Research conducted by Answers in Genesis staff scientists or sponsored by Answers in Genesis is funded solely by supporters’ donations.
Abstract
The meta-arkoses of the Thunderhead Sandstone in the Great Smoky Mountains of Tennessee and North Carolina contain both detrital zircon grains and metamorphic biotite flakes in all zones of regional metamorphism. The original sandstones after deposition would have contained water. Furthermore, the reaction responsible for the mineralogical changes at the staurolite isograd would have produced large volumes of water. Thus it was predicted that during the regional metamorphism these waters as hydrothermal fluids should have transported Po from the zircon grains to the biotite flakes to generate Po radiohalos in the latter. Also, the greater volume of hydrothermal fluids at the staurolite isograd should have generated more Po radiohalos there. Both predictions were verified, with four–five times more Po radiohalos in the meta-arkoses straddling the staurolite isograd. These results also verify the hydrothermal fluid transport model for Po radiohalo formation. It was concluded that the regional metamorphism, the hydrothermal fluid flows, the cooling of the regional metamorphic complex, and the formation of the Po radiohalos all had to have occurred within a few weeks. This is feasible in the context of catastrophic plate tectonics and grossly accelerated 238U decay during the Genesis Flood.
Keywords: Po radiohalos, 238U decay, hydrothermal fluid transport model, sandstones, regional metamorphism, meta-arkoses, detrital zircon grains, metamorphic biotite flakes, staurotile isograd, mineral reaction
Introduction
Radiohalos research was a major focus of the RATE (Radioisotopes and the Age of The Earth) project (Snelling 2000). As a result of this research it was concluded that the 238U and Po radiohalos frequently found together in biotite flakes in granitic rocks had to have formed simultaneously (Snelling 2005). Because of the very short half-lives of the parent Po isotopes, this implies that hundreds of million of years worth of 238U decay (at today’s rates) had to have instead occurred in only a matter of a few days. There needs to have been that much decay of 238U to produce both the visible physical damage (the 238U radiohalos) and the 500 million–1 billion polonium atoms required to generate the polonium radiohalos. However, that much polonium would then have decayed within a few days. A hydrothermal fluid transport model was thus proposed which explains how the polonium was separated from its parent 238U, transported very short distances, and then concentrated in radiocenters close by to form the polonium radiohalos (Snelling and Armitage 2003; Snelling, Baumgardner, and Vardiman 2003; Snelling 2005).
Another outcome of this research was the discovery of plentiful polonium radiohalos in metamorphic rocks (Snelling 2005), an occurrence not previously documented. However, such a finding was predicted, because hydrothermal fluids are generated in water-saturated sedimentary rocks as they become deeply buried, helping to transform them into regional metamorphic complexes (Stanton 1982, 1989; Snelling 1994). Thus it was argued that the same hydrothermal fluid transport model could likewise explain the formation of polonium radiohalos in those regional metamorphic rocks where an adequate supply of 238U decay products was available (Snelling 2005).
In continued research, a test of this polonium radiohalo formation model in metamorphic rocks was proposed, and reasoned as follows. Sandstones often contain some zircon grains, derived from erosion of, for example, granitic rocks and deposited in water-transported sandy sediments. Chemical weathering of such source rocks, plus abrasion of grains during water transport, destroys biotite grains, so they tend to be absent in sandstones. However, after metamorphism of sandstones, the resultant schists and gneisses usually contain biotite grains. Thus it would seem that the biotite grains formed via mineral reactions during the metamorphism. Such mineral reactions have been studied in laboratory experiments, and in them water is often a by-product (Spear 1993). At the temperatures of these metamorphic processes, such water would become hydrothermal fluids, capable of transporting any 238U decay products from nearby zircon grains and depositing polonium in biotite flakes to form polonium radiohalos. If this is correct, metasandstones should contain polonium radiohalos, and metasandstones could be studied to test the claim.
