Creationism. Edited by John H. Whitmore, 540-560. Cedarville, Ohio: Cedarville University International Conference on Creationism.
Snelling, Andrew A., and Mark H. Armitage. 2003. “Radiohalos: A Tale of Three Granitic Plutons.” In Proceedings of the Fifth International Conference on Creationism. Edited by Robert L. Ivey, 243–267. Pittsburgh, Pennsylvania: Creation Science Fellowship.
Snelling, Andrew A., John R. Baumgardner, and Larry Vardiman. 2003. “Abundant Po Radiohalos in Phanerozoic Granites and Timescale Implications for their Formation.” EOS, Transactions of the American Geophysical Union 84, no. 46, Fall Meeting Supplement, Abstract V32C-1046.
Snelling, Andrew A., and Dallel Gates. 2009. “Implications of Polonium Radiohalos in Nested Plutons of the Tuolumne Intrusive Suite, Yosemite, California.” Answers Research Journal 2 (April 8): 53–77. https://answersresearchjournal.org/polonium-radiohalos-yosemite/.
Snelling, Andrew A., and John Woodmorappe. 1998. “The Cooling of Thick Igneous Bodies on a Young Earth.” In Proceedings of the Fourth International Conference on Creationism. Edited by Robert E. Walsh, 527–545. Pittsburgh, Pennsylvania: Creation Science Fellowship.
Stark, M. 1936. “Pleochroitische (Radioaktive) Höfe ihre Verbreitung in den Gesteinen und Veränderlickheit.” Chemie der Erde 10: 566–630.
Sun, S. S. 1980. “Lead Isotopic Study of Young Volcanic Rocks from Mid-Ocean Ridges, Ocean Islands and Island Arcs.” Philosophical Transactions of the Royal Society A 297, no. 1431 (24 July): 409–440.
Thurston, Olivia G., William R. Guenthner, Karl E. Karlstrom, Jason W. Ricketts, Matthew T. Heizler, and J. Michael Timmons. 2022. “Zircon (U-Th)/He Thermochronology of Grand Canyon Resolves 1250 Ma Unroofing at the Great Unconformity and <20 Ma Canyon Carving.” Geology 50, no. 2 (November 2): 222–226.
Thurston, Olivia G., William R. Guenthner, Karl E. Karlstrom, Matthew T. Heizler, Jason W. Ricketts, and K. T. McDannell. 2024. “Deep-Time Thermal History of the Great Unconformity in the Grand Canyon, USA: Combined Zircon (U-Th)/He and K-feldspar 40Ar/39Ar Thermochronometers.” Geological Society of America Bulletin 136, nos. 11–12 (May 6): 4815–4835.
Timmons, J. Michael, John Bloch, Kathryn Fletcher, Karl E. Karlstrom, Matthew T. Heizler, and Laura J. Crossey. 2012. “The Grand Canyon Unkar Group: Mesoproterozoic Basin Formation in the Continental Interior during Supercontinent Assembly.” In Grand Canyon Geology: Two Billion Years of Earth’s History. Edited by J. Michael Timmons, and Karl E. Karlstrom, 25–47. Boulder, Colorado: Geological Society of America, Special Paper 489.
Timmons, J. Michael, Karl E. Karlstrom, Matthew T. Heizler, Samuel A. Bowring, George E. Gehrels, and Laura J. Crossey. 2005. “Tectonic Inferences from the ca. 1254–1100 Ma Unkar Group and Nankoweap Formation, Grand Canyon: Intracratonic Deformation and Basin Formation During Protracted Grenville Orogenesis.” Geological Society of America Bulletin 117, nos. 11–12 (November 1): 1573–1595.
Vardiman, Larry, Andrew A. Snelling, and Eugene F. Chaffin (editors). 2005. Radioisotopes and the Age of the Earth: Results of a Young-Earth Creationist Research Initiative. El Cajon, California: Institute for Creation Research, and Chino Valley, Arizona: Creation Research Society.
Velde, B., and J. Espitalié. 1989. “Comparison of Kerogen Maturation and Illite/Smectite Composition in Diagenesis.” Journal of Petroleum Geology 12, no. 1 (January): 103–110.
Velde, B., and B. Lanson. 1993. “Comparison of I/S Transformation and Maturity of Organic Matter at Elevated Temperatures.” Clays and Clay Minerals 41, no. 2 (1 April): 178–183.
Walcott, C. D. 1889. “A Study of a Line of Displacement in the Grand Canyon of the Colorado in Northern Arizona.” Geological Society of America Bulletin 1: 49-64.
Whitmore, John H., Raymond Strom, Stephen Cheung, and Paul A. Garner. 2014. “The Petrology of the Coconino Sandstone (Permian), Arizona, USA.” Answers Research Journal 7 (December 10): 499–532. https://answersresearchjournal.org/petrology-of-the-coconino-sandstone/.
Whitmore, John H., and Paul A. Garner. 2018. “The Coconino Sandstone (Permian, Arizona, USA): Implications for the Origin of Ancient Cross-Bedded Sandstones.” In Proceedings of the Eighth International Conference on Creationism, Edited by John H. Whitmore, 581–627. Pittsburgh, Pennsylvania: Creation Science Fellowship.
Wiman, E. 1930. “Studies of Some Archaean Rocks in the Neighbourhood of Uppsala, Sweden, and their Geological Position.” Bulletin of the Geological Institute, University of Uppsala 23: 1–170.
Wise, Kurt P. 1989. “Radioactive Halos: Geological Concerns.” Creation Research Society Quarterly 25, no. 4 (March): 171–176.
Wise, Kurt P., and Andrew A. Snelling. 2005. “A Note on the Pre-Flood/Flood Boundary in the Grand Canyon.” Origins 58 (June 1): 7–29.