The Thunderhead Sandstone, Tennessee–North Carolina
The thick Thunderhead Sandstone forms a significant part of the 4,500–7,500 meter (15,000–25,000 feet) thick Great Smoky Group of the Ocoee Series in the Great Smoky Mountains along the Tennessee–North Carolina border in the southern part of the Blue Ridge province of the Appalachian Highlands (King 1964; Hadley and Goldsmith 1963; King et al. 1958). Its Upper Precambrian designation suggests it may have been deposited early in the Flood. The most common rock in the formation is relatively homogeneous medium- to coarse-grained feldspathic sandstone (arkose), with interbeds of argillaceous sandstone, and dark-gray argillite. The Ocoee Series was subsequently thrust northwest along the Greenbrier Fault early in the Paleozoic (fig. 1). The Thunderhead Sandstone comprises about half of the thrust sheet.
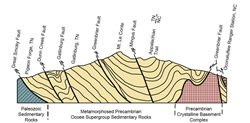
Click to enlarge.
Fig. 1. A geologic cross-section of the Great Smoky Mountains from Pigeon Forge, Tennesse, across Mt. Le Conte to the Oconaluftee Ranger Station near Cherokee, North Carolina (after Moore 1988).
After thrusting, the lithologic units of the entire Ocoee Series and the underlying Precambrian crystalline basement complex were regionally metamorphosed coincident with the orogenic (mountain-building) deformations during formation of the Appalachian Highlands, beginning in the Devonian (early-middle Flood). In the northwest the rocks were metamorphosed to the greenschist facies, but increasing temperatures and pressures to the southeast produced almandine amphibolite facies rocks. This regional metamorphism thus produced in the Thunderhead Sandstone a series of chemically and mineralogically distinct zones of schists and gneisses (fig. 2) (Allen and Ragland 1972). These zones are named according to the presence of the highest pressure/temperature metamorphic minerals in the rock. Shaly interbeds in the Thunderhead Formation are completely recrystallized throughout the sequence, so the demarcation of the isograds is based on the minerals in the shales. As the intensity of the metamorphism increases laterally from northwest to southeast—the shaly interbeds successively contain biotite, garnet, staurolite, and kyanite in the biotite, garnet, staurolite and kyanite zones, respectively. The boundaries between adjacent zones are called isograds.
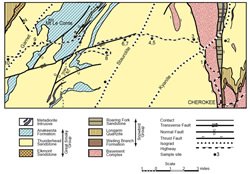
Click to enlarge.
Fig. 2. Geologic and metamorphic map of the central Great Smoky Mountains, showing the Thunderhead Sandstone,the isograds between regional metamorphic zones, and the location of the meta-arkose samples collected in this study (after Allen and Ragland 1972).
The arkosic sandstone in the Thunderhead Formation is slightly different. Figure 3 depicts the mineralogic variations within the metamorphosed arkoses of the Thunderhead Sandstone along a traverse from northwest (biotite zone) to southeast (kyanite zone). With the exception of garnet in the garnet and staurolite zones, the same mineral assemblage of quartz, K-feldspar (microcline), plagioclase, biotite, and muscovite is found throughout the sequence of meta-arkoses. Relict quartz and K-feldspar in the metamorphosed arkoses of the Thunderhead Sandstone exhibit little recrystallization through the biotite and garnet zones until the staurolite zone (Allen and Ragland 1972). In the garnet zone the presence of metamorphic garnet in the meta-arkose, which should not be stable according to the whole rock chemistry, indicates that equilibrium was obtained only in the interstitial portions of the rock and mineral system.
Regional Metamorphism—Hydrothermal Fluids and Po Radiohalos
Currently, in every regional metamorphic zone the meta-arkoses of the Thunderhead Formation contain both zircons and biotite flakes. In the original sedimentation process biotite flakes were probably destroyed, leaving the original Thunderhead sandstone without any biotite. However, Allen and Ragland (1972) specifically noted that the accessory zircon grains they observed in the Thunderhead were of detrital origin. Thus the original Thunderhead sandstones probably contained zircons but no biotite. Because the zircon grains would likely have still contained minor amounts of uranium, they could thus have been a source of 238U decay products, including polonium. During regional metamorphism biotite and hydrothermal fluids were generated—some from pre-metamorphism pore waters and some from metamorphic reactions. Therefore, according to the hydrothermal fluid transport model for polonium radiohalo formation, those hydrothermal fluids should have transported the polonium diffusing out of zircon grains into adjacent biotite flakes, where it should have concentrated in radiocenters and generated polonium radiohalos.
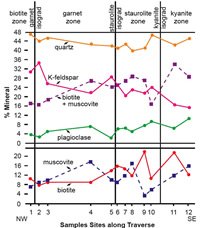
Click to enlarge.
Fig. 3. Mineralogic variations within the Thunderhead Sandstone (after Allen and Ragland 1972). The sample site numbers are those of Allen and Ragland (1972), as are the mineral percentages they determined.
Allen and Ragland (1972) found from analyses of the bulk rock geochemistry that metal/Al ratios in the Thunderhead meta-arkoses remain constant throughout the biotite and garnet zones. The preservation of relict grains in the biotite and garnet zones was attributed to lack of complete recrystallization during the regional metamorphism due to a lack of a significant aqueous phase. The high porosities and permeabilities inherent in the original sandstones would have resulted in most of their pore waters being driven off by the heat and load pressure during the initial stages of metamorphism. Structural water and some absorbed pore water in the clays of the sandstone matrix probably provided the water necessary for crystallization of the micas in the biotite and garnet zones. Thus most of the water was held in crystal structures within the meta-arkoses and was not readily available to act as a transporting medium. According to the hydrothermal fluid transport model for polonium radiohalo formation, relatively little radiohalo generation would be expected—at least in the meta-arkoses of the Thunderhead Formation.
Near the staurolite isograd, the boundary between the garnet and staurolite zones, however, numerous chemical changes are apparent in the bulk rocks and in the micas (Allen and Ragland 1972). These changes and the disappearance of relict minerals suggest a significant aqueous phase was generated at the staurolite isograd. Mineral phases such as staurolite were formed that contain notably less structural water, while chlorite disappears entirely in the pelitic beds within the meta-arkoses, muscovite decreases sharply, and biotite becomes more abundant. This suggests the following reaction predominated at the staurolite isograd:
54 muscovite + 31 chlorite
→54 biotite + 24 staurolite + 152 quartz + 224 water
This reaction has been confirmed experimentally (Hoschek 1967, 1969). In the pelitic beds it would have released some 63 percent of the structural water from the reacting minerals. The generation of large quantities of water by this reaction within the pelitic interbeds at the prevailing high temperatures determined experimentally would have resulted in relatively large volumes of hydrothermal fluids migrating down the PH20 free-energy gradient into the adjacent meta-arkoses. Within the meta-arkoses these hydrothermal fluids would have provided an aqueous transporting medium that resulted in a rapid catalyzing action. This would have caused the recrystallization of all relict grains and a rapid approach to equilibrium. At the entry of this aqueous phase, chemical equilibrium was set up over the macrosystem of large volumes of meta-arkose, rather than just in the interstitial microsystems encountered in the lower metamorphic grade zones.
These conditions in the Thunderhead meta-arkoses at the staurolite isograd should have been ideal for the generation of Po radiohalos in them, assuming that Po radiohalo formation does indeed occur as described by the hydrothermal fluid transport model (Snelling and Armitage 2003; Snelling, Baumgardner, and Vardiman 2003; Snelling 2005). Detrital zircon grains to provide 238U decay products, and metamorphic biotite flakes to host Po concentrations in radiocenters, were present in the meta-arkoses, and around the staurolite isograd the copious quantities of hydrothermal fluids flowed through the meta-arkoses as a result of the mineral reaction in the pelitic interbeds. Therefore, it was predicted that many more Po radiohalos should be found in the Thunderhead Sandstone in the meta-arkoses surrounding the staurolite isograd than elsewhere in the meta-arkoses throughout these regional metamorphic zones.
Field and Laboratory Work
A field test of this prediction was thus proposed. Nine samples of the meta-arkoses of the Thunderhead Sandstone were collected from road-cut outcrops along U.S. Highway 441 between Cherokee, North Carolina, and Gatlinburg, Tennessee (figs. 2 and 4). This effectively formed a traverse through the kyanite, staurolite, garnet, and biotite zones of the regional metamorphism as already described (Allen and Ragland 1972). A basement gneiss sample was also collected.
Click to enlarge.
Fig. 4. Outcrops of the meta-arkoses of the Thunderhead
Sandstone, where the samples for this study were
collected, mainly along U.S. Highway 441 between
Cherokee NC and Gatlinburg TN. Their locations are
plotted on Fig. 2.
(a) Sample site RSM-2, kyanite zone
(b) Sample site RSM-3, staurolite zone
(c) Sample site RSM-4 & 5, staurolite zone (note the
shaly interbeds to the bottom right)
(d) Sample site RSM-6 & 7, garnet zone
(e) Sample site RSM-8, garnet zone
(f) Sample site RSM-10, biotite zone
A standard petrographic thin section was obtained for each meta-arkose sample. In the laboratory, portions of each sample were crushed to liberate the constituent mineral grains. For each sample, biotite flakes were then hand-picked using tweezers and placed on the adhesive surface of a piece of clear Scotch tape. Once numerous biotite flakes had been mounted on the adhesive surface of this tape, a fresh piece of clear Scotch tape was placed over them and firmly pressed along its length so as to ensure the two pieces of tape were stuck together with the biotite flakes firmly wedged between them. The upper piece of clear Scotch tape was then peeled back in order to pull apart the biotite flakes. This upper piece of clear Scotch tape with thin biotite sheets adhering to it was then placed over a standard glass microscope slide. This procedure was repeated with another piece of clear Scotch tape placed over the original Scotch tape with the biotite flakes adhering to it. These adhering biotite flakes were progressively pulled apart and transferred to microscope slides. As necessary, further hand-picked biotite flakes were added to replace those fully pulled apart. In this way 50 microscope slides were prepared for each meta-arkose sample, each slide with many (at least 20–30) thin biotite flakes mounted on it. This is similar to the method pioneered by Gentry (Gentry, pers. comm.). The basement gneiss sample was treated in the same way. Fifty microscope slides for each sample were prepared to ensure good representative sampling statistics. Thus there was a minimum of 1,000 biotite flakes mounted on microscope slides for each sample.
Click to enlarge.
Fig. 5. Representative photo-micrographs of the meta-arkose samples of the Thunderhead Sandstone
used in this study collected from outcrops of each regional metamorphic zone (fig. 4), as plotted on Fig. 2. All photomicrographs are at the same scale (20× or 1 mm = 40 μ) and the meta-arkoses are as viewed under crossed polars.
(a) Sample RSM-2 (kyanite zone): quartz, biotite, muscovite, zircon
(b) Sample RSM-3 (staurolite zone): quartz, plagioclase, biotite, muscovite, garnet
(c) Sample RSM-4 (staurolite zone): quartz, biotite, garnet, muscovite, staurolite
(d) Sample RSM-5 (staurolite zone): quartz, muscovite, biotite
(e) Sample RSM-6 (garnet zone): quartz, plagioclase, muscovite, biotite, zircon
(f) Sample RSM-7 (garnet zone): quartz, biotite, garnet, muscovite
(g) Sample RSM-8 (garnet zone): quartz, muscovite, biotite
(h) Sample RSM-10 (biotite zone): quartz, plagioclase, muscovite, biotite
Each slide for each sample was then carefully examined under a petrological microscope in plane polarized light, and all radiohalos present were identified, noting any relationships or unusual features. The numbers of each type of radiohalo in each slide were counted by progressively moving the slide backward and forward across the field of view, and the numbers recorded for each slide were then tallied and tabulated for each sample. Because of the progressive peeling apart of many of the same biotite flakes during the preparation of the microscope slides, it was possible that some of the radiohalos appeared on more than one microscope slide. Only radiohalos whose radiocenters were clearly visible were thus counted to ensure each radiohalo was only counted once.
Results
Representative photo-micrographs of meta-arkose samples from each regional metamorphic zone are shown in Fig. 5. All results are listed in Table 1. Nine of the samples (eight meta-arkoses and one basement gneiss) contained only 210Po radiohalos, while one meta-arkose sample also contained 214Po radiohalos. Some representative examples of the radiohalos in the samples can be seen in Fig. 6. Table 1 lists both the total number of 210Po and 214Po radiohalos found in each sample, and the average numbers of Po radiohalos per slide in each sample. The total number of Po radiohalos found in each sample was then plotted against each sample’s relative position along the traverse in Fig. 2 through the metamorphic zones, which is displayed in Fig. 7.
As can be readily seen in Table 1 and Fig. 7, whereas seven of the meta-arkose samples averaged a total of around 30 Po radiohalos each, the two samples straddling the staurolite isograd contained 177 and 147 Po radiohalos, respectively.
Discussion
The results of this test of the hydrothermal fluid transport model for Po radiohalo formation were astounding, being exactly as predicted. The Po radiohalos were four–five times more abundant in the two meta-arkose samples straddling the staurolite isograd than in the immediately adjoining meta-arkose samples in the garnet and staurolite zones (table 1 and fig. 7). The Po radiohalo numbers were even less in the other meta-arkose samples, including the one sample from the high-grade kyanite zone.
Table 1. Data table of radiohalos numbers counted in samples of the meta-arkoses of the Thunderhead Sandstone and the basement gneiss. | ||||||||
Radiohalos | ||||||||
---|---|---|---|---|---|---|---|---|
Sample | Number of Slides | 210Po | 214Po | 218Po | 238U | 232Th | Number of Po Radiohalos per Slide | Metamorphic Zone |
RSM-1 | 50 | 4 | 0 | 0 | 0 | 0 | 0.08 | Basement Gneiss |
RSM-2 | 50 | 34 | 0 | 0 | 0 | 0 | 0.68 | Kyanite |
RSM-3 | 50 | 24 | 0 | 0 | 0 | 0 | 0.48 | Staurolite |
RSM-4 | 50 | 26 | 19 | 0 | 0 | 0 | 0.90 | Staurolite |
RSM-5 | 50 | 147 | 0 | 0 | 0 | 0 | 2.94 | Staurolite |
RSM-6 | 50 | 177 | 0 | 0 | 0 | 0 | 3.54 | Garnet |
RSM-7 | 50 | 41 | 0 | 0 | 0 | 0 | 0.82 | Garnet |
RSM-8 | 50 | 35 | 0 | 0 | 0 | 0 | 0.70 | Garnet |
RSM-9 | 50 | 6 | 0 | 0 | 0 | 0 | 0.12 | Garnet |
RSM-10 | 50 | 28 | 0 | 0 | 0 | 0 | 0.56 | Biotite |
The numbers of Po radiohalos do not correlate with metamorphic grade. There were only 34 Po radiohalos in the kyanite zone (high grade) meta-arkose sample compared with only 28 Po radiohalos in the biotite zone (low grade) meta-arkose sample. Yet there were U-bearing zircon grains and biotite flakes present in all samples of the Thunderhead Sandstone along the traverse from low grade through to high grade regional metamorphism.
Neither the Po which generated the Po radiohalos nor the biotite flakes which host the Po radiohalos were primordial. Unlike the zircon grains, the biotite flakes were not present in the arkoses when they were deposited but were produced by mineral transformations and reactions during the subsequent regional metamorphism. This means that the Po which generated the Po radiohalos in the biotite flakes had to be transported from in situ nearby sources into the biotite flakes after they had formed. As has been argued by Snelling and Armitage (2003) and Snelling (2005), the only in situ nearby sources of Po are the decaying 238U atoms in the zircon grains in the originally deposited arkoses. Thus it is argued that the required Po had to be transported from the zircon grains into the biotite flakes after they had formed, and hydrothermal fluids (hot waters) seem to be the logical candidate (Snelling and Armitage 2003; Snelling 2005).
Click to enlarge.
Fig. 6. Some representative examples of the 210Po radiohalos found in biotite grains separated from the
meta-arkoses of the staurolite and garnet zones in the Thunderhead Sandstone in this study. All photo-micrographs
are at the same scale (40× or 1 mm = 20 μm) and the biotite grains are as viewed in plane polarized light.
(a) & (b) RSM-4 (c), (d) & (e) RSM-5 (f), (g) & (h) RSM-6
When arkoses (and sandstones) are deposited by and from moving water, some of that water is often trapped between the mineral grains making up the sandy sediments. The cement that binds the mineral grains together to transform the sand into sandstone and arkose usually is precipitated from that trapped water, but also from meteoric water that percolates down into the sediments. Once lithified, sandstone and arkoses still often contain much ground water. When such water-saturated sedimentary rocks subsequently become deeply buried and are subjected to tectonic forces, the heat generated thereby turns the contained waters into hydrothermal fluids as the sedimentary rocks undergo regional metamorphism. Stanton (1982, 1989) and Snelling (1994) have argued that such hydrothermal fluids are primary catalysts in helping to transform sedimentary rocks into regional metamorphic complexes.
It was these hydrothermal fluids generated during regional metamorphism that were available to transport Po from 238U decay in detrital zircon grains into metamorphic biotite flakes to form Po radiohalos (Snelling and Armitage 2003; Snelling 2005). It was thus predicted, based on the hydrothermal fluid transport model for Po radiohalo formation, that Po radiohalos would be found in regionally metamorphosed sedimentary rocks. Consequently, because a search for Po radiohalos in metamorphic rocks was undertaken, Po radiohalos have been found in metamorphic rocks (Snelling 2005, 2006, 2008b).
The results of this study also confirm that the hydrothermal fluid transport model for Po radiohalo formation applies to regional metamorphic rocks. The 6–41 Po radiohalos per sample in each of the meta-arkose examples, except for the two straddling the staurolite isograd (table 1 and fig. 7), would appear to have been generated from Po transported by the hydrothermal fluids produced from the water originally in the sandstones during their regional metamorphism. However, where during the regional metamorphism the mineral reaction in the pelitic interbeds at the staurolite isograd produced a lot of extra hot water, the extra flow of hydrothermal fluids in the adjacent meta-arkoses generated four–five times more Po radiohalos, 144 and 177 in two relevant meta-arkose samples (table 1 and fig. 7).
This successful verification of the hydrothermal fluid transport model for Po radiohalo formation adds to the supporting evidence found by Snelling (2006). Where metamorphism along shear zones had been rapid, even in conventional terms, due to tectonic “pumping” of hydrothermal fluids, Po radiohalos had been generated in biotite flakes in the resultant rocks. In these examples, mainly 210Po radiohalos were generated in the metamorphic biotite flakes. This has timescale implications for these metamorphic processes and the hydrothermal fluid flows. 218Po and 214Po have half-lives of 3.1 minutes and 164 microseconds respectively, whereas 210Po’s half-life is 138 days. Thus, even if 218Po from 238U decay diffused out of the detrital zircon grains, by the time the hydrothermal fluids had transported the Po into the biotite flakes, where it was concentrated in radiocenters by Po-bonding atoms such as Cl or S at lattice defects, the 218Po had decayed through 214Po to 210Po, so only 210Po radiohalos would be generated.
This implies that the Po transport, from within the zircon grains the >1 mm (approximately 2–10 mm) distances across into the biotite flakes and along the cleavage planes in them to the Po radiocenters, probably took as long as a few days to even weeks. Note that in contrast, many granites contain 218Po and/or 214Po radiohalos, as well as 210Po radiohalos, due to the much shorter transport distances (<1 mm) because the source zircon grains are within the biotite flakes where the Po radiohalos are generated.
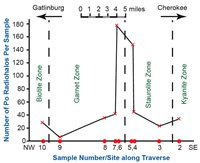
Click to enlarge.
Fig. 7. Po radiohalos for the meta-arkose samples along the traverse in this study (fig. 2) through the regional metamorphic zones across the Great Smoky Mountains, Tennessee–North Carolina.
On the other hand, the Po radiohalos in both these metamorphic rocks and granites would only have formed below 150°C, the annealing temperature of radiohalos in biotite. As in crystallizing granites, at higher temperatures during regional metamorphism, Po transport would have commenced as soon as the hydrothermal fluids were produced. Even at temperatures well above 400°C, the temperatures at which the mineral reaction occurred at the staurolite isograd, the hydrothermal fluids would have flowed vigorously (but under pressure) transporting available Po. However, because the Po radiohalos could not be generated and visually registered in the biotite flakes until the temperature in the metamorphosed rocks fell below 150°C, the cooling of the whole regional metamorphic complex needs to have been relatively rapid, within days to a few weeks, to ensure there was still sufficient 210Po to produce the 210Po radiohalos before all the 210Po decayed.
It may be countered that because 238U today decays slowly, there would have been a continuous supply of Po over millions of years. So the hydrothermal fluid transport of Po and the generation of the Po radiohalos could have taken millions of years of incremental additions of Po to the radiocenters. However, the concurrent formation of 238U and Po radiohalos in the same biotite flakes in many granites (Snelling and Armitage 2003; Snelling 2005, 2008a), and other evidence (Vardiman, Snelling, and Chaffin 2005), is consistent with 238U decay having been grossly accelerated during an event, or events, in earth history, particularly the Genesis Flood. This is when the deposition of the Thunderhead Sandstone is envisaged, followed by the regional metamorphism. So an abundant supply of Po during this accelerated 238U decay in the detrital zircons was only very short-lived. Thus, unless the Po was transported rapidly from the detrital zircons to the radiocenters in the metamorphic biotite flakes, the Po would have decayed before the Po radiohalos could have formed.
Furthermore, both the hydrothermal fluid flows and the regional metamorphism had to also have been rapid and short-lived. Unless the hydrothermal fluid flows below 150°C were rapid, the transported Po would have decayed before reaching the radiocenters. However, the hydrothermal fluid flows are driven by the heat energy associated with the regional metamorphism. As with cooling granite bodies (Snelling 2008a), much of the heat would have been dissipated by the time the temperatures in the regionally metamorphosed rock had fallen below 150°C, so the hydrothermal fluid flows would have begun to diminish. Thus, the regional metamorphism had to be a rapid event to drive the rapid hydrothermal fluid flows.
In the context of the year-long Genesis Flood event, catastrophic plate tectonics would have driven the rapid, catastrophic earth movements that produced the tectonic settings, where rapid regional metamorphism of thick sedimentary rock sequences would have taken place (Austin et al. 1994). Coupled with catastrophic plate tectonics, concurrent catastrophic accelerated radioisotope decay (Vardiman, Snelling, and Chaffin 2005) would have provided a rapid burst of heat in those developing regional metamorphic complexes to generate the rapid hydrothermal fluid flows and the metamorphic mineral reactions. Thus the presence of the Po radiohalos in the regionally metamorphosed Thunderhead Sandstone are testimony to the catastrophic tectonic and geologic processes that formed the Great Smoky Mountains as part of the Appalachian mountain-building episode early in the Genesis Flood, and validate the hydrothermal fluid transport model for their formation.
Conclusions
Based on the mineralogical changes produced by regional metamorphism in the arkoses of the Thunderhead Sandstone, Great Smoky Mountains, Tennessee–Carolina, a test of the hydrothermal fluid transport model for Po radiohalo formation was proposed. These meta-arkoses contain both detrital zircon grains (a potential source of Po from 238U decay) and metamorphic biotite flakes (a potential host for Po radiohalos). When originally deposited the arkoses would have retained pore and meteoric waters, which during regional metamorphism would have become hydrothermal fluids. Furthermore, the reaction responsible for the mineralogical changes at the staurolite isograd (the boundary between the garnet and staurolite metamorphic zones) would have produced large volumes of water as hydrothermal fluids. It was thus predicted that if the hydrothermal fluid transport model for Po radiohalo formation is valid, then there should be Po radiohalos in the biotite flakes of these meta-arkoses, and there should be greater numbers of Po radiohalos at the staurolite isograd.
Both predictions were verified. All nine samples of the meta-arkoses collected along a traverse through the regional metamorphic zones contained Po (mainly 210Po) radiohalos. Furthermore, the two samples straddling the staurolite isograd contained four–five times more Po radiohalos than the other seven samples. These results also verify the hydrothermal fluid transport model for Po radiohalo formation. The presence of mainly 210Po radiohalos was also consistent with the longer transport distance (> 1mm) from the zircon grains to the biotite flakes. It was concluded that, because these Po radiohalos could only have formed below 150°C (the annealing temperature of radiohalos in biotite), both the regional metamorphism and the hydrothermal fluid flows to transport the required Po had to be rapid. These processes, plus the cooling of the regional metamorphic complex and the formation of the Po radiohalos, had to have all occurred within a few weeks. This is all feasible in the context of catastrophic plate tectonics and grossly accelerated 238U decay during the Genesis Flood.
Acknowledgments
This research was encouraged by Kurt Wise, who also provided the logistical support for the field work. Mark Armitage is acknowledged for his work in processing the samples and counting the radiohalos. Funding was provided by the Institute for Creation Research from donations received toward the RATE project.
References
Allen, G. C., and P. C. Ragland. 1972. Chemical and mineralogical variations during prograde metamorphism, Great Smoky Mountains, North Carolina and Tennessee. Geological Society of America Bulletin 83:1285–1298.
Austin, S. A., J. R. Baumgardner, D. R. Humphreys, A. A. Snelling, L. Vardiman, and K. P. Wise. 1994. Catastrophic plate tectonics: A global Flood model of earth history. In Proceedings of the Third International Conference on Creationism, ed. R. E. Walsh, pp. 609–621. Pittsburgh, Pennsylvania: Creation Science Fellowship.
Hadley, J. B., and R. Goldsmith. 1963. Geology of the eastern Great Smoky Mountains, North Carolina—Tennessee. Professional Paper 349-B. Washington, D.C.: U.S. Geological Survey.
Hoschek, G. 1967. Untersuchungen zum stabilitätsbereich von chloritoid und staurolith. Contributions to Mineralogy and Petrology 14:123–162.
Hoschek, G. 1967. The stability of staurolite and chloritoid and their significance in metamorphism of pelitic rocks. Contributions to Mineralogy and Petrology 22:208–232.
King, P. D. 1964. Geology of the central Great Smoky Mountains, Tennessee. Professional Paper 349-C. Washington, D.C.: U.S. Geological Survey.
King, P. B., J. B. Hadley, R. B. Neuman, and W. B. Hamilton. 1958. Stratigraphy of the Ocoee Series, Great Smoky Mountains, Tennessee and North Carolina. Geological Society of America Bulletin 69:947–956.
Moore, H. L. 1988. A roadside guide to the geology of the Great Smoky Mountains National Park. Knoxville, Tennessee: The University of Tennessee Press.
Snelling, A. A. 1994. Towards a creationist explanation of regional metamorphism. Creation Ex Nihilo Technical Journal 8:51–77.
Snelling, A. A. 2000. Radiohalos. In Radioisotopes and the age of the earth: A young-earth creationist initiative, ed. L. Vardiman, A. A. Snelling, and E. F. Chaffin, pp. 381–468. El Cajon, California: Institute for Creation Research; St. Joseph, Missouri: Creation Research Society.
Snelling, A. A. 2005. Radiohalos in granites: Evidence for accelerated nuclear decay. In Radioisotopes and the age of the earth: Results of a young-earth creationist research initiative, ed. L. Vardiman, A. A. Snelling, and E. F. Chaffin, pp. 101–207. El Cajon, California: Institute for Creation Research; Chino Valley, Arizona: Creation Research Society.
Snelling, A. A. 2006. Confirmation of rapid metamorphism of rocks. Impact #392. El Cajon, California: Institute for Creation Research.
Snelling, A. A. 2008a. Catastrophic granite formation: Rapid melting of source rocks and rapid magma intrusion and cooling. Answers Research Journal 1:11–25.
Snelling, A. A. 2008b. Radiohalos in the Cooma Metamorphic Complex, NSW, Australia: The mode and rate of regional metamorphism. In Proceedings of the Sixth International Conference on Creationism, ed. A. A. Snelling, in press. Pittsburgh, Pennsylvania: Creation Science Fellowship; Dallas, Texas: Institute for Creation Research.
Snelling, A. A., and M. H. Armitage. 2003. Radiohalos—A tale of three granitic plutons. In Proceedings of the Fifth International Conference on Creationism ed. R. L. Ivey, Jr., pp. 243–267. Pittsburgh, Pennsylvania: Creation Science Fellowship.
Snelling, A. A., J. R. Baumgardner, and L. Vardiman. 2003. Abundant Po radiohalos in Phanerozoic granites and timescale implications for their formation. EOS, Transactions of the American Geophysical Union 84:46. Fall Meeting Supplement: Abstract V32C-1046.
Spear, F. S. 1993. Metamorphic phase equilibria and pressure-temperature-time paths. Washington, D.C.: Mineralogical Society of America.
Stanton, R. L. 1982. An alternative to the Barrovian interpretation? Evidence from stratiform ores. Proceedings of the Australasian Institute of Mining and Metallurgy 282:11–32.
Stanton, R. L. 1989. The precursor principle and the possible significance of stratiform ores and related chemical sediments in the elucidation of processes of regional metamorphic mineral formation. Philosophical Transactions of the Royal Society of London A328:529–646.
Vardiman, L., A. A. Snelling, and E. F. Chaffin, eds. 2005. Radioisotopes and the age of the earth: Results of a young-earth creationist research initiative. El Cajon, California: Institute for Creation Research; Chino Valley, Arizona: Creation Research Society.